ABSTRACT
Objectives: In the present study, we first confirmed the suppressive function of MSCs in allogeneic T cell proliferation and then examined the underlying mechanisms for MSCs’ immunomodulation and the role of the pro-inflammatory cytokine interferon (IFN)-γ.
Methods: Human MSCs were cultured in the presence or absence of IFN-γ. The expression level of prostaglandin E2 (PGE2), hepatocyte growth factor (HGF), transforming growth factor (TGF)-β1 and indoleamine 2,3-dioxygenase (IDO) by MSCs were measured. T lymphocytes were isolated from peripheral blood of healthy donors and then induced to proliferate under the stimulation of anti-human CD3 mAb and anti-human CD28 mAb. In the presence of MSCs, T cell proliferation was examined by BrdU incorporation. In addition, PGE2, HGF, TGF-β1, Kynurenine, recombinant human IFN-γ and anti-IFN-γ mAb were added and cell proliferation was examined.
Results: Compared to the controls (MSCs alone), MSCs cocultured with IFN-γ expressed significantly higher concentrations of PGE2, HGF and TGF-β1. The mRNA level of IDO was remarkably increased. Human bone marrow-derived MSCs alone notably suppressed T lymphocytes proliferation in vitro. Addition of exogenous IFN-γ did not ablate the immunosuppressive effects of MSCs. Addition of anti-IFN-γ mAb partially restored suppression of T cell proliferation by MSCs.
Conclusions: Human MSCs constitutively expressed immunosuppressive levels of PGE2, HGF and TGF-β1. The proinflammatory cytokine IFN-γ exhibited synergistic effects with MSCs on immunosuppression, possibly by up-regulating PGE2, HGF and TGF-β1 in MSCs and inducting MSCs expression of IDO, involved in tryptophan catabolism.
Introduction
Mesenchymal stem cells (MSCs) are nonhematopoietic, multipotent progenitor cells with the capacity of multipotential differentiation. They are able to differentiate into several mesenchymal lineages, such as bone, cartilage, adipose tissue, tendon, neural precursor cells, muscle and cardiomyocytes in certain microenvironments in vitro [Citation1]. MSCs are generally isolated based on their adherence to tissue-culture plates. They can be expanded ex vivo from a small amount of bone marrow aspirates and expansion does not cause apparent phenotypic changes or loss of function [Citation2]. Therefore, MSCs have emerged as a promising tool for clinical applications such as tissue engineering and cell and gene therapy.
Another intriguing feature of MSCs is their capacity to regulate immune responses while escaping immune recognition. MSCs have the ability to modulate functions of major immune cell populations including dendritic cells, T cells, B cells, and natural killer (NK) cells [Citation3]. Moreover, MSCs are considered to be non-immunogenic as they do not elicit allogeneic immune response [Citation4].
MSCs are not always immunosuppressive but have a distinct immunomodulatory profile determined by the local environment. MSCs are sensors of inflammation and are able to adopt a proinflammatory or anti-inflammatory phenotype by interfering with innate and adaptive immune responses both in vitro and in vivo [Citation5]. The immunomodulatory mechanisms of MSCs are not fully understood. However, several possible mechanisms have been proposed. In an inflammatory environment (with high levels of pro-inflammatory cytokines such as interferon (IFN)-γ, tumor necrosis factor-α and interleukin-1β), MSCs become activated and adopt an immune-suppressive phenotype by producing nitric oxide, indoleamine 2,3-dioxygenase (IDO), and other immunosuppressive cytokines and chemokines [Citation6]. MSCs can also function through modulating regulatory T lymphocytes (Treg) or direct suppression of T cells by inducting apoptosis [Citation7]. Furthermore, T cells are the major effectors mediating acute graft-versus-host disease (aGVHD) after allogeneic hematopoietic stem cell transplantation. MSCs have been shown to inhibit T-cell activation and proliferation triggered by cellular or nonspecific mitogen stimuli [Citation8]. MSCs generated in vitro have already been used in the treatment of severe aGVHD, especially in steroid-refractory cases, and have shown encouraging efficacy [Citation9–11].
