ABSTRACT
Background: Classical BCR-ABL1-negative myeloproliferative neoplasms (MPNs) including polycythemia vera, essential thrombocythemia (ET), and primary myelofibrosis frequently harbor JAK2, MPL, and CALR somatic mutations.
Methods: AS-PCR for JAK2 V617F, pyrosequencing for MPL W515L/K, and PCR-fragment analysis for CALR exon 9 mutations were established to analyze genomic DNA isolated from peripheral blood samples of 58 newly diagnosed ET patients in Thailand.
Results: JAK2 V617F was detected in 41 patients (71%) and CALR exon 9 mutation was positive in eight patients (14%), whereas no mutation of MPL W515L/K was observed in this study. Patients with CALR mutation were older (p = 0.023) and exhibited lower number of platelet count (p = 0.041) than patients without CALR mutation. Two previously known CALR mutation types were identified in this study (six patients with CALR-type 1 and two patients with CALR-type 2). Additionally, no co-existence of JAK2 V617F and CALR mutations was identified in this work.
Conclusion: We reported the frequency of JAK2 V617F, MPL W515L/K, and CALR mutations in Thai patients with ET. Clinical and hematological phenotypes of patients were associated with JAK2 and CALR mutation statuses. The combination of laboratory testing for the detection of JAK2, CALR, and MPL mutations is necessary to improve the diagnosis and classification of BCR-ABL1-negative MPN.
Introduction
Myeloproliferative neoplasm (MPN) is a heterogeneous group of clonal hematopoietic stem disorder characterized by the abnormal proliferation of single- or multi-lineage myeloid cells in bone marrow. In addition to chronic myeloid leukemia (CML) with BCR-ABL1 fusion gene, there are three common BCR-ABL1-negative MPNs including polycythemia vera (PV), essential thrombocythemia (ET), and primary myelofibrosis (PMF). Since the discovery of Janus kinase 2 (JAK2) mutations in a majority of PV and about half of patients with ET and PMF, these markers have significantly improved the diagnostic criteria for the classification of MPNs [Citation1–3]. Presently, the detection of JAK2 V617F mutation is included into the WHO classification of myeloid neoplasms and acute leukemia [Citation4]. About 90% of the patients with PV harbor JAK2 V617F mutation, whereas approximately to 50–60% of patients with ET or PMF harbor JAK2 V617F mutation [Citation2,Citation3,Citation5–7]. In addition, the activating mutations of the thrombopoietin receptor gene, MPL (mainly W515L/K) have been reported in about 5% of patients with ET and PMF [Citation8,Citation9]. Recently, Klampfl et al. and Nangalia et al., revealed that calreticulin (CALR) mutations were frequently identified in patients with MPN without JAK2 and MPL mutations (about 20–25% of all ET and PMF and none in PV) [Citation10,Citation11]. CALR is located on the short arm of chromosome 19 (19p13.13) and encodes protein which is involved in several critical cellular functions including Ca2+ binding (Ca2+ homeostasis) and cellular signaling processes [Citation12]. In MPNs, somatic CALR mutations with insertion or deletion (indel), resulting in frameshift mutations, frequently occur at the terminal sequences of the exon 9. At present, there are about 70 types of CALR exon 9 mutations identified in MPNs. CALR type 1 (52 base pairs deletion; about 50%) and type 2 (5 base pairs insertion; about 30%) mutations are recognized as the two majors of CALR mutations identified in patients when compared with other types of CALR mutations [Citation10,Citation11,Citation13–15]. CALR type 1 mutation is associated with PMF, whereas CALR type 2 mutation is associated with ET phenotype [Citation13]. Additionally, low hemoglobin and leukocyte count, high platelet number, and low risk of thrombolytic events were observed in ET patients with CALR mutations [Citation16,Citation17]. Consequently, these findings highlight the pathogenesis of BCR-ABL1-negative MPN driving by CALR mutations and the usefulness applications of CALR as a molecular biomarker for the diagnosis and classification of MPNs.
