ABSTRACT
Objectives: To analyze the kinetics of T-cell subsets and thymic function reconstitution after allogeneic hematopoietic stem cell transplantation (AHSCT); to determine whether sjTREC (signal joint TCR rearrangement excision circle) and CD31-positive recent thymic emigrant (CD31 + RTE) are correlated with acute graft versus host disease (aGVHD) or CMV (cytomegalovirus) viremia after AHSCT.
Methods: Forty-nine patients who underwent AHSCT in our institution were prospectively enrolled. Periphery blood samples were collected before conditioning and at 1, 2, 3 months after AHSCT. T-cell subsets were analyzed with flow cytometry. Genomic DNA was purified from peripheral blood mononuclear cells (PBMCs), and sjTREC was quantified by real-time PCR. Impact of sjTREC and CD31 + RTE on aGVHD and CMV viremia was evaluated by univariate and multivariate Cox regression analyses.
Results: The analyzed T-cell subsets and sjTREC of patients before AHSCT were all significantly lower than those of healthy donors (p < 0.05). sjTREC and CD31 + RTE were remarkably decreased in 3 months after AHSCT (p < 0.05). Patients with lower pre-transplantation sjTREC and CD31 + RTE level had higher incidence of CMV viremia after AHSCT (p < 0.05). sjTREC/106 PBMCs was negatively correlated with aGVHD (p = 0.024).
Conclusion: Thymic function was impaired before transplantation, and was consistently decreased in 3 months after AHSCT. Patients who had lower pre-transplantation sjTREC level were at high risk of aGVHD and CMV viremia after AHSCT, low pre-transplantation CD31 + RTE was correlated with CMV viremia after AHSCT.
Introduction
Allogeneic hematopoietic stem cell transplantation (AHSCT) has been widely used in the treatment of malignant and nonmalignant disorders. However, the efficacy of HSCT is still limited by the subsequent complications including graft versus host disease and opportunistic infections [Citation1]. Previous studies have indicated that T-cell reconstitution after HSCT is correlated with acute graft versus host disease (aGVHD), CMV (cytomegalovirus) infection [Citation2–4].
Peripheral T-cell reconstitution after AHSCT is accomplished through two mechanisms [Citation5,Citation6]: thymus-independent and thymus-dependent pathways. In the thymic-independent pathway, graft-derived mature donor T-cells and host T-cells having survived the conditioning regimen were triggered by antigen or cytokine, leading to cell expansion and skewed T-cell receptor (TCR) cell repertoire. On the other hand, the thymic-dependent pathway depends on the recruitment of donor-derived naïve T-cells into the thymus. It is slower and generates a more diverse TCR repertoire. Accordingly, thymic function is essential for the reconstitution of a fully functional TCR repertoire.
There are several methods that have been used as markers of thymic function. Among them, the sjTREC (signal joint TCR rearrangement excision circle) has been widely used to quantify the thymic output of de novo T cells [Citation7–9]. sjTRECs are formed as extrachromosomal circular DNA by-product after the deletion of TCR-d locus [Citation10,Citation11]; they are not duplicated during cell proliferation and diluted out during cell division, therefore, can be used as an indirect measurement of thymic output [Citation12]. Additionally, CD4 + CD45RA + CD31+T-cells are also used as a marker of recent thymic emigrant (CD31 + RTE), and contain high concentration of sjTREC [Citation13,Citation14]. sjTREC and CD31 + RTE were analyzed in many recent studies to evaluate thymic function in patients with different disorders. Increasing amount of clinical researches indicate that monitoring sjTREC and CD31 + RTEC is of great value in terms of predicting and diagnosing clinical events in AHSCT [Citation2,Citation15–20].
CMV infection and aGVHD are major life-threatening complications after AHSCT; hence, studies which focus on screening for risk factors of aGVHD and CMV infection are of important value in clinical practice. Clave et al. [Citation16] reported that sjTREC was of prognostic value in AHSCT, indicating pre-transplantation thymic function is inversely correlated with GVHD and CMV infection; however, in that study, they only included HLA-identical sibling HSCT patients.