In the present study, we examined the mechanisms by which MSCs exert immunomodulatory effects by assessing the role of several MSC-derived soluble factors and the tryptophan degrading enzyme (IDO). The role of the pro-inflammatory cytokine IFN-γ was also examined. The results showed that IFN-γ did not inhibit but enhanced the immunosuppressive capacity of MSCs, indicating synergistic effects of IFN-γ and MSCs on immunomodulation.
Materials and methods
Generation of human bone marrow MSCs
Bone marrow aspirates were collected from healthy volunteers after informed consent. Mononuclear cells were isolated using Ficoll-Hypaque solution and obtained with density-gradient centrifugation. Cells were resuspended in Dulbecco’s modified Eagle’s medium (DMEM) supplemented with 10% fetal bovine serum (FBS) (Invitrogen-Gibco, Beijing, China) and 1% penicillin/streptomycin (HyClone, Utah, U.S.A.) and cultured in 25-cm2 flasks (Corning, Durham, NC 27712, U.S.A.) at a concentration of 1 × 106/ml, at 37°C in a 5% CO2 atmosphere. After culture for 72 hours, nonadherent cells were removed and MSCs developed to an adherent fibroblast-like population. After reaching 70–80% confluency, the adherent MSCs were digested with 0.05% trypsin (Invitrogen-Gibco) at 37°C for 5 minutes, harvested, and expanded in larger flasks. By the third to fourth passage, 3–5 × 106 MSCs per donor could be retrieved. MSCs were collected and cryopreserved for further use.
Cytokine measurement
MSCs were resuspended in DMEM supplemented with 10% FBS at a concentration of 1 × 106/ml and then cultured in 24-well plates (Corning, U.S.A.) at the amount of 1 × 105/well in 0.5 ml DMEM culture media in the presence or absence of recombinant human IFN-γ (100 ng/ml, Peprotech, Rocky Hill, NJ 08553, U.S.A.). The supernatant of the culture media was collected every 24 hours until 144 hours after MSCs inoculation, and then assayed for prostaglandin E2 (PGE2), hepatocyte growth factor (HGF) and transforming growth factor (TGF)-β1 by enzyme immunoassay (EIA) (Senxiong, Shanghai, China).
RT-PCR quantification of IDO
MSCs were cultured in 75-cm2 flasks (Corning, U.S.A.) at the concentration of 1 × 106/ml in the presence or absence of recombinant human IFN-γ (100 ng/ml). After culture for 48 hours, cells were collected and RNA was extracted using Trizol (Invitrogen, San Diego, CA, U.S.A.) and reversely transcribed to cDNA using PrimeScript (TaKaRa, Dalian, China). Expression of human IDO transcripts was quantified by semiquantitative RT-PCR according to the manufacturer’s instruction (TaKaRa). The expression levels were compared in these two conditions. Primer sequences were: forward: 5′-AGACTGCTGGTGGAGGACATG-3′, reverse: 5′-AAAGGACAAACTCACGGACTG-3′. The product size was 123 bp. The annealing temperature was 60°C. β-Actin was used as the internal reference, primer sequences were: forward: 5′ GGCACCCAGCACAATGAA 3′, reverse: 5′ GTCATAGTCCGCCTAGAAGCAT 3′.
