ABSTRACT
Objectives: The improved passive immune thrombocytopenia (ITP) mouse model has been extensively utilized for the study of ITP. However, how closely this model matches the human inflammation state and immune background is unclear. Our study aimed to explore the profile of Th cytokines and Th17/Treg cells in the model.
Methods: We induced the ITP mouse model by dose-escalation injection of MWReg30. The serum levels of cytokines (IFN-γ, IL-2, IL-4, IL-10, IL-17A, and TGF-β1) were measured by enzyme-linked immunosorbent assay and the frequency of Th17 and Treg cells was measured by flow cytometry. The mRNA expression of Foxp3 and RORrt was measured by real-time PCR.
Results: The serum levels of cytokines IFN-γ, TGF-β1, IL-4, and IL-10 were significantly lower in ITP mice. The secretion of serum proinflammatory cytokines IL-2 and IL-17A and the percentage of Th17 cells showed no statistically significant increase. In ITP mice the frequency of Treg cells and mRNA expression of Foxp3 was significantly lower in splenocytes.
Conclusion: Our data suggest that the improved passive ITP mouse model does not mimic the autoimmune inflammatory process of human ITP. Compared with human ITP, this model has a similar change in frequency of Treg cells, which may directly or indirectly result from antibody-mediated platelet destruction due to attenuated release of TGF-β.
Introduction
Immune thrombocytopenia (ITP) is an acquired autoimmune bleeding disorder in which circulating platelets are opsonized by IgG anti-platelet autoantibodies against platelet membrane glycoproteins, such as GPIIb/IIIa and GPIb/IX, and then destroyed in the reticuloendothelial system [Citation1]. To date, it is widely appreciated that not only autoreactive B lymphocytes but also dysfunctional T helper (Th) cells play a primary role in the pathogenesis of ITP [Citation2Citation[3] Citation[4] Citation–5]. The present studies have provided evidence suggesting that the imbalance of Th and regulatory T (Treg) cytokines can result in ITP [Citation6]. Th cells can be divided into Th1, Th2, and Th17 cells. Th1 cells mainly induce cellular immunity by producing interleukin-2 (IL-2) and interferon-γ (IFN-γ). These two proinflammatory cytokines are involved in the inflammatory response by activating macrophages, lymphocytes, and other inflammatory cells. In contrast, Th2 cells play a pivotal role in the inactivation of macrophages and the humoral immune response by secreting anti-inflammatory cytokines, such as interleukin-4 (IL-4) and interleukin-10 (IL-10). Th17 and Treg cells are distinct from Th1 and Th2 cells. Th17/Treg imbalance plays a major role in the development and outcomes of autoimmune/inflammatory diseases, such as systemic lupus erythematosus, rheumatoid arthritis, and asthma [Citation7]. Th17 cells, the cellular source of interleukin-17 (IL-17), participate in inflammatory reactions and autoimmunity. Treg cells are a subset of CD4+ lymphocytes that have high expression of both CD25 and the transcription factor forkhead box P3 (Foxp3). They secrete suppressor cytokines IL-10 and transforming growth factor (TGF-β) and are responsible for maintaining immune tolerance and homeostasis.
There have been several animal models of ITP, and mouse models have quickly emerged as the primary animal model for human ITP [Citation8Citation[9] Citation[10] Citation[11] Citation[12] Citation[13] Citation[14] Citation[15] Citation–16]. In recent years, passive transfer of anti-platelet antibodies has been extensively utilized in ITP models to initiate and maximize the thrombocytopenia [Citation13Citation[14] Citation[15] Citation–16]. Although, there are a variety of models for human ITP, they may only capture some, but not all, aspects of ITP onset and/or progression. It is still unclear which aspects of the autoimmune inflammatory disorders are accurately reflected in published models of ITP that are widely used. Until now, comparisons of the improved passive mouse model to human ITP have failed to address whether or not immune-mediated platelet destruction can affect the immune background and whether this published model can accurately reflect the inflammatory state of human ITP. Both of these are important for examining mechanisms of different treatment modalities.