In the present study, we prospectively measured thymic function before and in 100 days after AHSCT in 49 patients with sjTREC and CD31 + RTE to determine whether they are associated with aGVHD or CMV viremia and simultaneously investigate the kinetics of T-cell reconstitution after HSCT.
Methods
Patients
The study included 49 patients who underwent AHSCT in Institute of Hematology and Blood Diseases Hospital, Chinese Academy of Medical Sciences, from October 2014 to March 2015. Informed consents were obtained from all patients according to the Declaration of Helsinki. Clinical characteristics of these patients are summarized in . The grafts were obtained from HLA-matched-related, HLA-matched-unrelated donors or HLA-haploidentical donors. Blood samples were obtained before conditioning regimen and at 1, 2, 3 months after transplantation and were immediately used for analysis. Diagnosis of aGVHD was based on Seattle criteria [Citation21,Citation22]. CMV viremia was identified by plasma CMV real-time polymerase chain reaction (RT-PCR) assay.
Table 1. Patient characteristics.
Flow cytometry analysis
Peripheral blood mononuclear cells (PBMCs) were isolated by density gradient centrifugation using Ficoll-Hypaque (GE Healthcare Bio-Sciences Corp., Piscataway, NJ, U.S.A.) from fresh heparinized patient blood samples. Cells were stained for 20 min at 4°C in dark with the following fluorochrome-conjugated anti-human monoclonal antibodies and isotype antibodies (Biolegend, San Diego, CA, U.S.A.): CD3 APC/CY7,CD4 FITC (Clone: A161A1), CD8 PE-CY7 (Clone: SK1), CD45RA Percp-CY5 (Clone: HI100), CD62L APC (Clone: DREG-56), CD31 PE (Clone: WM59); PBMCs were analyzed by flow cytometry using Canto2 flow cytometer (BD Biosciences) supported by the FlowJo software (Tree Star Inc., Ashland, OR, U.S.A.). Absolute blood counts of lymphocyte subsets were then calculated by multiplying T-cell subset percentage with ALC, where ALC indicates the absolute lymphocyte count.
sjTREC quantification
Genomic DNA was purified from PBMCs using QIAamp DNA Blood Mini Kit (QIAGEN GmbH, Hilden, Germany) according to the manufacturer’s instructions. sjTREC was quantified by RT-PCR [Citation23]. Briefly, we prepared a plasmid containing one fragment of sjTREC and TR alpha constant gene (TRAC) DNA in each plasmid. And then we used the following primer and probes to detect sjTREC and TRAC: sjTREC forward primer (5′-CACATC CCT TTCAACCATGCT-3′), reverse primer (5′-GCCAGCTGCAGGGTTTAGG-3′) and the probe (5′-FAM-ACA CCT CTG GTT TTT GTA AAG GTG CCC ACT TAMRA-3′) [Citation24]. TRAC forward primer (5′-TGGCCTAACCCTGATCCTCTT-3′), reverse primer (5′-GGA TTTAGAGTCTCTCAGCTGGTACAC-3′) and the probe (5′-FAM-TCC CACAGATATCCAGAACCCTGACCCTAMRA-3′) [Citation23]. PCR reactions were performed in PCR plate, 384-well, standard (Applied Biosystems, Foster City, CA, U.S.A.) in a final volume of 10 μl consisting of 1 μl (100–500 ng) genomic DNA, 5 μl of 2×TaqMan Universal PCR master mix containing AmpErase UNG (Applied Biosystems, Foster City, CA, U.S.A.), primers and probes for sjTREC and TRAC at a final concentration of 400 and 200 nM, respectively. sjTREC and TRAC copy number were determined by a unique standard curve which was obtained by the amplification of serial dilutions of the plasmid (106, 105, 104, 103, 102, 10) [Citation23]. TRAC served as a control for the quality and quantity of genomic DNA in the sample.