In this study, we established the PCR-Fragment analysis and conventional Sanger sequencing method for the detection of CALR exon 9 mutations in JAK2 V617F-negative ET patients. We described the prevalence and types of somatic CALR mutations in this study. Finally, we investigated the impacts of CALR mutations on clinical and hematological phenotypes in individual patients.
Materials and methods
Patients and samples
A total of 58 peripheral blood samples from newly diagnosed ET patients at Ramathibodi Hospital, Bangkok, Thailand were included in this study from 2013 to 2016. The diagnostic criteria were based on the 2008 WHO classification of myeloid neoplasms and acute leukemia. Hematological findings of individual patients, including hemoglobin and hematocrit levels, leukocyte count, and platelet amount, were recorded. Genomic DNA was isolated by QIAamp DNA Blood mini kit (Qiagen, Hilden, Germany) according to the manufacturer’s instruction and the concentration was subsequently measured by the Nanodrop 2000 spectrophotometer (Thermo Scientific, Waltham, MA, U.S.A.) according to the instruction protocol. The JAK2 V617F status of all tested samples was determined by the allele specific polymerase chain reaction (AS-PCR), as described in the previous published protocol [Citation2]. This study was approved by the ethic committee on human right related to research involving human subjects, Faculty of Medicine Ramathibodi Hospital, Mahidol University, Thailand and followed the principles of the Declaration of Helsinki (ID 03-59-59).
MPL W515L/K mutation analysis
We performed pyrosequencing technique to detect the hot spot mutation of MPL, W515L/K using the recent published protocol by our group [Citation18]. In brief, the optimal PCR reaction contained 1X PyroMark PCR master mix (Qiagen, Hilden, Germany), 1X CollaLoad Concentrate, 0.2 μM of each primer and 40.0 ng of gDNA in a total volume of 25 μl. The PCR primers used were MPL-forward 5′-CCGCTCTGCATCTAGTGCT-3′ and MPL-reverse 5′-biotin-CTGTAGTGTGCAGGAAACTG-3′. The amplification was carried out in the Veriti Thermal Cycler (Applied Biosystems, Foster City, CA, U.S.A.). The optimal PCR condition was as follows: initial denaturing at 95°C for 10 minutes, 40 cycles of denaturing at 95°C for 20 seconds, annealing at 59°C for 30 seconds, and extension at 72°C for 5 minutes. PCR products were analyzed on 2% gel electrophoresis prior to pyrosequencing analysis. The DNA templates were immobilized on the streptavidin-coated Sepharose high-performance beads (GE Health care, Sweden) using the PyroMark Q24 Vacuum Workstation (Qiagen, Hilden, Germany) according to the manufacture’s instruction. The pyrosequencing primer was 5′-TGCTGCTGCTGAGGT-3′. The pyrosequencing reaction was performed using the PyroGold Reagents (Biotage) and the PyroMark Q24 instrument was used according to the manufacture’s instruction. The pyrogram data were analyzed using the PyroMark Q24 software (Biotage). Finally, the mutation load (allele burden) was calculated using allele quantification (AQ) software.
