ABSTRACT
Background and objectives: DNA hypermethylation has been linked to poor treatment outcome in childhood acute lymphoblastic leukemia (ALL). Genes differentially methylated in the chemoresponsive pre-B-ALL compared to chemoresistant pre-B-ALL cases provide potential prognostic markers.
Methods: DNA methylation profiles of five B-ALL childhood patients who achieved morphological complete remission (chemoresponsive) and five B-ALL patients who did not (chemoresistant) after induction treatments as well as four normal controls were compared on 27 000 CpG sites microarray chips. Subsequently, methylation-specific polymerase chain reaction (MSP) on selected hypermethylated genes was conducted on an additional 37 chemoresponsive and 9 chemoresistant B-ALL samples and 2 normal controls.
Results: Both methods were found to be highly correlated. Unsupervised principal component analysis showed that the chemotherapy-responsive and -resistant B-ALL patients could be segregated from one another. Selection of segregated genes at high stringency identified two potential genes (CDH11 and ADAMTSL5). MSP analysis on the larger cohort of samples (42 chemoresponsive, 14 chemoresistant B-ALL samples and 6 normal controls) revealed significantly higher rates of hypermethylation in chemoresistant samples for ADAMTSL5 (93 vs. 38%; p = 0.0001) and CDH11 (79% vs. 40%, p < 0.01). All control cases remained unmethylated.
Conclusion: Chemoresistant B-ALL patients are associated with increased methylation in ADAMTSL5 and CDH11. These findings need to be validated in a larger group of patients, and the functional biological and prognostic significance of differential methylation needs to be studied further.
Introduction
Acute lymphoblastic leukemia (ALL) consists of a heterogeneous group of lymphoid malignancies [Citation1]. ALL is curable in 80% of children; however, a substantial number remains resistant to treatment [Citation2]. DNA methylation is a common mechanism where the transfer of a methyl group to cytosine in CG-rich regions, called CpG islands (CGI), is usually associated with gene silencing. The process is mediated by DNA methyltransferase and is reversible. Aberrant hypermethylation or hypomethylation may lead to abnormal gene expression and disease formation. In cancers, inactivation of tumor suppressor genes has been due to promoter CGI hypermethylation. Involvement of other sites in signaling pathways and cellular processes is beginning to be identified [Citation3].
DNA hypermethylation has also been linked with sensitivity of tumor cells toward chemotherapy treatment. Epigenetic inactivation of O6-methylguanine-DNA methyltransferase [Citation4], checkpoint with FHA and RING finger [Citation5], Werner syndrome gene (WRN) [Citation6], and p73 [Citation7] has shown a better response to 1,3-bis(2-chloroethyl)-1-nitrosourea, microtubule inhibitors, topoisomerase inhibitor irinotecan, and alkylating agents, respectively, in different cancer cell types. Interestingly, Shen et al. [Citation7] found that concurrent methylation of THBS1 (thrombospondin-1), p15INK4b (cell cycle inhibitory protein), and COX2 (cyclooxygenase2) showed a significant correlation with resistance to pentamethylmelamine.
Identifying epigenetic abnormality has added advantages, as current epigenetic therapies are available with the potential of reversing epigenetic state. In 2004 and 2006, the US FDA approved two demethylating agents, 5-azacytidine and decitabine, for the treatment of patients with myelodysplastic syndromes [Citation3]. Similar applications are encouraging for acute lymphoblastic leukemias [Citation8,Citation9]. Various recent genome-wide studies on DNA methylation in ALL looking at pathogenesis [Citation10–12] and risk of relapse [Citation13–15] have identified significant patterns of importance. Current data on drug response and methylation pattern in ALL are limited. Thus, genome-wide analysis on B-ALL with different treatment outcomes was conducted to identify genes which are differentially methylated in the chemoresponsive (or sensitive) pre-B-ALL as compared to that of chemoresistant pre-B-ALL cases which may be used as predictive markers in these cases.