Statistical analysis
Comparison between patients and healthy donors was done with the Mann–Whitney rank-sum test. Changes of T-cell subsets and sjTREC at different time points were longitudinally evaluated by the SPSS Mixed Model. Receiver operating characteristic (ROC) curves were performed to determine the cut-off values of sjTREC and CD31 + RTE: values were selected to maximize specificity while maintaining a good sensitivity. Probability of aGVHD and CMV viremia was analyzed and plotted by the cumulative incidence estimates and compared with Gray’s test. Cox proportional-hazards multivariate regression was performed, including recipient age, donor types in all multivariate models. Hazards ratios (HR) and 95% confidence intervals (CI) were shown for this model. The effect of CD31 + RTE levels on CMV viremia was not analyzed in Cox proportional-hazards multivariate regression model, because of the small sample size. Two-sided p value of 0.05 was regarded as significant. The statistics was performed by the software SPSS17.0 (IBM Corporation, Armonk, NY, U.S.A.) and R2.15.0.
Results
Characteristics of patients
We prospectively enrolled 49 patients who underwent AHSCT in our hematopoietic stem cell transplantation center. The median age of the patients was 35 years (range, 5–56). Bone marrow and mobilized peripheral blood was used as the stem cell source in 2 patients and 45 patients, respectively, and there were also two patients received stem cells from both bone marrow and mobilized peripheral blood. The median number of CD34+ cells infused was 2.4 × 106/kg (range, 0.44–8.3). Among those patients, 29 patients underwent matched sibling AHSCT, 9 patients underwent matched-unrelated AHSCT and 11 patients underwent HLA-haploidentical AHSCT. Blood samples were collected to monitor CMV viremia by RT-PCR every week till 100 days after AHSCT and 42.8% (21/49) patients were diagnosed with aGVHD. 30.6% (15/49) patients developed CMV viremia. All the patients received myeloablative conditioning, consisting of TBI-based regimen (16/49) and chemo-based regimen (33/49). After a median follow-up of 351 days (range, 60–592 days), 38 patients were still alive.
Thymic function recovery
sjTREC copies were analyzed before conditioning and at 1, 3 months in patients after AHSCT and also in 33 healthy donors. We simultaneously measured T-cell reconstitution by monitoring T-cell subsets with flow cytometry. T-cell subsets were significantly decreased before AHSCT, compared with healthy control (). CD3 + T cells and CD8 + T cells were restored to pre-transplantation level as early as 1-month post AHSCT, which may be attributed to antigen-driven expansion of graft-derived T-cells through thymus-independent pathway ((A,B)). Reconstitution of CD4 + T cells was slower than CD3 + T cells and CD8 + T cells ((C)). However, sjTREC copies and CD31 + RTE were remarkably decreased after transplantation and were kept at very low levels in 3 months after AHSCT ((D–F)). Both pre-transplantation CD31 + RTE and sjTREC copies were significantly lower than those of healthy control (p = 0.008, p < 0.001, respectively). As would be expected, absolute numbers of naive (CD4 + CD45RA + CD62L + T-cell) T-cells were also low in 3 months post AHSCT, showing a similar profile of recovery kinetics of sjTREC and CD31 + RTE ((G)). sjTREC was closely related with age (r = −0.435, p < 0.001, Figure S1A), and CD31 + RTE (r = 0.744, p < 0.001, Figure S1B).
Figure 1. Kinetics of T-cell subsets and sjTREC after AHSCT. In each panel, results are expressed as absolute number of cells per μl periphery blood for T-cell subsets, copies per 106 PBMCs and copies per ml periphery blood for sjTREC. Results of healthy donors are also included in each panel. Different panels show the distribution of a distinct cell subset at different time points: before AHSCT, and at 1 month, 2 months, 3 months. (A) CD3+T cells. (B) CD3+CD8+T cells. (C) CD3+CD4+T cells. (D) CD4+CD45RA+CD31+T cells. (E) sjTREC per ml blood. (F) sjTREC per 106 PBMCs. (G) CD4+CD45RA+CD62L+T cells. Each box shows the median, quartiles, and extreme values.

