ABSTRACT
Background
In chronic immune thrombocytopenic purpura (ITP), rituximab removes the harmful autoantibodies through antibody-dependent cellular cytotoxicity. The response to rituximab in ITP is variable; the effectiveness of rituximab is influenced by the process of activation of effector fragment C gamma receptors (FcγRs). Genetic factors may affect the response to rituximab.
Objectives
The influence of FcγRIIa (H131R) and FcγRIIIa (V158F) gene polymorphisms on the response to rituximab in ITP.
Methods
One hundred ITP patients were genotyped for FcγRIIa (H131R) and FcγRIIIa (V158F) gene polymorphisms using the polymerase chain reaction-restriction fragment length polymorphism assay. The response at the end of the third month was assessed by direct platelets count. Polymorphisms were analyzed in relation to the response.
Results
The mean platelets count at end of weeks 1–4 of rituximab was statistically significantly higher in patients who achieved complete response (CR) than partial response or no response (P-value = .001). Although RR (44.4%) and HR (38.9%) genotypes were observed to be higher in patients who achieved CR compared with the wild (HH) genotype (16.7%), it was not statistically significantly different (P-value = .648).
Conclusion
The higher platelet count achieved early is predictive for a better response to rituximab later. FCγRIIA polymorphisms did not significantly influence response to rituximab in ITP.
Introduction
Immune thrombocytopenic purpura (ITP) is an autoimmune disorder characterized by a low circulating platelet count caused by the destruction of antibody-sensitized platelets in the reticuloendothelial system; impaired production of platelet is also implicated [Citation1]. Corticosteroid is the ministry of ITP treatment. Patients who fail to respond to steroid (steroid resistant) or who relapse (steroid dependent) face the options of treatment with second-line drug therapy or splenectomy. Rituximab is one of the second-line drug therapies [Citation2].
Rituximab is a chimeric IgG1 monoclonal antibody (mAbs). The major mechanism of action of rituximab is the antibody-dependent cellular cytotoxicity (ADCC), where the Fab domain of rituximab binds to the CD20 antigen on B lymphocytes, and the Fc domain recruits immune effector cells to mediate B-cell lysis [Citation3]. In chronic ITP, rituximab eliminates autoreactive B-cell clones through ADCC and simply removes the source of harmful autoantibodies [Citation4]. ADCC effectiveness of rituximab is influenced by the process of activation of effector cells via their immunoglobulin G fragment C gamma receptors (FcγRs) [Citation5].
Leukocyte FcγRs are divided into three biochemically and structurally distinct classes that differ in their affinity for IgG subclasses, FcγRI (CD64), FcγRII (CD32), and FcγRIII (CD16); there are at least 12 subclasses [Citation6]. FcγRIII binds IgG1 and IgG3 better than IgG2 and IgG4 [Citation7]. FcγRIIa has a unique capacity to bind to IgG2 [Citation8].
Three FcγR subclasses, FcγRIIA, FcγRIIIA, and FcγRIIIB, display functional polymorphisms [Citation9]. Differential response to therapeutic mAbs has been reported to correlate with specific polymorphisms in two of FcγR genes: FcγRIIa (H131R) and FcγRIIIa (V158F) in some diseases [Citation5,Citation10]. In previous studies, patients carrying the homozygous mutant FcγRIIIa 158 V genotype were significantly associated with a higher response rate to IgG1, which may be explained by a higher affinity of FcγRIIIa of the V allele to human IgG1 than does the F allele, and mediates ADCC more effectively [Citation11]. The H allele of FCYRIIa (H131R) binds to human IgG2 better than that of the R allele [Citation12,Citation13]. However, the influence of both polymorphisms on the response rate to rituximab in lymphoma is still controversial [Citation14,Citation15].