In our study, to assess this improved mouse model, which is also known as the dose-escalation ITP mouse model induced by administration of anti-mouse antibody, we examined the Th17/Treg cells and the cytokine profiles in the model. To further investigate the possible underlying mechanisms of Th17/Treg cells in the ITP mouse, we also measured mRNA expression of Foxp3 and RORrt in sorted Treg cells and Th17 cells, respectively.
Materials and methods
Mice
Six- to eight-week-old female inbred BALB/c mice weighing 18–20 g were purchased from the Laboratory Animal Center in Medical Center of Sun Yat-sen University. The mice were randomly assigned to two groups of 10: an ITP group and a normal control group. Animal studies were approved by the Sun Yat-sen University Medical Center Ethics Committee for Animal Experiments.
Induction of passive immune thrombocytopenia mouse model
We induced the dose-escalation passive ITP mouse model by administering rat anti-mouse CD41 antibody at a dose that was increased daily to achieve sustained platelet destruction throughout the time course of the model, as previously described [Citation15]. The ITP group received daily intraperitoneal injections of monoclonal antibody MWReg30 (eBioscience, San Diego, CA, U.S.A.; recognizing CD41) diluted in 200 μl of PBS (Gibco, Grand Island, NY, U.S.A.). The dose of MWReg30 antibody was 68 μg/kg on Days 1 and 2, 102 μg/kg on Day 3, and 136 μg/kg from Day 4 to Day 8. The normal control group received injections of the same dose of IgG1 with an equal volume of PBS. Twenty microliters of whole blood was collected from the tail vein into microvette tubes prefilled with EDTA-Na2 and diluted in PBS (pH 7.2, Gibco) to a final blood dilution of 1:7. PLT counts were determined using an automatic blood cell counter (Model LH750 hematology analyzer, Beckman Coulter). From Day 1 to Day 8, blood samples were obtained before the MWReg30 antibody injection.
Measurement of cytokines serum levels
On Day 8, retro-orbital blood collection was performed for cytokine examination using the following method [Citation17]: Mice were anesthetized with isoflurane and placed on the tabletop. The eyelids were gently retracted to protrude the globe of the eye. A fine-walled Pasteur pipette was inserted into the corner of the eye socket at an angle of 45°. The pipette was rotated, and gentle downward pressure was applied until the vein was broken and blood was visualized flowing into the pipette. Then, we collected whole blood in EDTA sterile tubes and centrifuged the tubes at 2500 × g for 15 min to separate the plasma. The plasma samples were stored at −80°C for subsequent cytokine analysis. Serum levels of cytokines (IL-2, IFN-γ, IL-4, IL-10, IL-17, and TGF-β1) in the two groups were determined by enzyme-linked immunosorbent assays (ELISAs) as described in the manufacturer’s instructions (Neobioscience, Shenzhen, China). Absorbance was measured at 450 nm.
Determination of Th17 and Treg cells
Whole blood was obtained by enucleation, and single cell suspensions were prepared from spleens on Day 8. For detection of CD4+CD25+FoxP3+Treg cells in the peripheral blood and spleen, samples were fixed, permeabilized, and incubated with fluorescein isothiocyanate (FITC)-labeled monoclonal anti-CD4, phycoerythrin (PE)-labeled monoclonal anti-CD25 and phycoerythrin-labeled monoclonal anti-FoxP3 (eBioscience). CD25 high Foxp3+ cells gated in the CD4+ cell fraction were recorded as Tregs. For detection of Th17 cells, the cell suspensions were stimulated for 5 h with 25 ng/ml phorbol 12-myristate 13-acetate (Sigma-Aldrich, St Louis, MO, U.S.A.), 1 μg/ml ionomycin (Sigma-Aldrich), and 500 ng/ml monensin (Sigma-Aldrich). After that, samples were fixed, permeabilized, and incubated with FITC-labeled monoclonal anti-CD4 (eBioscience), PE-labeled monoclonal anti-IL-17A (Biolegend, San Diego, CA, U.S.A.). Cells incubated with a species- and isotype-matched mAb to an irrelevant antigen were used for negative controls. IL-17A+ cells gated in the CD4+ cell fraction were recorded as Th17 cells. The Th17/Treg cells were examined on a FACS Calibur flow cytometer (BD Biosciences) using the Cell Quest software.