Pre-transplantation thymic function predicts aGVHD after AHSCT
Acute GVHD is a common complication after AHSCT and also a major cause of morbidity and mortality after AHSCT [Citation25]. In 49 patients, 42.8% had acute GVHD (21/49). Patients were separated into two groups: sjTREC high and sjTREC low group, using the cut-off value from the ROC curve (Figure S2A and S2B). The cut-off value for sjTREC (per 106 PBMCs) and sjTREC (per ml blood) was 300/106 PBMCs and 370 per ml blood, respectively. Using death as a competing factor, low sjTREC level (per 106 PBMCs) after AHSCT was associated with higher incidence of aGVHD (p = 0.024, (A)). When we analyzed the correlation between sjTREC level (per ml blood) and aGVHD, we also observed the same trend, although it was not statistically significant (p = 0.053, (B)).
Figure 2. sjTREC level was of predictive value for aGVHD in patients after AHSCT. Cumulative incidence of aGVHD in patients who underwent AHSCT (n = 40). Gray line indicates high sjTREC level, black dashed line indicates low sjTREC level. sjTREC levels are expressed in sjTREC per 106 PBMCs (A) and sjTREC per ml blood (B).
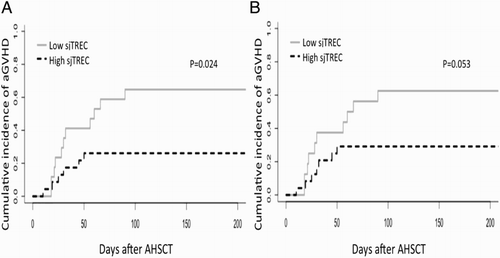
In the COX multivariate model (), which included recipient age, sjTREC levels, and donor types. sjTREC (per 106 PBMCs) had a significant impact on aGVHD incidence, independent of other two factors (HR = 3.352 95% CI1.214–9.258, p = 0.02). As expected, HLA-mismatched donor was an independent risk factor of aGVHD (HR = 0.353, 95% CI 0.128–0.984, p = 0.046).
Table 2. Cox multivariate analysis.
aGVHD was not associated with pre-transplantation absolute CD31 + RTE cell counts or CD31 + RTE percentage in CD4 + T cells (data not shown).
Pre-transplantation thymic function predicts CMV viremia after AHSCT
CMV viremia is also a major cause of morbidity and mortality after AHSCT. Among the enrolled patients, we recorded 16 patients who developed CMV viremia. A higher incidence of CMV viremia was found in patients with a low pre-transplantation sjTREC level (per 106 PBMCs), as compared with those with high sjTREC level (P = 0.004, (A)). Similarly, patients with low sjTREC level (per ml blood) also had a higher incidence of CMV viremia (p = 0.021, (B)). The cut-off value of sjTREC (per 106 PBMCs) and sjTREC (per ml blood) was determined by ROC curve (Figure S3A and S3B); and they were 256 per 106 PBMCs and 370 per ml blood, respectively. We also analyzed if CD31 + RTE has predictive value in CMV viremia after AHSCT, both lower CD31 + RTE percentage in CD4 + T cells and absolute CD31 + RTE cell number could predict CMV viremia (p = 0.022 and 0.002, respectively, (C,D)). The cut-off values were 32% and 110 μl blood, respectively (Figure S3C and S3D).
Figure 3. sjTREC level and CD31+RTE were of predictive value for CMV viremia in patients after AHSCT. Cumulative incidence of CMV viremia in patients who underwent AHSCT. Gray line indicates high sjTREC level, black dashed line indicates low sjTREC level. sjTREC are expressed in sjTREC copies per 106 PBMCs (n = 40) (A) and sjTREC copies per ml blood (n = 40) (B) CD31+RTE are expressed in percentage in CD4+T cells (n = 32) (C) and μl blood (n = 31) (D).