T lymphocytes proliferation assays
MSCs were cultured in flat-bottomed 96-well plates (Corning, U.S.A.) for 24 hours and irradiated with 30 Gy of γ-ray to inhibit proliferation. Peripheral blood mononuclear cells (PBMC) were separated from healthy donors (major histocompatibility complex (MHC) mismatched with MSCs) and resuspended at a concentration of 5 × 105/ml in RPMI-1640 (Hyclone, U.S.A.) supplemented with 10% FCS and anti-human CD3 monoclonal antibody (mAb) (1 μg/ml, Diaclone, France) and anti-human CD28 mAb 1 μg/ml, Diaclone, Besancon, France). The cell suspension (200 μl/well) was then added to fresh plates or the irradiated MSCs-bound plates (2 × 104/well). After 96 hours of incubation, BrdU labeling solution was added to each well and incubated for another 24 hours. The cell proliferation was analyzed with BrdU ELISA kit (Roche, Germany). Where indicated in the results, recombinant human IFN-γ (100 ng/ml, Peproptech), anti-IFN-γ mAb (5μg/ml, Peproptech, Rehovot, 76703, Israel), PGE2 (2.5–10 μg/ml, Peproptech, Rocky Hill, NJ 08553, U.S.A.), HGF (10–40 ng/ml, Peproptech, Rehovot, 76703, Israel), TGF-β1 (500–2000 pg/ml, Peproptech, Rehovot, 76703, Israel), or Kynurenine (100 μM, Sigma Aldrich, St. Louis, MO, U.S.A.) was added separately into the T lymphocyte culture system and their effects on T cell proliferation were examined by BrdU ELISA kit.
Statistical methods
SPSS 15.0 was used for statistical analysis. Results were presented as mean ± S.E. Data were compared using either paired t-test or ANOVA, where indicated, and were considered significantly different at p < 0.05.
Results
BM-derived MSCs remarkably suppress allogeneic T cell proliferation in vitro
MSCs were derived from normal human bone marrow as described in the methods and their characteristics were confirmed by cell morphology and proliferation analysis. The cells were CD34−, CD45−, CD14−, CD29+, CD44+, CD90+ and CD105+, consistent with immunophenotypes for human MSCs. Effects of MSCs on T lymphocyte proliferation were analyzed in vitro. Proliferation of T lymphocytes was suppressed in the presence of allogeneic MSCs in a dose-dependent manner [Citation2,Citation11] and suppression was detectable at 1:100 ratio (MSCs to T cell responders). A ratio of MSC to T cell responders at 1:5 was selected at subsequent analyses. T-lymphocyte proliferation was significantly inhibited under this experimental condition (p < 0.001). Furthermore, the suppressive effects of MSCs were not MHC restricted.
IFN-γ plays an essential role in the suppressive capacity of MSCs
In the case of aGVHD, MSCs exert their immunosuppressive capacity in an inflammed environment. The role of IFN-γ, which is one of the main pro-inflammatory factor in the process of aGVHD, was examined. T lymphocyte suspension (1 × 105/well) was added to the irradiated MSCs-bound plates (2 × 104/well) in the presence of anti-human CD3 mAb and anti-human CD28 mAb. As shown in , addition of exogenous IFN-γ (100 ng/ml) did not affect the immunosuppressive capacity of MSCs (p = 0.272). In contrast, when neutralizing antibody (anti-IFN-γ mAb, 5μg/ml) was added into the culture system , the effects of MSCs on T cell proliferation was attenuated (p = 0.002). Therefore, IFN-γ plays an essential role in the suppressive properties of MSCs on T cell proliferation.
Figure 1. IFN-γ plays an essential role in the suppressive capacity of MSCs. Under the stimulation of anti-human CD3 mAb and anti-human CD28 mAb, T-lymphocyte proliferation was examined and served as the control group. When cocultured with MSCs, T cell proliferation was significantly inhibited (*p < 0.001). Addition of exogenous IFN-γ (100 ng/ml) did not affect the immunosuppressive capacity of MSCs (p = 0.272). In the blocking study, neutralizing antibody against IFN-γ (anti-IFN-γ mAb, 5 μg/ml) was added into the culture system , the rate of T cell proliferation was partially restrained (*p = 0.002). Results were representative of eight independent experiments, expressed as mean ± S.E, each performed in triplicate.