Isolation of highly purified CD4+CD25+T cell subsets of spleen
Magnetic cell sorting was used to isolate the spleen CD4+CD25+ T cells. Specifically, a mouse CD4+CD25+ regulatory T cell isolation kit (R&D, U.S.A.) was used according the manufacturer’s instructions. CD4+CD25+ T cells were isolated by negative selection of CD4+ T cells followed by positive selection of CD25+ T cells from the CD4+ population. Flow cytometry was used to measure the purity of isolated cells, which was 85%. Trypan blue staining was used to measure the activity of the sorted cells, which was 95%.
Analysis of mRNA expression of Foxp3 and RORrt
The mRNA expression levels of Th17/Treg associated transcription factors from spleen mononuclear cells were measured by real-time PCR. Total RNA was extracted with RNAiso Plus (Takara, Shiga, Japan). Then, cDNA was synthesized with PrimeScript® RT Master Mix (Takara) as described in the manufacturer’s instructions. Quantitative Real-time PCR was performed using TaqMan Premix Ex Taq™ (Perfect Real-Time) master mix (Takara). shows all PCR primer pairs. The Real-Time PCR was carried out in duplicate wells using a Light Cycle® Real Time machine (Roche Diagnostic, Branchburg, NJ, U.S.A.), and the Ct values for target genes and the housekeeping gene (GAPDH) were calculated. The levels of expression were normalized to GAPDH, and the fold changes in expression of transcription factor genes in ITP mice compared to control mice were calculated.
Table 1. Primer sequences used for real-time PCR.
Statistical analysis
All the data were expressed as the means ± s.d. and assessed using the unpaired Student’s t-test. A Kolmogorov–Smirnov goodness-of-fit model was used to assess the normality of the data. All the data showed a normal distribution. Tests were performed using SPSS software version 20.0 for Windows. A P-value of <0.05 was considered statistically significant.
Results
Immune thrombocytopenia mouse model
As shown in , BALB/c mice injected with the monoclonal PLT antibody (MWReg30) became thrombocytopenic; PLT counts were reduced to half of the initial values by 24 h and were relatively constant from Day 3 to Day 8. At the same time, 10 mice were injected with IgG as controls. PLT counts in control mice remained stable at 1200 × 109 throughout the study. In the study, we successfully established 10 ITP mice ().
Figure 1. Platelet counts in mice at different times after the injection of MWReg30 monoclonal antibody. BALB/c mice were injected with 1.4 µg MWReg30 antibody on Days 1 and 2, 2.1 μg on Day 3, and 2.8 μg from Day 4 to Day 8. The control group was injected with the same volume of IgG1. The PLT count was reduced to half the initial value by 24 h and the PLT count was constant from Day 3 to Day 8 in the ITP mouse model (n = 10 mice/group).
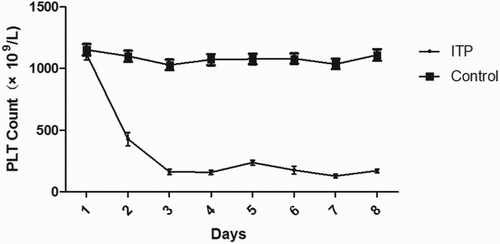
Serum Th and Treg cytokine levels
shows the results of cytokine analysis in the ITP and control groups. We found that the levels of the Treg cytokine (TGF-β1) and Th2 cytokines (IL-4, IL-10) were significantly lower in the ITP mouse model (P = 0.012 for TGF-β1, P = 0.021 for IL-4, and P = 0.019 for IL-10). The secretion of serum Th1 cytokine (IL-2) and Th17 cytokine (IL-17A) showed no statistically significant difference between the two groups (P = 0.112 for IL-2 and P = 0.357 for IL-17A). There was, however, a statistically significant decrease in the secretion of IFN-γ in the ITP mouse model (P = 0.001) compared to the normal mice ().