CALR exon 9 mutation analysis
PCR genotyping of CALR exon 9 mutations was performed according to Klampfl et al. [Citation10]. In brief, PCR condition had the following parameters: initial denaturing at 95°C for 10 minutes, 10 cycles of 94 °C for 15 seconds, 55°C for 15 seconds, 72°C for 30 seconds, 20 cycles of 89°C for 15 seconds, 55°C for 15 seconds, 72°C for 30 seconds, and final extension at 72°C for 20 minutes. Forward and reverse primer sequences were 6-FAM-5′-GGCAAGGCCCTGAGGTGT-3′ and 5′-GGCCTCAGTCCAGCCCTG-3′, respectively. PCR products were measured on the 3130xl Genetic Analyzer (Applied Biosystems, Foster City, CA, U.S.A.) and subsequently analyzed using the Gene Mapper software version 4.0 (Applied Biosystems, Foster City, CA, U.S.A.). Sanger sequencing was performed on genomic DNA of patients who were positive for CALR exon 9 mutation. Sequencing primers were as follows: CALR-F2-5′ACAACTTCCTCATCACCAACG-3′ and CALR-R2-5′-GGCCTCAGTCCAGCCCTG-3′. Sequencing reaction was performed using BigDye Terminator (version 3.1) Cycle Sequencing Kit (Life Technologies). The sequencing products were run on the 3130xl Genetic Analyzer (Applied Biosystems, Foster City, CA, U.S.A.) and subsequently analyzed with SeqScape® software version 2.5 (Thermo Fisher Scientific, Waltham, MA, U.S.A.).
Statistical analysis
The statistical analysis of obtained data was performed using the SPSS version 16.0 (SPSS Inc, Chicago, IL, U.S.A.) software. Mann–Whitney U test was used for the comparison of demographic data and laboratory findings. Chi-squared test was used for the comparison of categorical variables. The p-value of less than 0.05 was considered to indicate statistical significance.
Results
Clinical and laboratory characteristics of 58 ET patients
We analyzed the mutations of JAK2 V617F, CALR exon 9, and MPL W515L/K and studied the impact of those mutations on the demographic data and clinical findings of 58 newly diagnosed ET patients. While MPL W515L/K was not positive in all tested samples, JAK2 V617F mutation was positive in 41 of 58 (71%) patients and frameshift mutation of CALR exon 9 was positive in 8/58 (14%) of patients (). There were not statistically significant differences in all tested parameters, including sex, hemoglobin, hematocrit, leukocyte count, and platelet count, between JAK2 V617F-positive and -negative patients ( and ). In contrast, ET patients with CALR frameshift mutations were older than those who carried wild-type CALR alleles (p = 0.023). Additionally, patients with CALR mutation exhibited lower hemoglobin (p = 0.015) and hematocrit levels (p = 0.008), leukocyte count (p = 0.047), and platelet count (p = 0.041) when compared with CALR-negative patients ( and ). Furthermore, ET patients with CALR mutations had lower hemoglobin (p = 0.013, hematocrit (p = 0.007), lower leukocyte count (p = 0.045), and lower platelet count (p = 0.041) than patients with JAK2 V617F mutations. This finding further supported that CALR mutation is associated with less aggressive disease phenotypes and contributes to a good prognosis of ET patients.
Figure 1. The distribution of JAK2 V617F, MPL W515L/K, and CALR exon 9 frameshift mutations in 58 ET patients.
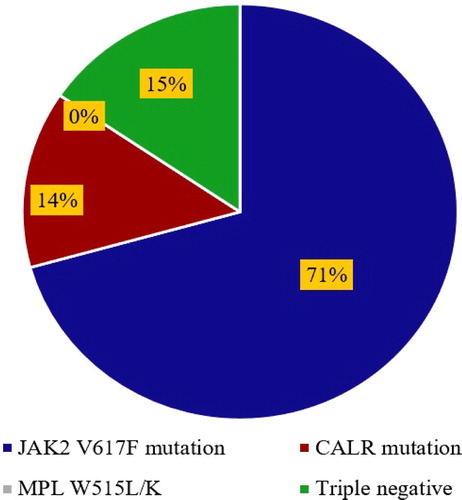
Table 1. JAK2 V617F mutation status and patient characteristics in ET.
Table 2. CALR exon 9 frameshift mutation and patient characteristics in ET.