Patients and methods
Clinical and hematologic analysis
In this study, 100 patients with ITP were recruited from the outpatient clinic and department of the clinical hematology in Kasr El-Einy hospital. The patients were grouped according to the type of second-line therapy received as: rituximab-treated group and non-rituximab-treated group; 50 patients were treated with rituximab as second-line therapy at a dose of 375 mg/m2 weekly for 4 weeks; and 50 patients were treated with second-line therapy – other than rituximab including: azathioprine, pulse dexamethasone every 28 days, danazol, IVIG, eltrombopag and splenectomy. All patients had given written informed consent before enrollment in the study. The study protocol was in accordance with the local hospital research guidelines.
Inclusion criteria, including adult individuals (>18 years) both sex but non-pregnant females, are only enrolled. All patients should be diagnosed as ITP for a duration of 3 months or more, that is, persistent ITP (duration of the disease = 3–12 month) and chronic ITP (duration >12 months). The enrolled patients are either steroid dependent or steroid resistant. Steroid dependence was considered when there was need for ongoing or repeated doses administration of corticosteroids for at least 2 months to maintain a platelet count at or above 30 × 109/l and/or to avoid bleeding. Steroid resistance was considered for those who fail to respond to treatment with methylprednisone after 2 weeks or dexamethasone for 4 weeks [Citation16].
All patients underwent history taking and full examination. Evaluation of platelets counts was done initially before starting second-line therapy then weekly for 1 month then monthly for 3 months. At the end of the third month, the response to second-line therapy was considered as follows; complete response (CR), which is defined as any platelet count of at least 100 × 109/l, partial response (PR), which is defined as any platelet count between 30 and 100 × 109/l and at least doubling of the baseline count, no response (NR) was considered if the platelet count was lower than 30 × 109/l or less than doubling of the baseline count [Citation16].
Molecular analysis
Blood samples and DNA extraction: Blood samples of 5 ml were obtained from all participants, collected in sterile EDTA tubes, and then stored at −20°C. Genomic DNA of patients and healthy controls was extracted from whole blood using an established protocol for DNA extraction from blood samples using a DNA extraction kit (Qiagen, Hilden, Germany, catalog number 51104).The extracted DNA was amplified by the polymerase chain reaction (PCR) using the recombinant Taq polymerase master mix (Qiagen,Catalog number 201443).
PCR analysis: PCR reactions were carried out in 25 μl volumes comprising 12.5 μl Taq PCR master mix (0.1 units/μl Taq DNA polymerase, 32 mM (NH4)2 SO4, 130 mM Tris HCl, 5.5 mM MgCl2, and 0.4 mM of each dNTP), 5.5 μl of distilled water, 5 μl of template DNA and 1 μl of each primer. Primers were supplied in a lyophilized form. Primers were prepared according to the manufacturer’s instructions by adding the required volumes of nuclease-free water (supplied with the master mix) to the vials supplied so that each 1 μl contains 25 pmol of the primer. After preparation, it was stored at −20°C. The primers for FcγRIIa gene and FcγRIIIa gene were prepared as described before [Citation17,Citation18]. For the FcγRIIa gene: forward primer: 5′-GGAAAATCCCAGAAATTCTCGC-3′; reversed primer: 5′-CAACAGCCTGACTACCTATTACGCGGG-3′. For the FcγRIIIa gene: First cycle primers: forward primer: 5′ATATTTACAGAATGGCACAGG-3′; reversed primer: 5′ GACTTGGTACCCAGGTTGAA-3′. Second cycle primers: forward primer: 5′-TCAGATTCGATCCTACTTCTGCAGGGGGCAT-3′; reversed primer: 5′-ACGTGCTGAGCTTGAGTGATGGTGATGTTCAC-3′.
The PCR amplifications were carried out using the thermal cycler Applied Biosystems 9600 (Perkin Elmer). The main cycling parameters were 94°C for 4 min for initial denaturation, followed by 35 cycles of denaturation at 94°C for 30 s and annealing at 63°C for 30 s with the final extension being at 72°C for 5 min. The PCR product was run in 4% agarose gel.