Materials and methods
Patients
B-ALL patients of both genders, aged 0–18 years old from UKM Medical Centre and Institute of Paediatrics, Kuala Lumpur, were recruited for this study. This study was approved by both Institutional Review Boards and Ethic Committees. Six peripheral blood samples from normal individuals were also included as control. All procedures complied with good clinical practice outlined in the Declaration of Helsinki (1964). Diagnosis of ALL was based on morphology, negative myeloperoxidase cytochemistry, and immunophenotyping, showing positivity to B-lineage-associated lymphoid differentiation antigens. The treatment of pre-ALL was according to the UKALL 2003 protocol.
Bone marrow remission was defined as the presence of fewer than 5% blasts with hemopoietic recovery. Patients who did not achieve complete remission (CR) after one course of chemotherapy at first induction (CR1) chemotherapy or induction following relapse (CR2) were defined as chemoresistant. Mononuclear cells were isolated using Ficoll-Paque according to the standard methods. Samples were cryopreserved in 10% DMSO, 20% FBS in RPMI 1640. An initial 10 B-ALL samples (five resistant to induction treatment at CR1 and five in CR) and 4 normal controls were used for methylation array study (Supplementary Table S1).
DNA extraction and bisulfite modification
Genomic DNA was isolated from cryopreserved bone marrow samples of B-ALL cases and normal controls using the QIAamp DNA Blood Mini Isolation Kit (Qiagen, Chatsworth, CA, U.S.A.). The extracted DNA was treated with sodium bisulfite using the Zymo Research EZ DNA Methylation Kit (Zymo Research Cooperation, Orange, CA, U.S.A.).
Genome-wide DNA methylation profiling
Genome-wide DNA methylation profiling was performed on five chemoresistant pre-B-ALL, five CR (chemoresponsive) ALL, and four normal controls. Sodium bisulfite-treated DNA was hybridized to Infinium HumanMethylation27 BeadChip according to the manufacturer’s protocol. Genome-wide DNA methylation profiling of samples was done using HumanMethylation27 Beadchip (Illumina, San Diego, CA, U.S.A.), which contained more than 14 000 well-annotated human genes and 27 000 highly informative CpG sites.
Methylation microarray analysis
Beadstudio Methylation Module v3.2. (Illumina) was used for background correction. A preliminary methylation analysis was conducted at a false discovery rate (FDR) below 0.01%. Filtering was applied at a beta-value of more than or equal to 0.5 for chemoresistant group, while the beta-values for the CR group and normal controls were less than or equal to 0.3. CpGs that reside in the X-chromosome were excluded to avoid bias caused by methylation of inactive X-chromosome. Based on these, 11 genes were identified (Supplementary Figure S1). Four genes (SFRS7, UNC5C, DMN, and ZNF667) with at least 60% methylation in the resistant group were randomly selected and further tested to confirm microarray intensity levels by methylation-specific polymerase chain reaction (MSP). PCR validation of microarray experiment was done on the same five chemoresistant ALL, five CR (chemoresponsive/sensitive) pre-B-ALL but only two normal controls, as those used in the genome-wide DNA methylation assay.
PARTEK Genome Suite (Partek, Inc., St Louis, MO, U.S.A.) software was used for global comparisons between samples. Exploratory data analysis was performed using unsupervised principal component analysis (PCA). Two-dimensional unsupervised clustering clearly grouped the patients according to their resistance to chemotherapy. Using a stringent significance level (p < 0.0001) and FDR (0.001%), 37 significantly differentially methylated genes were identified in chemotherapy-resistant group. CDH11 and ADAMTSL5 were hypermethylated in four and five chemotherapy-resistant patients (n = 5), respectively. None of these two genes were found to be hypermethylated in chemotherapy-sensitive patients (n = 5) and in the normal control group (n = 4). Another gene, ZNF214, was hypermethylated in all 10 B-ALL samples but not in normal controls. By setting a higher stringent discovery criterion with a significant level (p < 0.0001) and FDR (0.0001%) at 10 times lower than before, 12 genes were identified ((B)) including CDH11 and ADAMTSL5 but not ZNF214. These genes were chosen because of their relatively higher beta-values in chemoresistant samples ((C), shown for CDH11 and ADAMTSL5). All three were included for further analysis on an additional 46 B-ALL (37 CR and 9 Res) and 2 normal controls using methylation-specific primer PCR to confirm the suitability of these genes as potential prognostic markers. Thus, samples used in this validation study were normal (n = 6), pre-B-ALL-resistant (n = 14), and pre-B-responsive (n = 42). The product and annotation for these genes, as provided in the manufacturer’s gene list, are shown in Supplementary Table S2.