CALR mutations as a biomarker for the diagnosis of JAK2-negative MPN
We further analyzed the distributions of JAK2 V617F and CALR mutations in the studied population. JAK2 V617F was frequently identified in ET patients (71%), whereas frameshift mutations of CALR exon 9 were identified in 14% of patients (8/58) . Furthermore, no co-existence mutation of JAK2 V617F and CALR frameshift mutations was observed in this study. Thus, 47% (8/17) of CALR exon 9 frameshift mutations were observed in JAK2 V617F-negative ET patients. There were two common types of CALR mutations detected in this report: six patients with CALR-type 1 (p.L367fs*46) () and two patients with CALR-type 2 (p.K385fs*47) (). The data further demonstrated the usefulness of CALR mutations analysis for the classification of JAK2-negative MPN. Additionally, we demonstrated that CALR exon 9 mutations were exclusively positive in ET patients with JAK2 V617F-negative and further confirmed that CALR exon 9 frameshift mutations could be used as a biomarker for the classification of JAK2-negative MPN with BCR-ABL1 negative.
Discussion
During the past decade, several genetic aberrations in genes involved in the initiation of hematological malignancies including MPNs have been discovered and well-characterized. Those are including the mutations on JAK2 (JAK2 V617F and JAK2 exon 12), MPL W515L/K, and more recently, CALR frameshift mutations. Despite BCR-ABL1- positive CML, JAK2 mutations (JAK2 V617F and JAK2 exon 12) are predominantly identified in the main entity of MPN including PV, ET, and PMF. In this study, we analyzed both JAK2 V617F and CALR exon 9 frameshift mutations in a total of 58 newly diagnosed ET patients. Similar to previous reports, we further confirmed that JAK2 V617F is exclusively mutated in nearly half of patients with ET. Moreover, there were no statistically significant differences in several parameters including age, sex, hemoglobin, hematocrit, and platelet count in ET patients with wild-type or JAK2 V617F mutation. These data were concordant with previous report by Sultan and Irfan [Citation19]. Additionally, a slightly increase in total leukocyte counts (p = 0.141) was observed in ET patients who carry the JAK2 V617F mutation. This data suggests the critical function of JAK2 in the progression of the disease. JAK2 is involved in several cellular signaling processes including proliferation, differentiation, survival, and stem cell self-renewal. It is evident that hematopoietic cells with mutated JAK2 are hypersensitive to several cytokines such as thrombopoietin, erythropoietin, stem cell factors, and granulocyte colony-stimulating factors. This could explain the predominant rise in several blood parameters (overproduction of blood compartments) in common MPN patients with JAK2 mutation [Citation3,Citation5,Citation20]. Consequently, laboratory analysis of JAK2 mutations is very important for disease classification, prognosis, and monitoring during treatment.
At present, several PCR-based techniques have been validated and routinely used for both qualitative and quantitative analysis of JAK2 V617F, MPL W515L/K, and CALR. While the simple qualitative method AS-PCR for JAK2 V617F is frequently used in several labs worldwide, the assay has low sensitivity to detect the tiny amount of mutation load (allele burden) as found in treated/monitored specimens. In parallel with this work, our group established the quantitative pyrosequencing technique specially to detect both JAK2 V617F and MPL W515L/K allele burden in PV and we could find that the sensitivity of pyrosequencing technique to detect JAK2 V617F was 5% of mutant in wild-type DNA (r2 = 0.9959) [Citation18]. Additionally, there was not difference in both AS-PCR and pyrosequencing technique to detect JAK2 V617F in newly diagnosed samples. Although the quantification of JAK2 allele burden is beneficial only for patients who receive stem cell transplantation, the assay is essentially used in clinical trial treatment with promising JAK2 inhibitors [Citation21]. Furthermore, in ET patients with frequently exhibiting low level of JAK2 V617F, more sensitive method is very necessary [Citation22]. Recently, several studies have elaborately reported the application of very high performance deep-sequencing technique to detect a tiny amount of aberrant cells in normal population [Citation11,Citation23,Citation24]. While deep-sequencing could detect several mutations simultaneously and show many advantages, the technique is now still very expensive and challenging for our resource-limited laboratory.