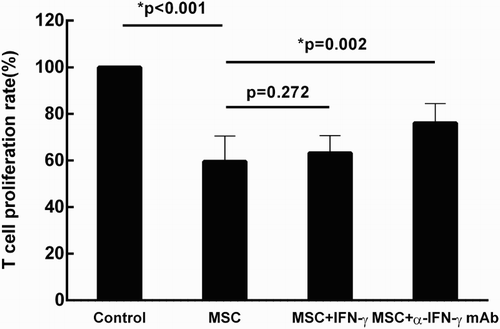
IFN-γ up-regulates the expression of PGE2, HGF, TGF-β1 and IDO by MSCs
In order to dissect the immunosuppressive effects of MSCs, the culture supernatant was examined for levels of three potent immunomodulatory mediators. When MSCs were cultured alone, PGE2, HGF and TGF-β1 were detectable in the culture supernatant within 24–48 hours and their concentrations continually increased until 144 hours after inoculation ((A–C)). This was consistent with previous reports that human MSCs constitutively express immunosuppressive cytokines, such as PGE2, HGF, and TGF-β1 [Citation6,Citation12]. The immunosuppressive functions of these soluble factors in T-lymphocyte proliferation were then examined. As shown in (A,B), addition of exogenous PGE2, HGF, and TGF-β1 significantly suppressed T cell proliferation in a dose-dependent manner. The effect of IFN-γ on cytokine production by MSCs was also examined. Consistently, exogenous IFN-γ significantly increased the production of PGE2, HGF, and TGF-β1 by MSCs at 144 hours after inoculation (p = 0.001, 0.011, and <0.001, respectively, ).
Figure 2. Suppressive role of PGE2, HGF and TGF-β1 on T cell proliferation. T lymphocytes cultured alone (under the stimulation of anti-human CD3 mAb and anti-human CD28 mAb) was served as the control group. Addition of PGE2 (10 μg/ml), HGF (40 ng/ml) and TGF-β1 (2000 pg/ml) significantly suppressed T cell proliferation (*p < 0.001, *p = 0.016, and *p = 0.006, respectively) (A). The immunosuppressive effects of these cytokines intensified in a dose-dependent manner (B). Results were representative of more than six independent experiments, expressed as mean ± S.E, each performed in triplicate.
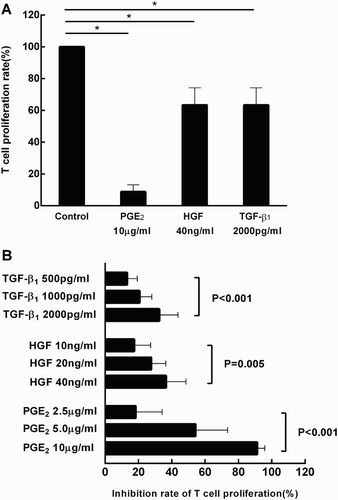
Figure 3. IFN-γ stimulation results in a significant increase in production of PGE2, HGF and TGF-β1 by MSCs. MSCs were cultured in the presence or absence of exogenous IFN-γ (100 ng/ml) for 144 hours. The supernatant was collected and then assayed by EIA. Production of PGE2, HGF and TGF-β1 were significantly enhanced in the presence of IFN-γ. Results were representative of seven independent experiments, expressed as mean ± S.E, each performed in triplicate.
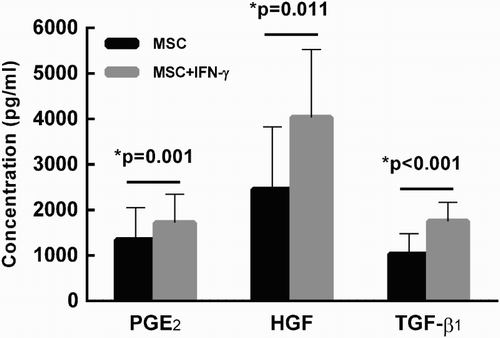
Table 1. MSCs constitutively express immunosuppressive cytokines, including PGE2, HGF and TGF-β1. MSCs were generated from three healthy donors, and then cultured alone in 24-well plates (1 × 105/well) separately. The supernatant of culture media was collected every 24 hours. PGE2(A), HGF(B) and TGF-β1(C) were detectable within 24–48 hours by EIA. With the prolongation of time, concentrations of these cytokines continually increased until 144 hours after MSCs inoculation.