Frequency of Treg and Th17 cells
The peripheral blood and spleen mononuclear cells were screened by flow cytometry for the presence of Treg cells. As shown in , the percentage of Treg cells was significantly lower in the splenocytes and peripheral blood of ITP mice than in normal controls (peripheral blood: 4.80 ± 0.26% vs. 6.55 ± 0.62%, P = 0.018; spleen: 10.25 ± 0.55% vs. 14.27 ± 0.50%, P = 0.001, respectively). Although the percentage of Th17 cells was higher in ITP mice than in control mice (: peripheral blood: 0.82 ± 0.17% vs. 0.62 ± 0.20%, P = 0.47; spleen: 1.15 ± 0.22% vs. 1.09 ± 0.40%, P = 0.92, respectively), the differences were not statistically significant ().
Figure 3. CD4+CD25+Foxp3+ Treg and Th17 cells in peripheral blood and spleen of ITP and control groups. (a) Flow cytometric analysis of Treg and Th17 cells. The lymphocytes were first selected based on their side and forward scatters. The CD25+Foxp3+ double-positive cells were defined in the CD4 gate and considered the Treg population. The CD4+IL17+ positive cells were defined as Th17 populations; (b) Box plots represent the percentage of Treg and Th17 cells in ITP mice and controls. *P < 0.05. **P < 0.01. vs. normal controls.
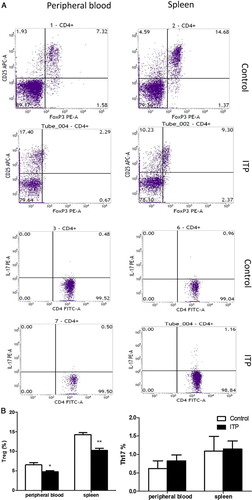
mRNA expression levels of Foxp3 and RORrt
We analyzed the mRNA expression levels of transcription factors associated with Th17/Treg cells in CD4+CD25+ cells and Th17 cells. Our results showed a significant reduction in mRNA expression of Foxp3 in ITP mice (0.067 ± 0.012 vs. 0.137 ± 0.008, P = 0.001). The mRNA expression of RORrt was not significantly different between the two groups (0.0141 ± 0.0020 vs. 0.0135 ± 0.0022, P = 0.86).
Discussion
ITP is a common autoimmune blood disorder characterized by impaired platelet production, and platelet over-destruction is mediated by anti-platelet antibodies [Citation1,Citation18]. There are some animal models for understanding the mechanisms and exploring treatment modalities of ITP disease; mouse models have been often used. The passive antibody-induced mouse model is widely employed for the study of ITP. This model can become thrombocytopenic as a result of immune-mediated PLT destruction within 24 h of injection and reach a PLT nadir with continuous daily anti-CD41 administration. However, the conventional passive model may cause a PLT rebound on Day 4. Katsman et al. [Citation15] explored an alternative improved model, dose-escalation mouse model of ITP, which could maintain the PLT nadir throughout the course of the experiment. Thus, this model has been extensively utilized in understanding the onset and progression of chronic ITP. In our study, we successfully induced a dose-escalation passive ITP mouse model with very low levels of PLT counts for 8 days. Our work was undertaken to investigate the changes in the immunological milieu (CD4+ T cells and cytokine secretion profile) in the improved passive mouse model of ITP due to antibody-mediated platelet destruction and explore whether the experimental ITP model could accurately mirror the human condition.