Besides demonstrating JAK2 V617F acquired mutation, Klampfl et al. [Citation10] and Nangalia et al. [Citation11] demonstrated that the exon 9 of CALR coding sequence is exclusively mutated in approximately 70% of MPN patients who have negative results for JAK2 mutations. Interestingly, it was evident that CALR mutations are not present in PV, suggesting the utility of CALR mutations analysis for molecular classification of MPN [Citation7,Citation10,Citation25–27]. Similar to previous studies () [Citation17,Citation25,Citation28], we could detect 14% (8/58) of ET patients harboring CALR exon 9 frameshift mutations. Additionally, the incidence was increased to 47% (8/17) in ET with JAK2 negative group. This finding further highlighted the important use of CALR mutations as the biomarker for the classification of Ph-negative MPN.
Table 3. The distribution of CALR exon 9 frameshift mutations in ET from different publications.
We compared several hematological parameters including leukocyte count, hemoglobin and hematocrit levels, and platelet count in ET patients with or without CALR mutations. Similar to previous reports, ET patients with CALR mutations exhibited lower leukocyte count, lower hemoglobin and hematocrit levels when compared with patients without CALR mutation. However, in this study, we observed that patients with CALR mutations have slightly lower platelet counts than patients without CALR mutation. This difference could be explained by the limited sample scale. Moreover, it was evident that ET patients with CALR-type 1 mutation have lower platelet count than patients with CALR-type 2 mutation [Citation14]. This finding further supported that CALR is functionally involved in megakaryocyte development and various types of CALR mutations may contribute to different disease phenotypes in MPN.
In summary, we reported the frequency of JAK2 V617F and CALR exon 9 frameshift mutations in Thai patients with ET. JAK2 V617F was commonly identified in our study population. Additionally, CALR exon 9 mutations were frequently detected in ET patients without JAK2 V617F. The clinical and hematological phenotypes of individual ET patient were associated with JAK2 and CALR mutation status. The integration of laboratory testings for JAK2, CALR, and MPL mutations is necessary to improve the diagnosis and classification of BCR-ABL1-negative MPN.
Acknowledgments
We thank Dr Suporn Chuncharunee and staffs at Division of Hematology, Department of Medicine, Faculty of Medicine Ramathibodi Hospital, Mahidol University for their valuable help especially in obtaining samples and clinical data. The authors thank Ramathibodi Cancer Center, Ramathibodi Hospital, Mahidol University for the support of reagents and chemicals.
Disclosure statement
No potential conflict of interest was reported by the authors.
Notes on contributors
N. Limsuwanachot is a senior scientist in Molecular Oncology group, pursuing research in myeloproliferative neoplasm, biomarkers of leukemia, and molecular pathology.
B. Rerkamnuaychoke is a head of Human Genetic Laboratory, pursuing research in molecular oncology and forensic genetics.
S. Chuncharunee is a senior doctor, pursuing research in hematological malignancy and blood disorders.
T. Pauwilai is a senior doctor, pursuing research in hematological malignancy and myeloma.
R. Singdong is a lecturer at the Department of Medical Technology, pursuing research in myeloproliferative neoplasm.
P. Rujirachaivej is a medical technologist, pursuing research in myelodysplastic syndrome and molecular pathology.
T. Chareonsirisuthigul is a medical doctor, pursuing research in genetic diseases and molecular pathology.
T. Siriboonpiputtana is a senior lecturer and leader of Molecular Oncology group, pursuing research in biomarkers of leukemia and cancer stem cell.