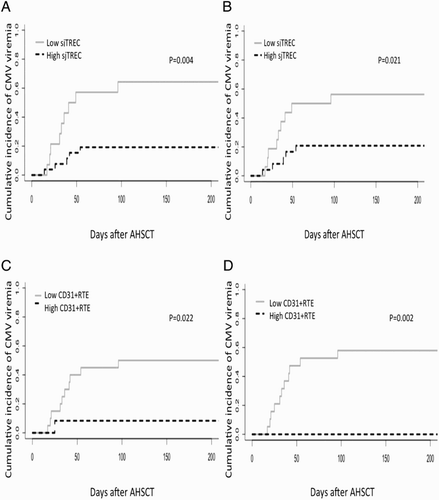
Using the same parameters as for aGVHD, multivariate analysis was also done for CMV viremia (). sjTREC (per 106 PBMCs) was still related with CMV viremia (HR = 9.949 95% CI 2.281–43.386, p = 0.002). In consistent with sjTREC (per 106 PBMCs), lower sjTREC (per ml) was also an independent risk factor for CMV viremia (HR = 4.802 95% CI1.173–19.667, p = 0.029). Interestingly, HLA-mismatched donor was also at higher risk of CMV viremia (p < 0.05).
Discussion
In the current study, we monitored kinetics of sjTREC and T-cell subsets before and early after AHSCT in 49 patients who underwent AHSCT in our institution. We found that both sjTREC and CD31 + RTE cells were remarkably lower in patients before AHSCT, compared with healthy donors, and were significantly depressed till 100 days after AHSCT. sjTREC value had predictive value for aGVHD after AHSCT. Moreover, sjTREC value and CD31 + RTE cells were of predictive value for CMV viremia after AHSCT.
aGVHD is a major cause of mortality and morbidity following AHSCT and therefore limits AHSCT success [Citation25]. There are many predictors of aGVHD, which include HLA differences between donor and recipient, recipient age, gender mismatch between donor and recipient, mHA in otherwise identical AHSCT, donor age, source and dose of stem cells, intensity of conditioning, and GVHD prophylaxis [Citation26]. In this study, we want to investigate whether pre-transplantation sjTREC and CD31 + RTE are of predictive value for aGVHD and CMV viremia after AHSCT. Our data showed lower pre-transplantation sjTREC level (per 106 PBMCs) was associated with higher aGVHD incidence after AHSCT. Similarly, the same trend was found when we analyzed sjTREC (per ml blood), despite it was not statistically significant. Previous studies have associated sjTREC levels with aGVHD, but there is still no clear conclusion. Clave et al. [Citation16] showed that there was a significant association between low pre-transplantation sjTREC counts and a higher incidence of grade II-IV aGVHD. However, when they included other parameters (age of 25 years, donor/recipient CMV serology, ABO incompatibility, sex mismatch, and disease risk) in the COX multiparameter analysis, it was not significantly important. The disparity of this study with our results may be explained by the difference of donor types between these two studies.
The probable mechanism may involve impaired negative selection [Citation27]. It has been long known thymic epithelial cells (TECs) play an essential role in negative selection in thymus to get rid of autoimmune T-cells [Citation28]. The negative selection relies on the ectopic expression of tissue-restricted peripheral self-antigens (TRA) in mature medullary thymic epithelial cells, which may be damaged in patients with impaired thymic function, thus, lead to compromised negative selection and GVHD [Citation27,Citation29].
Moreover, another mechanism relevant to thymic function could implicate donor-derived CD4 CD25high regulatory T (Treg)-cell reconstitution [Citation30,Citation31]. Those cells were also selected in the thymus, a competent thymic is needed for regulatory T-cells recovery in AHSCT patients [Citation32]. Previous studies showed the important role of Treg cells in the prevention and treatment of GVHD in murine models and humans [Citation33–36]. Impaired thymic function due to pre-transplantation chemotherapy, conditioning regimen or aging may lead to failed negative selection and impaired regulatory T-cell recovery, which may consequently increase the risk of GVHD after AHSCT.