One conclusion of our data was that we documented no inflamed microenvironment in the passive ITP mouse model, suggesting that autoreactive CD4+ Th cells were not activated. It suggests that this model cannot exactly mimic the inflammatory process of human ITP. Recent studies have mainly focused on two aspects of the immune dysregulation in ITP: activated platelet-autoreactive T cells with increasing proinflammatory cytokines and impaired immune tolerance with decreasing Treg cells [Citation19Citation[20] Citation[21] Citation–22]. Several studies have observed that Th1 cytokines (IFN-g and IL-2) increased, while Th2 cytokines (IL-4 and IL-10) decreased in ITP patients, implying a Th1-dominated cytokine profile in ITP [Citation23]. Th17 cells represent a proinflammatory subset, which have been associated with numerous autoimmune diseases. More recently, increasing evidence has suggested that there is an increase in Th17 cells or IL-17 cytokine levels in ITP patients [Citation24]. These data suggest an inflammatory microenvironment in human ITP disease. Our results showed that the Th2 cytokines (IL-4 and IL-10) were decreased as expected. However, instead of the increased inflammatory factors, Th1 cytokine levels (IFN-γ) were reduced and there was no statistically significant difference in Th1 and Th17 cytokine levels (IL-2, IL-17A) in the passive ITP mouse model. Furthermore, we assessed the frequency of Th17 cells in both peripheral blood and the spleen. Our results showed no difference between the ITP mouse model and the control.
On one hand, this ITP mouse model is only based on passive transfer of anti-platelet antibodies, but human ITP is an acquired autoimmune disease influenced by many factors. On the other hand, the ITP mouse model is a model of antibody-mediated platelet destruction that presents the innate response phase of human ITP disease. In contrast, the adaptive autoimmunity mechanisms are responsible for the development of human ITP. Excessive platelet phagocytosis by macrophages might lead to the impaired capacity of autoantigen processing and presenting to CD4+ Th cells with passive daily administration of anti-CD41. Therefore, a better animal ITP model may be the alloimmune ITP model, which was induced by splenocytes of CD61 knockout mice immunized against platelets from CD61+ mice into severe SCID mice [Citation25,Citation26]. This model may better reflect the inflammatory phase of human ITP.
Another important finding of our study was that we found the frequency of CD4+CD25+Foxp3+ Treg cells both from peripheral blood and the spleen, as well as the inhibitory cytokine TGF-β1, to be markedly decreased in the improved mouse ITP model, which is consistent with previous studies [Citation19,Citation27Citation[28] Citation[29] Citation–30]. Because this model is induced by the passive transfer of anti-platelet antibodies, anything that is seen in the innate and adaptive immune system should be a result of antibody-mediated platelet destruction. Our hypothesis is that as platelet counts decrease, there is decreased release of TGF-β, resulting in decreased Treg cell counts.
As reported, platelets contain more TGF-β1 than other cell types, and TGF-β1 can be released by platelet activation/degranulation or from megakaryocytes [Citation31,Citation32]. It is possible that with decreased platelet counts in mice, there is attenuated release of TGF-β1. More studies on the direct correlation between TGF-β1 levels and platelet counts are still needed. In regulating the immune response, especially in adaptive immunity, the cytokine TGF-β plays an integral role. TGF-β can be a potent inhibitor of T/B cell proliferation and autoantibody production [Citation33]. Furthermore, TGF-β also contributes to the suppression of the T cell responses by regulating Foxp3+ Tregs, and it is essential to the development of nTreg and iTreg [Citation34]. We hypothesize that the decrease in Treg cell counts in the ITP mouse model is mediated directly or indirectly by TGF-β1 released by platelets and/or megakaryocytes. Treg cells maintain peripheral tolerance by both cell-contact-dependent and cell-contact-independent mechanisms [Citation35]. The change of Treg cells in the model, however, does not involve ITP autoimmune pathophysiology such as immune tolerance. Whether the decrease in the frequency of Treg cells in this passive model is only a consequence of platelet destruction or whether it actually also contributes to the decrease in platelet count requires further investigation.