Additional information
Funding
References
- Vardiman JW, Thiele J, Arber DA, et al. The 2008 revision of the World Health Organization (WHO) classification of myeloid neoplasms and acute leukemia: rationale and important changes. Blood. 2009;114:937–951. doi: 10.1182/blood-2009-03-209262
- Baxter EJ, Scott LM, Campbell PJ, et al. Acquired mutation of the tyrosine kinase JAK2 in human myeloproliferative disorders. Lancet. 2005;365:1054–1061. doi: 10.1016/S0140-6736(05)74230-6
- Levine RL, Wadleigh M, Cools J, et al. Activating mutation in the tyrosine kinase JAK2 in polycythemia vera, essential thrombocythemia, and myeloid metaplasia with myelofibrosis. Cancer Cell. 2005;7:387–397. doi: 10.1016/j.ccr.2005.03.023
- Arber DA, Orazi A, Hasserjian R, et al. The 2016 revision to the World Health Organization classification of myeloid neoplasms and acute leukemia. Blood. 2016;127:2391–2405. doi: 10.1182/blood-2016-03-643544
- James C, Ugo V, Le Couédic JP, et al. A unique clonal JAK2 mutation leading to constitutive signalling causes polycythaemia vera. Nature. 2005;434:1144–1148. doi: 10.1038/nature03546
- Li N, Yao QM, Gale RP, et al. Frequency and allele burden of CALR mutations in Chinese with essential thrombocythemia and primary myelofibrosis without JAK2(V617F) or MPL mutations. Leuk Res. 2015;39:510–514. doi: 10.1016/j.leukres.2015.02.006
- Lin Y, Lui E, Sun Q, et al. The prevalence of JAK2, MPL, and CALR mutations in Chinese patients with BCR-ABL1-negative myeloproliferative neoplasms. Am J Clin Pathol. 2015;144:165–171. doi: 10.1309/AJCPALP51XDIXDDV
- Pikman Y, Lee BH, Mercher T, et al. MPLW515L is a novel somatic activating mutation in myelofibrosis with myeloid metaplasia. PLoS Med. 2006;3:e270. doi: 10.1371/journal.pmed.0030270
- Pardanani AD, Levine RL, Lasho T, et al. MPL515 mutations in myeloproliferative and other myeloid disorders: a study of 1182 patients. Blood. 2006;108:3472–3476. doi: 10.1182/blood-2006-04-018879
- Klampfl T, Gisslinger H, Harutyunyan AS, et al. Somatic mutations of calreticulin in myeloproliferative neoplasms. N Engl J Med. 2013;369:2379–2390. doi: 10.1056/NEJMoa1311347
- Nangalia J, Massie CE, Baxter EJ, et al. Somatic CALR mutations in myeloproliferative neoplasms with nonmutated JAK2. N Engl J Med. 2013;369:2391–2405. doi: 10.1056/NEJMoa1312542
- Wang WA, Groenendyk J, Michalak M. Calreticulin signaling in health and disease. Int J Biochem Cell Biol. 2012;44:842–846. doi: 10.1016/j.biocel.2012.02.009
- Rumi E, Pietra D, Ferretti V, et al. JAK2 or CALR mutation status defines subtypes of essential thrombocythemia with substantially different clinical course and outcomes. Blood. 2014;123:1544–1551. doi: 10.1182/blood-2013-11-539098
- Tefferi A, Lasho TL, Finke C, et al. Type 1 vs type 2 calreticulin mutations in primary myelofibrosis: differences in phenotype and prognostic impact. Leukemia. 2014;28:1568–1570. doi: 10.1038/leu.2014.83
- Rumi E, Pietra D, Pascutto C, et al. Clinical effect of driver mutations of JAK2, CALR, or MPL in primary myelofibrosis. Blood. 2014;124:1062–1069. doi: 10.1182/blood-2014-05-578435
- Cazzola M, Kralovics R. From Janus kinase 2 to calreticulin: the clinically relevant genomic landscape of myeloproliferative neoplasms. Blood. 2014;123:3714–3719. doi: 10.1182/blood-2014-03-530865
- Rotunno G, Mannarelli C, Guglielmelli P, et al. Impact of calreticulin mutations on clinical and hematological phenotype and outcome in essential thrombocythemia. Blood. 2014;123:1552–1555. doi: 10.1182/blood-2013-11-538983
- Singdong R, Siriboonpiputtana T, Chareonsirisuthigul T, et al. Characterization and prognosis significance of JAK2 (V617F), MPL, and CALR mutations in Philadelphia-negative myeloproliferative neoplasms. Asian Pac J Cancer Prev. 2016;17:4647–4653.