Figure 1. (A) Using PCA with the Partek Genomics Suite software showed good segregation of normal (N1–N4), chemotherapy-responsive/-sensitive (NR1–NR5), and chemotherapy-resistant (R1–R5) samples. (B) Hierarchical clustering identified 12 significantly differently methylated genes in the chemoresistant group. Majority were methylated higher in this group. Acute lymphoblastic leukemia samples (NR and R) were tested in duplicates. (C) Dot plots of the most differentially methylated genes, CDH11 and ADAMTSL5, showing beta-values for individual samples (N1–N4, NR1–NR5, and R1–R5) and also median beta-values for the three groups, chemoresponsive (sensitive), chemoresistant (resistant) acute lymphoblastic leukemia, and normal samples.
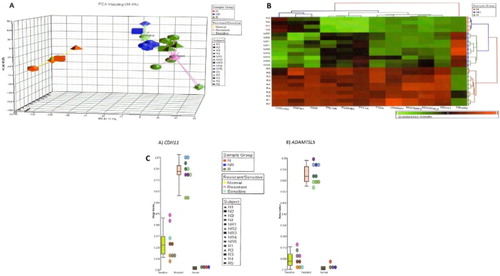
Methylation-specific PCR analysis
MSP analysis was performed on 56 ALL cases and 6 normal controls (Supplementary Table S1). All the cases analyzed in the methylation microarray were included in the MSP analysis. Primers specifically targeting the methylated and unmethylated alleles were designed using the PerlPrimer software [Citation16], which is freely downloadable from http://perlprimer.sourceforge.net/. After identifying the SourceSeq of the gene of interest from the Illumina_Human Methylation27_Content which provided the gene list, a forward primer was designed with a CpG site located in the very 3′-end of the sequence. The unmethylated primer set uses thymine (T) in place of C at CpG locations. Primer sequences are shown in Supplementary Table S3 for both genes used to confirm microarray results and to confirm potential prognostic genes. Each of the MSP reactions contained sodium bisulfite-treated DNA (∼30 ng), 0.4 µM each primer, and 1× of Hotstar Taq master mix (Qiagen) in a final volume of 20 µl. MSP was carried out at 95°C for 5 min, 40 cycles of 95°C for 30 s, specific annealing for each gene for 30 s, and 72°C for 30 s, and a final extension at 72°C for 5 min in a thermocycler (ABI Applied Biosystem). For each PCR, a universally methylated DNA (Universal Methylated Human DNA Standard™, Zymo Research Cooperation) and water were used as positive and negative controls, respectively. PCR products were subjected to agarose gel electrophoresis ethidium bromide staining and viewed under a gel imager (Kodak Imaging System).
Results
A total of 56 B-ALL samples were collected, 42 achieved CR, and 14 were chemoresistant (Res). Clinical characteristics of pre-B-ALL with known treatment outcome are presented in . Six normal controls were also included.
Table 1. Clinical characteristics of pre-B-ALL achieving CR and Res.
Methylation analysis, using the BeadStudio Methylation Module v3.2 software, identified 11 genes, including ZNF667, HHN2, CARS, WRN, DMN, LNC5C, SYNC1, SFRS7, PAX6, SCUBE1, and ST6GALNAC3, showing higher frequency of hypermethylation in the chemoresistant group (Supplementary Figure S1).
MSP analysis, of four randomly selected genes (SFRS7, UNC5C, DMN, and ZNF667) from the 11 genes, showed good correlation in the methylation status of the original five chemoresistant (Res1–Res5), five with CR (CR1–CR5) pre-B-ALL and two normal controls (N1–N2) with results from the microarray experiment (Supplementary Figures S2A and S2B).