CMV infection is also a life-threatening complication after AHSCT. We analyzed the effect of pre-transplantation thymic function on CMV viremia, our data indicated that both sjTREC (per 106 PBMCs) and sjTREC (per ml blood) were correlated with CMV viremia, which confirmed the result of the previous study [Citation16]. Additionally, pre-transplantation CD31 + RTE level also had the same impact on CMV viremia as sjTREC. As reported by many previous studies, CD31 + RTE and sjTREC are closely related with each other, they may both act as markers of thymic function [Citation13]. The possible explanation may be that pre-transplantation thymic function may predict residual thymic function after AHSCT, which was shown in Chen’s study [Citation15], and residual thymic function may be helpful to early reconstitution of CMV-specific T-cells and thus protect patients from CMV reactivation.
In summary, we showed pre-transplantation CD31 + RTE and sjTREC were both related with CMV viremia after HSCT. sjTREC was of predictive value for aGVHD after AHSCT. sjTREC and CD31 + RTE may be useful in guiding individualized prophylaxis and pre-emptive therapies for aGVHD and CMV infection.
Supplementary_material.zip
Download Zip (1.1 MB)Disclosure statement
No potential conflict of interest was reported by the authors.
Notes on contributors
Xin Yang is an MD, PhD student, Peking Union Medical College and Chinese Academy of Medical Sciences.
Yuanxin Sun is an MD, PhD student, Shandong University.
Sudong Zhang is an MD, PhD student, Peking Union Medical College and Chinese Academy of Medical Sciences.
Hui Yang is an MD, PhD student, Shandong University.
Jialin Wei is an MD, PhD, associate chief physician, Hematopoietic Stem Cell Transplantation Center, Institute of Hematology and Blood Diseases Hospital, Peking Union Medical College and Chinese Academy of Medical Sciences.
Yi He is an MD, PhD, associate chief physician, Hematopoietic Stem Cell Transplantation Center, Institute of Hematology and Blood Diseases Hospital, Peking Union Medical College and Chinese Academy of Medical Sciences.
Donglin Yang is an MD, PhD, associate chief physician, Hematopoietic Stem Cell Transplantation Center, Institute of Hematology and Blood Diseases Hospital, Peking Union Medical College and Chinese Academy of Medical Sciences.
Erlie Jiang is an MD, PhD, associate chief physician, Hematopoietic Stem Cell Transplantation Center, Institute of Hematology and Blood Diseases Hospital, Peking Union Medical College and Chinese Academy of Medical Sciences.
Mingzhe Han is an MD, PhD, chief physician, director of Hematopoietic Stem Cell Transplantation Center, Institute of Hematology and Blood Diseases Hospital, Peking Union Medical College and Chinese Academy of Medical Sciences.
Xuemei Qin is an MD, PhD, associate chief physician, Department of Hematology, Qilu Hospital, Shandong University.
Sizhou Feng is an MD, PhD, chief physician, the vice director of Hematopoietic Stem Cell Transplantation Center, Institute of Hematology and Blood Diseases Hospital, Peking Union Medical College and Chinese Academy of Medical Sciences.
Additional information
Funding
References
- Singh AK, McGuirk JP. Allogeneic stem cell transplantation: a historical and scientific overview. Cancer Res. 2016 Nov 15;76(22):6445–6451.