To further investigate the possible mechanisms causing deficient Treg cells in ITP mouse, we measured the mRNA expression levels of key transcription factors that play an important role in the proliferation and differentiation of Treg cells in the ITP mouse model. Foxp3 is known as a specific molecular marker for Treg cells and is indispensable in the development and function of Treg cells [Citation36]. Mice and humans carrying an inactivating mutation in the Foxp3 present with severe inflammation and autoimmune diseases due to Treg deficiency. Li et al. showed that Foxp3 was significantly down-regulated in ITP patients, and dexamethasone corrected the Treg cell count by promoting Foxp3 expression [Citation37]. A decrease in spleen CD4+CD25+ Tregs of ITP mice was also found in our study. This may partly explain the change in Treg cell counts in the model.
Taken together, we observed cytokine profiles (Th and Treg) and Th17/Treg cells in the improved dose-escalation antibody-induced mouse model for the first time. Our data suggest that the improved passive model does not match the inflammatory state of human ITP but has the similar changes in Treg and TGF-β, although the causes may be different. The passive ITP mouse model has been utilized to understand the underlying mechanism of new and existing therapeutic modalities, especially related to the role of immunoglobulins in ITP disease, and it may be useful for evaluating novel therapeutic strategies that use Treg cells as therapeutic targets.
The different animal models of ITP excel in their ability to recapitulate various aspects of this autoimmune disorder, including the nature of the autoantigen, the breakdown of tolerance, the dysfunction of several immune system components, the inflammatory response, and the kinetics and mechanisms of destruction and production of platelets [Citation16]. However, there are still significant differences and potential shortcomings of these current mouse models as they relate to human ITP.
Ethics approval
This study was approved by the Sun Yat-sen University Medical Center Ethics Committee for Animal Experiments.
Acknowledgments
We thank the Lim Por Yen Medical Research Center of Sun Yat-sen Memorial Hospital (Guangdong, China) for their equipment support and technical guidance.
Disclosure statement
No potential conflict of interest was reported by the authors.
Notes on contributors
Guoyang Zhang, a medical postgraduate student of Sun Yat-sen Memorial Hospital, Sun Yat-sen University, has research interests in bleeding and coagulation.
Ping Zhang, a resident doctor, has research interests in bleeding and coagulation.
Hongyun Liu, a resident doctor, has research interests in bleeding and coagulation.
Xiaoyan Liu, a resident doctor, has research interests in platelet function and infection.
Shuangfeng Xie, an associate professor, has research interest in blood malignancies.
Xiuju Wang, an associate professor, has research interest in multiple myeloma.
Yudan Wu, an associate professor, has research interest in lymphoma.
Jianxing Chang, an associate professor, has research interest in oncology.
Liping Ma, a professor, has research interests in bleeding and coagulation, blood malignancies.
Additional information
Funding
References
- Cines DB, Bussel JB, Liebman HA, et al. The ITP syndrome: pathogenic and clinical diversity. Blood. 2009;113:6511–6521. doi: 10.1182/blood-2009-01-129155
- Semple JW, Freedman J. Increased antiplatelet T helper lymphocyte reactivity in patients with autoimmune thrombocytopenia. Blood. 1991;78:2619–2625.
- Semple JW, Milev Y, Cosgrave D, et al. Differences in serum cytokine levels in acute and chronic autoimmune thrombocytopenic purpura: relationship to platelet phenotype and antiplatelet T-cell reactivity. Blood. 1996;87:4245–4254.