Preliminary data exploration with the Partek Genomics Suite software by unsupervised PCA showed clear separation between normal, chemotherapy-sensitive/-responsive and -resistant ALL patients ((A)). At higher FDR (0.0001%), 12 significantly differentially methylated genes (C20orf55, ROPN1, NGB, PRLHR, PCDHB3, PTF1A, TTPA, JAKMIP1, MAPK8IP2, ADAMTSL5, CDH11, and C8orf70) were identified in the chemotherapy-resistant group as visualized with hierarchical clustering ((B)). Specific gene comparison for methylation between the groups (normal control, chemoresponsive, and chemoresistant) for the selected genes showed that CDH11 and ADAMTSL5 were the most differently methylated ((C)).
MSP analysis of methylated and unmethylated ADAMTSL5, CDH11, and ZNF214 was performed on 56 B-ALL and 6 normal controls. Representative gel images are shown in . Distribution of DNA hypermethylation in normal controls, chemoresistant, and chemosensitive pre-B-ALL is shown in . Statistical analysis to compare the methylated status of ADAMTSL5, CDH11, and ZNF214 in chemoresistant, CR B-ALL, and normal controls showed significant difference between all groups. Methylation of ADAMTSL5 and CDH11 was significantly higher in the chemoresistant group, with ADAMTSL5 being most significant (). In contrast, ZNF214 showed the least significant difference between chemoresistant and CR B-ALL, but was highly differentiated between B-ALL and normal controls.
Figure 2. Representative gel electrophoresis of amplicons from PCR using methylated (M) and unmethylated (U) primers specific to (A) ADAMTSL5, (B) CDH11, and (C) ZNF214 on normal controls (n = 6), chemoresistant (n = 5), and chemoresponsive/-sensitive (n = 5) pre-B-ALL. These are the samples used in the microarray experiment. Each sample amplified by methylated and unmethylated primers is separated by dotted lines.
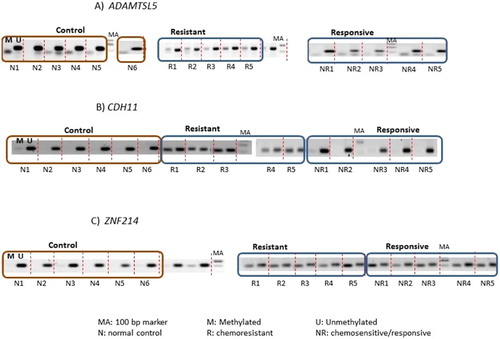
Discussion
The HumanMethylation27 BeadChip provided genome-wide analysis of 27 000 CpG sites from 14 000 human genes. Microarray results were reproducible with methylation-specific primers by PCR, suggesting that both methods were suitable for detecting methylation. Hypermethylation in cancer cells results from aberrant methylation of tumor suppressor genes. This affects key molecular pathways such as apoptosis, DNA repair pathways, cell cycle checkpoints, and cell differentiation as well as results in activation of metastasis/invasion pathways, drug resistance, and proliferation signal transduction [Citation8].
Applying highly stringent differential methylation options using the Partek Genomic Studio software identified ADAMTSL5 (ADAMTS-Like 5) and CDH11 as most hypermethylated in chemoresistant samples. Testing methylation status of these two genes on a larger number of samples confirmed the potential of using these genes in identifying chemoresistant patients before treatment. ADAMTSL5 has heparin binding and metalloendopeptidase activity. There is an inferred relationship between ADAMTSL5 and ADAMTS5. ADAMTS5 (ADAM Metallopeptidase with Thrombospondin Type 1 Motif, 5) is involved in degradation of the extracellular matrix. This gene encodes a member of the ADAMTS (a disintegrin and metalloproteinase with a thrombospondin motif) protein family which has antiangiogenic property. It also has roles in inflammatory process which can contribute to cachexia [provided by RefSeq, July 2008]. It is not clear whether other studies have identified significance of this gene in chemoresistant acute lymphoblastic leukemia. Another member of this group, ADAM23, however, is much recognized for involvement in cancer. ADAM23 belongs to the ADAM (a disintegrin and metalloproteinase domain) family of proteins. The ADAM23 gene can be silenced by DNA promoter methylation in some solid cancers. The progression of cancer and tumor cell mobility are enhanced by epigenetic inactivation. Epigenetic silencing has been implicated in breast carcinoma, lung carcinoma, gastric tumors, and pancreatic tumors [Citation17].