- Brown JA, Stevenson K, Kim HT, et al. Clearance of CMV viremia and survival after double umbilical cord blood transplantation in adults depends on reconstitution of thymopoiesis. Blood. 2010;115:4111–4119. doi: 10.1182/blood-2009-09-244145
- Geddes M, Storek J. Immune reconstitution following hematopoietic stem-cell transplantation. Best Pract Res Clin Haematol. 2007 Jun;20(2):329–348. doi: 10.1016/j.beha.2006.09.009
- Lilleri D, Gerna G, Fornara C, et al. Prospective simultaneous quantification of human cytomegalovirus-specific CD4+ and CD8+ T-cell reconstitution in young recipients of allogeneic hematopoietic stem cell transplants. Blood. 2006 Aug 15;108(4):1406–1412. doi: 10.1182/blood-2005-11-012864
- Toubert A, Glauzy S, Douay C, et al. Thymus and immune reconstitution after allogeneic hematopoietic stem cell transplantation in humans: never say never again. Tissue Antigens. 2012 Feb;79(2):83–89. doi: 10.1111/j.1399-0039.2011.01820.x
- Hakim FT, Gress RE. Reconstitution of thymic function after stem cell transplantation in humans. Curr Opin Hematol. 2002 Nov;9(6):490–496. doi: 10.1097/00062752-200211000-00004
- Hochberg EP, Chillemi AC, Wu CJ, et al. Quantitation of T-cell neogenesis in vivo after allogeneic bone marrow transplantation in adults. Blood. 2001;98(4):1116–1121. doi: 10.1182/blood.V98.4.1116
- Dion ML, Sekaly RP, Cheynier R. Estimating thymic function through quantification of T-cell receptor excision circles. Methods Mol Biol. 2007;380:197–213. doi: 10.1007/978-1-59745-395-0_12
- Ringhoffer S, Rojewski M, Dohner H, et al. T-cell reconstitution after allogeneic stem cell transplantation: assessment by measurement of the sjTREC/TREC ratio and thymic naive T cells. Haematologica. 2013 Oct;98(10):1600–1608. doi: 10.3324/haematol.2012.072264
- de Villartay J-P, Hockett RD, Korsmeyer SJ, et al. Deletion of the human T-cell receptor δ-gene by a site-specific recombination. 1988.
- Takeshita S, Toda M, Yamagishi H. Excision products of the T cell receptor gene support a progressive rearrangement model of the alpha/delta locus. EMBO J. 1989 Nov;8(11):3261–3270.
- Poulin JF, Viswanathan MN, Harris JM, et al. Direct evidence for thymic function in adult humans. J Exp Med. 1999 Aug 16;190(4):479–486. doi: 10.1084/jem.190.4.479
- Kimmig S, Przybylski GK, Schmidt CA, et al. Two subsets of naive T helper cells with distinct T cell receptor excision circle content in human adult peripheral blood. J Exp Med. 2002;195(6):789–794. doi: 10.1084/jem.20011756
- Kohler S, Thiel A. Life after the thymus: CD31+ and CD31-human naive CD4+ T-cell subsets. Blood. 2009 Jan 22;113(4):769–774. doi: 10.1182/blood-2008-02-139154
- Chen X, Barfield R, Benaim E, et al. Prediction of T-cell reconstitution by assessment of T-cell receptor excision circle before allogeneic hematopoietic stem cell transplantation in pediatric patients. Blood. 2005 Jan 15;105(2):886–893. doi: 10.1182/blood-2004-04-1405
- Clave E, Rocha V, Talvensaari K, et al. Prognostic value of pretransplantation host thymic function in HLA-identical sibling hematopoietic stem cell transplantation. Blood. 2005 Mar 15;105(6):2608–2613. doi: 10.1182/blood-2004-04-1667
- Wils EJ, van der Holt B, Broers AE, et al. Insufficient recovery of thymopoiesis predicts for opportunistic infections in allogeneic hematopoietic stem cell transplant recipients. Haematologica. 2011 Dec;96(12):1846–1854. doi: 10.3324/haematol.2011.047696
- Sairafi D, Mattsson J, Uhlin M, et al. Thymic function after allogeneic stem cell transplantation is dependent on graft source and predictive of long term survival. Clin Immunol. 2012 Mar;142(3):343–350. doi: 10.1016/j.clim.2011.12.001