- Zhang J, Ma D, Zhu X, et al. Elevated profile of Th17, Th1 and Tc1 cells in patients with immune thrombocytopenic purpura. Haematologica. 2009;94:1326–1329. doi: 10.3324/haematol.2009.007823
- Yazdanbakhsh K, Zhong H, Bao W. Immune dysregulation in immune thrombocytopenia. Semin Hematol. 2013;50(Suppl 1):S63–S67. doi: 10.1053/j.seminhematol.2013.03.011
- Talaat RM, Elmaghraby AM, Barakat SS, et al. Alterations in immune cell subsets and their cytokine secretion profile in childhood idiopathic thrombocytopenic purpura (ITP). Clin Exp Immunol. 2014;176:291–300. doi: 10.1111/cei.12279
- Noack M, Miossec P. Th17 and regulatory T cell balance in autoimmune and inflammatory diseases. Autoimmun Rev. 2014;13:668–677. doi: 10.1016/j.autrev.2013.12.004
- Oyaizu N, Yasumizu R, Miyama-Inaba M, et al. (NZW x BXSB)F1 mouse. A new animal model of idiopathic thrombocytopenic purpura. J Exp Med. 1988;167:2017–2022. doi: 10.1084/jem.167.6.2017
- Musaji A, Vanhoorelbeke K, Deckmyn H, et al. New model of transient strain-dependent autoimmune thrombocytopenia in mice immunized with rat platelets. Exp Hematol. 2004;32:87–94. doi: 10.1016/j.exphem.2003.09.024
- Chow L, Aslam R, Speck ER, et al. A murine model of severe immune thrombocytopenia is induced by antibody- and CD8+ T cell-mediated responses that are differentially sensitive to therapy. Blood. 2010;115:1247–1253. doi: 10.1182/blood-2009-09-244772
- Dekel B, Marcus H, Shenkman B, et al. Human/BALB radiation chimera engrafted with splenocytes from patients with idiopathic thrombocytopenic purpura produce human platelet antibodies. Immunology. 1998;94:410–416. doi: 10.1046/j.1365-2567.1998.00516.x
- Corash L, Levin J. The relationship between megakaryocyte ploidy and platelet volume in normal and thrombocytopenic C3H mice. Exp Hematol. 1990;18:985–989.
- Song S, Crow AR, Freedman J, et al. Monoclonal IgG can ameliorate immune thrombocytopenia in a murine model of ITP: an alternative to IVIG. Blood. 2003;101:3708–3713. doi: 10.1182/blood-2002-10-3078
- Crow AR, Song S, Semple JW, et al. IVIg inhibits reticuloendothelial system function and ameliorates murine passive-immune thrombocytopenia independent of anti-idiotype reactivity. Br J Haematol. 2001;115:679–686. doi: 10.1046/j.1365-2141.2001.03136.x
- Katsman Y, Foo AH, Leontyev D, et al. Improved mouse models for the study of treatment modalities for immune-mediated platelet destruction. Transfusion. 2010;50:1285–1294. doi: 10.1111/j.1537-2995.2009.02558.x
- Neschadim A, Branch DR. Mouse models of autoimmune diseases: immune thrombocytopenia. Curr Pharm Des. 2015;21:2487–2497. doi: 10.2174/1381612821666150316123436
- Fried JH, Worth DB, Brice AK, et al. Type, duration, and incidence of pathologic findings after retroorbital bleeding of mice by experienced and novice personnel. J Am Assoc Lab Anim Sci. 2015;54:317–327.