IDO is a tryptophan-catabolizing enzyme that catalyzes the first step in tryptophan catabolism via the kynurenine pathway, and has been identified as a major immunosuppressive effector pathway that inhibits T-cell responses [Citation13,Citation14]. Therefore, we also tested the effect of IFN-γ on IDO expression by MSCs. As shown in , the expression of IDO was not detectable in MSCs by RT-PCR in the absence of IFN-γ, while addition of IFN-γ at a concentration of 1 ng/ml induced the expression of IDO and enhanced IDO expression in a dose-dependent manner. Then a tryptophan metabolite, Kynurenine (100 μM), was added into the T lymphocyte culture system in the absence of MSCs. T cell proliferation was significantly restrained (p = 0.004, ), suggesting that immunosuppression may be mediated through the local accumulation of tryptophan metabolites and that IDO may contribute to IFN-γ-mediated MSC immunomodulation.
Figure 4. IFN-γ up-regulates IDO expression by MSCs. When MSCs were cultured alone, IDO mRNA was not detectable by RT–PCR, but the expression level was remarkably enhanced when MSCs were cultured in the presence of IFN-γ (100 ng/ml) (A). The IDO expression was examined under different IFN-γ concentrations, even at the concentration of 1 ng/ml, it could still be detected (B).
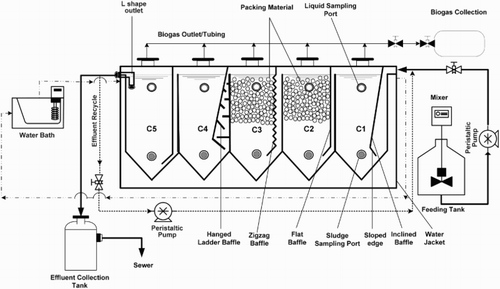
Figure 5. Kynurenine directly inhibits T cell proliferation, suggesting that IDO may contribute to IFN-γ-mediated MSC immunomodulation. T lymphocytes cultured alone (under the stimulation of anti-human CD3 mAb and anti-human CD28 mAb) was served as the control group. When exogenous kynurenine (100 μΜ) was added into the culture system, the rate of T cell proliferation was significantly restrained (p = 0.004). Results were representative of six independent experiments, expressed as mean ± S.E, each performed in triplicate.

Discussion
Bone marrow MSCs were first described in 1968 by Friedenstein and coworkers. In 2006, the Mesenchymal and Tissue Stem Cell Committee of the International Society for Cellular Therapy (ISCT) proposed the ‘three minimal criteria’ for the definition of MSCs [Citation15]. MSCs have gained considerable interest as a promising choice for cell therapy because of their unique combined properties of immunomodulation and self-renewal and multilineage differentiation capacity.
MSCs demonstrate immunosuppressive functions by suppressing T cell proliferation and dendritic cell maturation, reducing B-cell activation and proliferation, inhibiting the proliferation and cytotoxicity of NK cells and promoting the generation of regulatory T cells [Citation16]. Although the precise mechanisms are not fully understood, several main mechanisms have been proposed including cell-cell contact, release of soluble factors, and generation of regulatory lymphocytes. In particular, soluble factors produced by MSCs have been associated with the immune-modulatory capacity on T-lymphocyte activation and proliferation.