- Sultan S, Irfan SM. Acquired JAK-2 V617F Mutational analysis in Pakistani patients with essential thrombocythemia. Asian Pac J Cancer Prev. 2015;16:7327–7330. doi: 10.7314/APJCP.2015.16.16.7327
- Rampal R, Al-Shahrour F, Abdel-Wahab O, et al. Integrated genomic analysis illustrates the central role of JAK-STAT pathway activation in myeloproliferative neoplasm pathogenesis. Blood. 2014;123:e123–e133. doi: 10.1182/blood-2014-02-554634
- Azzato EM, Bagg A. Molecular genetic evaluation of myeloproliferative neoplasms. Int J Lab Hematol. 2015;37(Suppl 1):61–71. doi: 10.1111/ijlh.12353
- Park SH, Chi HS, Cho YU, et al. The allele burden of JAK2 V617F can aid in differential diagnosis of Philadelphia chromosome-negative myeloproliferative neoplasm. Blood Res. 2013;48:128–132. doi: 10.5045/br.2013.48.2.128
- Cabagnols X, Favale F, Pasquier F, et al. Presence of atypical thrombopoietin receptor (MPL) mutations in triple-negative essential thrombocythemia patients. Blood. 2016;127:333–342. doi: 10.1182/blood-2015-07-661983
- Milosevic Feenstra JD, Nivarthi H, Gisslinger H, et al. Whole-exome sequencing identifies novel MPL and JAK2 mutations in triple-negative myeloproliferative neoplasms. Blood. 2016;127:325–332. doi: 10.1182/blood-2015-07-661835
- Kim SY, Im K, Park SN, et al. CALR, JAK2, and MPL mutation profiles in patients with four different subtypes of myeloproliferative neoplasms: primary myelofibrosis, essential thrombocythemia, polycythemia vera, and myeloproliferative neoplasm, unclassifiable. Am J Clin Pathol. 2015;143:635–644. doi: 10.1309/AJCPUAAC16LIWZMM
- Gardner JA, Peterson JD, Turner SA, et al. Detection of CALR mutation in clonal and nonclonal hematologic diseases using fragment analysis and next-generation sequencing. Am J Clin Pathol. 2016;146:448–455. doi: 10.1093/ajcp/aqw129
- Clinton A, McMullin MF. The Calreticulin gene and myeloproliferative neoplasms. J Clin Pathol. 2016;69:841–845. doi: 10.1136/jclinpath-2016-203899
- Wojtaszewska M, Iwola M, Lewandowski K. Frequency and molecular characteristics of calreticulin gene (CALR) mutations in patients with JAK2 -negative myeloproliferative neoplasms. Acta Haematol. 2015;133:193–198. doi: 10.1159/000366263
- Nunes DP, Lima LT, Chauffaille Mde L, et al. CALR mutations screening in wild type JAK2(V617F) and MPL(W515 K/L) Brazilian myeloproliferative neoplasm patients. Blood Cells Mol Dis. 2015;55:236–240. doi: 10.1016/j.bcmd.2015.07.005
- Labastida-Mercado N, Galindo-Becerra S, Garcés-Eisele J, et al. The mutation profile of JAK2, MPL and CALR in Mexican patients with Philadelphia chromosome-negative myeloproliferative neoplasms. Hematol Oncol Stem Cell Ther. 2015;8:16–21. doi: 10.1016/j.hemonc.2014.12.002