Our study also showed significant CDH11 hypermethylation in the drug-resistant group. Integrated genomic analysis of relapsed ALL identified deletion and/or hypermethylated and/or downregulated genes at relapse included many cadherins, such as CDH1, CDH11, and CD13 [Citation14]. These genes are inhibitors of the Wnt pathway and increased activation of β-catenin was observed at relapse in ALL samples. Inhibition of the Wnt pathway resulted in increased chemosensitivity [Citation18]. PCDH10 was found frequently hypermethylated in B-cell ALL. Cell lines harboring this aberration were less sensitive to commonly used leukemia-specific drugs, suggesting its potential as a drug-resistant marker [Citation19]. These findings support the potential of cadherin as a target for chemoresistance.
We found that ZNF214 hypermethylation may be suitable to identify B-ALL from normal samples but not as suitable to distinguish CR from chemoresistant B-ALL. This corresponded to results obtained from the microarray experiment. Increasing FDR stringency from 0.001 to 0.0001% selected for genes that better separated the two pre-B-ALL groups with different responses to chemotherapy. This also implied that the selection method using microarray on a small number of discovery cohort was sufficient to identify genes highly methylated and common to chemoresistant pre-B-ALL.
Conclusion
In summary, DNA hypermethylation in ADAMTSL5 and CDH11 was associated with chemoresistance in B-ALL. However, these results require validation on a larger number of patients. Further verification of its biological function and mechanism of action is also needed before differential methylation may be supported for its prognostic significance.
Supplementary_Data.docx
Download MS Word (1 MB)Disclosure statement
No potential conflict of interest was reported by the authors.
Notes on contributors
Dr. Maha Abdullah is a faculty member at Immunology Unit, Department of Pathology, UPM, Malaysia. She is an associate professor of Immunology.
Mr. Chee Wei Choo is a researcher at Department of Pathology, UKM Medical Center, Malaysia. He is an expert in epigenetics.
Dr. Hamidah Alias is a faculty member at Deparment of Paediatrics, UKM Medical Center, Malaysia. She is a professor of paediatrics.
Dr. Eni Juraidah Abdul Rahman is a consultant paediatrician at Institute of Paediatrics, Hospital Kuala Lumpur. She is an expert in paediatric oncology/hematology.
Dr. Hishamshah Mohd Ibrahim is a senior consultant paediatrician at Institute of Paediatrics, Hospital Kuala Lumpur. He is an expert in paediatric oncology/hematology.
Dr. Rahman Jamal is the director of UKM Medical Molecular Biology Insitute. He is a professor of paediatric oncology and molecular medicine.
Dr. Noor Hamidah is a faculty member at Hematology Unit, Department of Pathology, UKM Medical Center, Malaysia. She is a professor of haematopathology.
Additional information
Funding
References
- Pui CH. Recent advances in the biology and treatment of childhood acute lymphoblastic leukemia. Curr Opin Hematol. 1998;5(4):292–301. Epub 1998/09/25. doi: 10.1097/00062752-199807000-00009
- Pui CH, Sandlund JT, Pei D, et al. Improved outcome for children with acute lymphoblastic leukemia: results of Total Therapy Study XIIIB at St Jude Children's Research Hospital. Blood. 2004;104(9):2690–2696. Epub 2004/07/15. doi: 10.1182/blood-2004-04-1616
- Florean C, Schnekenburger M, Grandjenette C, et al. Epigenomics of leukemia: from mechanisms to therapeutic applications. Epigenomics. 2011;3(5):581–609. Epub 2011/12/01. doi: 10.2217/epi.11.73
- Esteller M, Garcia-Foncillas J, Andion E, et al. Inactivation of the DNA-repair gene MGMT and the clinical response of gliomas to alkylating agents. N Engl J Med. 2000;343(19):1350–1354. Epub 2000/11/09. doi: 10.1056/NEJM200011093431901
- Satoh A, Toyota M, Itoh F, et al. Epigenetic inactivation of CHFR and sensitivity to microtubule inhibitors in gastric cancer. Cancer Res. 2003;63(24):8606–8613. Epub 2003/12/26.