In the present study, we confirmed that the immunosuppressive functions of MSCs in vitro are not MHC restricted. We found that MSCs constitutively secreted low levels of immunosuppressive cytokines such as PGE2, HGF and TGF-β1 and that addition of IFN-γ significantly increased their production by MSCs. Expression of IDO by activated MSCs is another T-cell inhibitory pathway [Citation17–19]. We found that stimulation with IFN-γ increased the IDO expression level in MSCs. The MSCs production of suppressive molecules may depend on a cross talk between MSCs and activated T-cells as the culture supernatant of MSCs alone did not suppress T cell proliferation [Citation20–22], while supernatant in the presence of T-cells was suppressive [Citation23]. Therefore, MSCs themselves are objects of cytokine regulation. Thus, INF-γ is an essential factor to license (or prime) MSCs for their immunoregulatory functions [Citation5,Citation24–26].
MSCs expanded ex vivo have been used successfully in clinical trials to treat ongoing and steroid-refractory GVHD. These IFN-γ ‘activated MSC’ present a new strategy regulating allogeneic T cell proliferation and will be helpful for the optimization of therapeutic use of MSCs.
Disclosure statement
No potential conflict of interest was reported by the authors.
References
- Pittenger MF, Mackay AM, Beck SC, et al. Multilineage potential of adult human mesenchymal stem cells. Science. 1999;284(5411):143–147. doi: 10.1126/science.284.5411.143
- Tse WT, Pendleton JD, Beyer WM, et al. Suppression of allogeneic T-cell proliferation by human marrow stromal cells: implications in transplantation. Transplantation. 2003;75(3):389–397. doi: 10.1097/01.TP.0000045055.63901.A9
- Uccelli A, Moretta L, Pistoia V. Immunoregulatory function of mesenchymal stem cells. Eur J Immunol. 2006;36(10):2566–2573. doi: 10.1002/eji.200636416
- Klyushnenkova E, Mosca JD, Zernetkina V, et al. T cell responses to allogeneic human mesenchymal stem cells: immunogenicity, tolerance, and suppression. J Biomed Sci. 2005;12(1):47–57. doi: 10.1007/s11373-004-8183-7
- Bernardo ME, Fibbe WE. Mesenchymal stromal cells: sensors and switchers of inflammation. Cell Stem Cell. 2013;13(4):392–402. doi: 10.1016/j.stem.2013.09.006
- Kyurkchiev D, Bochev I, Ivanova-Todorova E, et al. Secretion of immunoregulatory cytokines by mesenchymal stem cells. World J Stem Cells. 2014;6(5):552–570. doi: 10.4252/wjsc.v6.i5.552
- Haddad R, Saldanha-Araujo F. Mechanisms of T-cell immunosuppression by mesenchymal stromal cells: what do we know so far? Biomed Res Int. 2014;2014:216806.
- Di Nicola M, Carlo-Stella C, Magni M, et al. Human bone marrow stromal cells suppress T-lymphocyte proliferation induced by cellular or nonspecific mitogenic stimuli. Blood. 2002;99(10):3838–3843. doi: 10.1182/blood.V99.10.3838
- Blanc KL, Frassoni F, Ball L, et al. Mesenchymal stem cells for treatment of steroid-resistant, severe, acute graft-versus-host disease: a phase II study. Lancet. 2008;371(9624):1579–1586. doi: 10.1016/S0140-6736(08)60690-X
- Muroi K, Miyamura K, Okada M, et al. Bone marrow-derived mesenchymal stem cells (JR-031) for steroid-refractory grade III or IV acute graft-versus-host disease: a phase II/III study. Int J Hematol. 2016;103(2):243–250. doi: 10.1007/s12185-015-1915-9