- Clave E, Lisini D, Douay C, et al. Thymic function recovery after unrelated donor cord blood or T-cell depleted HLA-haploidentical stem cell transplantation correlates with leukemia relapse. Front Immunol. 2013;4:54. doi: 10.3389/fimmu.2013.00054
- Uzunel M, Sairafi D, Remberger M, et al. T-cell receptor excision circle levels after allogeneic stem cell transplantation are predictive of relapse in patients with acute myeloid leukemia and myelodysplastic syndrome. Stem Cells Dev. 2014 Jul 15;23(14):1559–1567. doi: 10.1089/scd.2013.0588
- Deeg HJ, Storb R. Graft-versus-host disease: pathophysiological and clinical aspects. Annu Rev Med. 1984;35:11–24. doi: 10.1146/annurev.me.35.020184.000303
- Lee SJ, Vogelsang G, Flowers ME. Chronic graft-versus-host disease. Biol Blood Marrow Transplant. 2003 Apr;9(4):215–233. doi: 10.1053/bbmt.2003.50026
- Sottini A, Ghidini C, Zanotti C, et al. Simultaneous quantification of recent thymic T-cell and bone marrow B-cell emigrants in patients with primary immunodeficiency undergone to stem cell transplantation. Clin Immunol. 2010 Aug;136(2):217–227. doi: 10.1016/j.clim.2010.04.005
- Douek DC, McFarland RD, Keiser PH, et al. Changes in thymic function with age and during the treatment of HIV infection. Nature. 1998 Dec 17;396(6712):690–695. doi: 10.1038/25374
- Blazar BR, Murphy WJ, Abedi M. Advances in graft-versus-host disease biology and therapy. Nat Rev Immunol. 2012 May 11;12(6):443–458. doi: 10.1038/nri3212
- Ball LM, Egeler RM, Party EPW. Acute GvHD: pathogenesis and classification. Bone Marrow Transplant. 2008 Jun;41(Suppl 2):S58–S64. doi: 10.1038/bmt.2008.56
- Teshima T, Reddy P, Liu C, et al. Impaired thymic negative selection causes autoimmune graft-versus-host disease. Blood. 2003 Jul 15;102(2):429–435. doi: 10.1182/blood-2003-01-0266
- Peterson P, Org T, Rebane A. Transcriptional regulation by AIRE: molecular mechanisms of central tolerance. Nat Rev Immunol. 2008 Dec;8(12):948–957. doi: 10.1038/nri2450
- Dertschnig S, Hauri-Hohl MM, Vollmer M, et al. Impaired thymic expression of tissue-restricted antigens licenses the de novo generation of autoreactive CD4+ T cells in acute GVHD. Blood. 2015 Apr 23;125(17):2720–2723. doi: 10.1182/blood-2014-08-597245
- Reubsaet LL, de Pagter AP, van Baarle D, et al. Stem cell source-dependent reconstitution of FOXP3+ T cells after pediatric SCT and the association with allo-reactive disease. Bone Marrow Transplant. 2013 Apr;48(4):502–507. doi: 10.1038/bmt.2012.174
- Johnson BD, Konkol MC, Truitt RL. CD25+ immunoregulatory T-cells of donor origin suppress alloreactivity after BMT. Biol Blood Marrow Transplant. 2002;8(10):525–535. doi: 10.1053/bbmt.2002.v8.pm12434947
- Miura Y, Thoburn CJ, Bright EC, et al. Association of Foxp3 regulatory gene expression with graft-versus-host disease. Blood. 2004 Oct 1;104(7):2187–2193. doi: 10.1182/blood-2004-03-1040
- Cohen JL, Trenado A, Vasey D, et al. CD4+ CD25+ immunoregulatory T cells new therapeutics for graft-versus-host disease. J Exp Med. 2002;196(3):401–406. doi: 10.1084/jem.20020090
- Hoffmann P, Ermann J, Edinger M, et al. Donor-type CD4+ CD25+ regulatory T cells suppress lethal acute graft-versus-host disease after allogeneic bone marrow transplantation. J Exp Med. 2002;196(3):389–399. doi: 10.1084/jem.20020399
- Taylor PA, Lees CJ, Blazar BR. The infusion of ex vivo activated and expanded CD4+CD25+ immune regulatory cells inhibits graft-versus-host disease lethality. Blood. 2002;99(10):3493–3499. doi: 10.1182/blood.V99.10.3493
- Rezvani K, Mielke S, Ahmadzadeh M, et al. High donor FOXP3-positive regulatory T-cell (treg) content is associated with a low risk of GVHD following HLA-matched allogeneic SCT. Blood. 2006 Aug 15;108(4):1291–1297. doi: 10.1182/blood-2006-02-003996