- Agrelo R, Cheng WH, Setien F, et al. Epigenetic inactivation of the premature aging Werner syndrome gene in human cancer. Proc Natl Acad Sci USA. 2006;103(23):8822–8827. Epub 2006/05/26. doi: 10.1073/pnas.0600645103
- Shen L, Kondo Y, Ahmed S, et al. Drug sensitivity prediction by CpG island methylation profile in the NCI-60 cancer cell line panel. Cancer Res. 2007;67(23):11335–11343. Epub 2007/12/07. doi: 10.1158/0008-5472.CAN-07-1502
- Burke MJ, Bhatla T. Epigenetic modifications in pediatric acute lymphoblastic leukemia. Front Pediatr. 2014;2(42):1–7. Epub 2014/05/27.
- Roberts KG, Mullighan CG. Genomics in acute lymphoblastic leukaemia: insights and treatment implications. Nat Rev Clin Oncol. 2015;12(6):344–357. Epub 2015/03/18. doi: 10.1038/nrclinonc.2015.38
- Almamun M, Levinson BT, van Swaay AC, et al. Integrated methylome and transcriptome analysis reveals novel regulatory elements in pediatric acute lymphoblastic leukemia. Epigenetics. 2015;10(9):882–890. Epub 2015/08/27. doi: 10.1080/15592294.2015.1078050
- Dunwell TL, Hesson LB, Pavlova T, et al. Epigenetic analysis of childhood acute lymphoblastic leukemia. Epigenetics. 2009;4(3):185–193. Epub 2009/05/12. doi: 10.4161/epi.4.3.8752
- Figueroa ME, Chen SC, Andersson AK, et al. Integrated genetic and epigenetic analysis of childhood acute lymphoblastic leukemia. J Clin Invest. 2013;123(7):3099–3111. Epub 2013/08/08. doi: 10.1172/JCI66203
- Gabriel AS, Lafta FM, Schwalbe EC, et al. Epigenetic landscape correlates with genetic subtype but does not predict outcome in childhood acute lymphoblastic leukemia. Epigenetics. 2015;10(8):717–726. Epub 2015/08/04. doi: 10.1080/15592294.2015.1061174
- Hogan LE, Meyer JA, Yang J, et al. Integrated genomic analysis of relapsed childhood acute lymphoblastic leukemia reveals therapeutic strategies. Blood. 2011;118(19):5218–5226. Epub 2011/09/17. doi: 10.1182/blood-2011-04-345595
- Nordlund J, Backlin CL, Wahlberg P, et al. Genome-wide signatures of differential DNA methylation in pediatric acute lymphoblastic leukemia. Genome Biol. 2013;14(9):r105–r100. Epub 2013/09/26. doi: 10.1186/gb-2013-14-9-r105
- Marshall OJ. PerlPrimer: cross-platform, graphical primer design for standard, bisulphite and real-time PCR. Bioinformatics. 2004;20(15):2471–2472. Epub 2004/04/10. doi: 10.1093/bioinformatics/bth254
- Costa ET, Camargo AA. ADAM23 (ADAM metallopeptidase domain 23). Atlas Genet Cytogenet Oncol Haematol in press; 2014.
- Dandekar S, Romanos-Sirakis E, Pais F, et al. Wnt inhibition leads to improved chemosensitivity in paediatric acute lymphoblastic leukaemia. Br J Haematol. 2014;167(1):87–99. Epub 2014/07/06. doi: 10.1111/bjh.13011
- Narayan G, Freddy AJ, Xie D, et al. Promoter methylation-mediated inactivation of PCDH10 in acute lymphoblastic leukemia contributes to chemotherapy resistance. Genes Chromosomes Cancer. 2011;50(12):1043–1053. Epub 2011/10/01. doi: 10.1002/gcc.20922