- McMillan R. Autoantibodies and autoantigens in chronic immune thrombocytopenic purpura. Semin Hematol. 2000;37:239–248. doi: 10.1016/S0037-1963(00)90102-1
- Yu J, Heck S, Patel V, et al. Defective circulating CD25 regulatory T cells in patients with chronic immune thrombocytopenic purpura. Blood. 2008;112:1325–1328. doi: 10.1182/blood-2008-01-135335
- Sakakura M, Wada H, Tawara I, et al. Reduced CD4+CD25+ T cells in patients with idiopathic thrombocytopenic purpura. Thromb Res. 2007;120:187–193. doi: 10.1016/j.thromres.2006.09.008
- Nishimoto T, Kuwana M. CD4+CD25+Foxp3+ regulatory T cells in the pathophysiology of immune thrombocytopenia. Semin Hematol. 2013;50(Suppl 1):S43–S49. doi: 10.1053/j.seminhematol.2013.03.018
- Liu H, Ouyang X, Li Y, et al. Involvement of levels of Toll like receptor-4 in monocytes, CD4+ T-lymphocyte subsets, and cytokines in patients with immune thrombocytopenic purpura. Thromb Res. 2013;132:196–201. doi: 10.1016/j.thromres.2013.04.025
- Guo C, Chu X, Shi Y, et al. Correction of Th1-dominant cytokine profiles by high-dose dexamethasone in patients with chronic idiopathic thrombocytopenic purpura. J Clin Immunol. 2007;27:557-562. doi: 10.1007/s10875-007-9111-1
- Hu Y, Ma DX, Shan NN, et al. Increased number of Tc17 and correlation with Th17 cells in patients with immune thrombocytopenia. PLoS One. 2011;6:e26522. doi: 10.1371/journal.pone.0026522
- Chow L, Aslam R, Speck ER, et al. A murine model of severe immune thrombocytopenia is induced by antibody- and CD8+ T cell-mediated responses that are differentially sensitive to therapy. Blood. 2010;115:1247–1253. doi: 10.1182/blood-2009-09-244772
- Aslam R, Hu Y, Gebremeskel S, et al. Thymic retention of CD4+CD25+FoxP3+ T regulatory cells is associated with their peripheral deficiency and thrombocytopenia in a murine model of immune thrombocytopenia. Blood. 2012;120:2127–2132. doi: 10.1182/blood-2012-02-413526
- Bao W, Bussel JB, Heck S, et al. Improved regulatory T-cell activity in patients with chronic immune thrombocytopenia treated with thrombopoietic agents. Blood. 2010;116:4639–4645. doi: 10.1182/blood-2010-04-281717
- Arandi N, Mirshafiey A, Jeddi-Tehrani M, et al. Alteration in frequency and function of CD4(+)CD25(+)FOXP3(+) regulatory T cells in patients with immune thrombocytopenic purpura. Iran J Allergy Asthma Immunol. 2014;13:85–92.
- Liu B, Zhao H, Poon MC, et al. Abnormality of CD4(+)CD25(+) regulatory T cells in idiopathic thrombocytopenic purpura. Eur J Haematol. 2007;78:139–143.
- Ji L, Zhan Y, Hua F, et al. The ratio of Treg/Th17 cells correlates with the disease activity of primary immune thrombocytopenia. PLoS One. 2012;7:e50909. doi: 10.1371/journal.pone.0050909
- Assoian RK, Komoriya A, Meyers CA, et al. Transforming growth factor-beta in human platelets. Identification of a major storage site, purification, and characterization. J Biol Chem. 1983;258:7155–7160.
- Fortunel NO, Hatzfeld A, Hatzfeld JA. Transforming growth factor-beta: pleiotropic role in the regulation of hematopoiesis. Blood. 2000;96:2022–2036.
- Cross D, Cambier JC. Transforming growth factor beta 1 has differential effects on B cell proliferation and activation antigen expression. J Immunol. 1990;144:432–439.
- Travis MA, Sheppard D. TGF-β Activation and Function in Immunity. Annu Rev Immunol. 2014;32:51–82. doi: 10.1146/annurev-immunol-032713-120257
- Josefowicz SZ, Lu LF, Rudensky AY. Regulatory T cells: mechanisms of differentiation and function. Annu Rev Immunol. 2012;30:531–564. doi: 10.1146/annurev.immunol.25.022106.141623
- Cretney E, Kallies A, Nutt SL. Differentiation and function of Foxp3+ effector regulatory T cells. Trends Immunol. 2013;34:74–80. doi: 10.1016/j.it.2012.11.002
- Li J, Wang Z, Hu S, et al. Correction of abnormal T cell subsets by high-dose dexamethasone in patients with chronic idiopathic thrombocytopenic purpura. Immunol Lett. 2013;154:42–48. doi: 10.1016/j.imlet.2013.08.006