- Yi HG, Yahng SA, Kim I, et al. Allogeneic clonal mesenchymal stem cell therapy for refractory graft-versus-host disease to standard treatment: a phase I study. Korean J Physiol Pharmacol. 2016;20(1):63–67. doi: 10.4196/kjpp.2016.20.1.63
- Bartholomew A, Sturgeon C, Siatskas M, et al. Mesenchymal stem cells suppress lymphocyte proliferation in vitro and prolong skin graft survival in vivo. Exp Hematol. 2002;30(1):42–48. doi: 10.1016/S0301-472X(01)00769-X
- Ryan JM, Barry F, Murphy JM, et al. Interferon-γ does not break, but promotes the immunosuppressive capacity of adult human mesenchymal stem cells. Clin Exp Immunol. 2007;149(2):353–363. doi: 10.1111/j.1365-2249.2007.03422.x
- King NJ, Thomas SR. Molecules in focus: indoleamine 2,3-dioxygenase. Int J Biochem Cell Biol. 2007;39(12):2167–2172. doi: 10.1016/j.biocel.2007.01.004
- Dominici M, Le Blanc K, Mueller I, et al. Minimal criteria for defining multipotent mesenchymal stromal cells. The International Society for Cellular Therapy Position Statement. Cytotherapy. 2006;8(4):315–317. doi: 10.1080/14653240600855905
- Glenn JD, Whartenby KA. Mesenchymal stem cells: emerging mechanisms of immunomodulation and therapy. World J Stem Cells. 2014;6(5):526–539. doi: 10.4252/wjsc.v6.i5.526
- Meisel R, Zibert A, Laryea M, et al. Human bone marrow stromal cells inhibit allogeneic T-cell responses by indoleamine 2,3-dioxygenase-mediated tryptophan degradation. Blood. 2004;103(12):4619–4621. doi: 10.1182/blood-2003-11-3909
- Li X, Xu Z, Bai J, et al. Umbilical cord tissue-derived mesenchymal stem cells induce T lymphocyte apoptosis and cell cycle arrest by expression of indoleamine 2, 3-dioxygenase. Stem Cells Int. 2016;2016:7495135.
- Jones BJ, Brooke G, Atkinson K, et al. Immunosuppression by placental indoleamine 2,3-dioxygenase: a role for mesenchymal stem cells. Placenta. 2007;28(11–12):1174–1181. doi: 10.1016/j.placenta.2007.07.001
- Augello A, Tasso R, Negrini SM, et al. Bone marrow mesenchymal progenitor cells inhibit lymphocyte proliferation by activation of the programmed death 1 pathway. Eur J Immunol. 2005;35(5):1482–1490. doi: 10.1002/eji.200425405
- Rasmusson I, Ringdén O, Sundberg B, et al. Mesenchymal stem cells inhibit lymphocyte proliferation by mitogens and alloantigens by different mechanisms. Exp Cell Res. 2005;305(1):33–41. doi: 10.1016/j.yexcr.2004.12.013
- Le Blanc K, Rasmusson I, Götherström C, et al. Mesenchymal stem cells inhibit the expression of CD25 (interleukin-2 receptor) and CD38 on phytohaemagglutinin-activated lymphocytes. Scand J Immunol. 2004;60(3):307–315. doi: 10.1111/j.0300-9475.2004.01483.x
- Djouad F, Plence P, Bony C, et al. Immunosuppressive effect of mesenchymal stem cells favors tumor growth in allogeneic animals. Blood. 2003;102(10):3837–3844. doi: 10.1182/blood-2003-04-1193
- Dazzi F, Krampera M. Mesenchymal stem cells and autoimmune diseases. Best Pract Res Clin Haematol. 2011;24(1):49–57. doi: 10.1016/j.beha.2011.01.002
- Waterman RS, Tomchuck SL, Henkle SL, et al. A new mesenchymal stem cell (MSC) paradigm: polarization into a pro-inflammatory MSC1 or an immunosuppressive MSC2 phenotype. PLoS ONE. 2010;5(4):e10088. doi: 10.1371/journal.pone.0010088
- English K. Mechanisms of mesenchymal stromal cell immunomodulation. Immunol Cell Biol. 2013;91(1):19–26. doi: 10.1038/icb.2012.56