Detection of the FcγRIIa gene H131R genetic polymorphism was done using the PCR-restriction fragment length polymorphism (RFLP) technique. The restriction enzyme BSH 12361 was used. The PCR product, which is 337 bp, will be cleaved into two products 316, and 21 bp. The 21 bp product is not seen on the gel and only the 316 bp product is detected. Therefore, in individuals lacking this polymorphism, the cleavage will not occur and a 337 bp band is detected. Both 337 and 316 bp bands are seen in individuals heterozygous for the mutation. The subjects were considered as: normal FcγRIIa gene (wild type) (HH genotype) if only one band 337(bp) is detected and homozygous mutation of the gene (RR homotype) if only one band (316 bp) is detected. Heterozygous mutation of the gene (HR heterotype) if the two bands (337 bp) and (316 bp) are present ().
Figure 1. RFLP analysis of FcγRIIa polymorphisms. Lane 1: DNA marker (50–1000 bps). Lanes 5, 7, 8, 10, 11: heterozygous HR (316 bp and 337 bp). Lanes 3, 4, 6, 9: homozygous RR (316 bp). Lane 2: Wild type HH (337 bp).
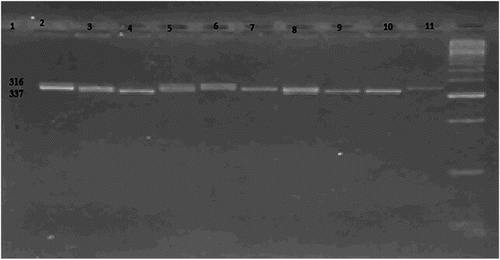
Detection of FcγRIIIa V-158 Fgenetic polymorphism: Genotyping of FcγIIIa polymorphism was performed using the PCR-RFLP technique. The initial PCR product from the first cycle is 1.2 kilobase fragments containing the polymorphic site and the second PCR product is 94 bp. This PCR product which is 94 bp will be cleaved into two products 61 and 33 bp. Therefore, in individuals lacking this mutation (wild type), cleavage will not occur. No digestion occurs and the original 94 bp band is seen. The original 94 bp band and the cleaved 61 and 33 bp bands are seen in individuals heterozygous for the mutation. The subjects were considered as: normal FcγIIIa gene (wild type) (FF genotype) if only one band (94 bp) is detected. Homozygous mutation of the FcγIIIa gene (VV genotype) if only two bands (61 bp) and (33 bp) are detected. Heterozygous mutation of the FcγIIIa gene (FV genotype) if the three bands (94 bp), (61 bp) and (33 bp) are present ().
Statistical methods
Data were transferred to the Statistical Package of Social Science Software program, version 21 (SPSS), to be statistically analyzed. Data were summarized using range, mean, standard deviation and median for quantitative variables or frequency and percentage for qualitative ones. Comparison between groups was performed using the Kruskal Wallis test and the Mann Whitney test (pairwise comparisons) for quantitative variables, while comparison for qualitative variables was performed through the chi square or Fisher’s exact test. P-values less than .05 were considered statistically significant.
Results
Out of the 100 ITP patients included in this study, 7 patients were males (7%) and 93 patients were female (93%), age ranged from 17 to 60 years (mean age = 35.5±). Nineteen patients (19%) were diagnosed as persistent ITP and 81 patients (81%) were diagnosed as chronic ITP. According to the initial response to steroid as first-line therapy, 67 patients (67%) were steroid dependent and 33 patients (33%) were steroid resistant.
Out of the 50 patients who received second-line therapy other than rituximab; 21 patients (42%) were treated with splenectomy, 17 patients (34%) received azathioprine, 6 patients (12%) received pulse dexamethasone every 28 days, 4 patients (8%) received danazol, 1 patient (2%) received IVIG, and 1 patient (2%) received eltrombopag.
At the end of the third month of second-line therapy, out of 100 patients, 43 patients (43%) achieved CR, 37 patients (37%) achieved PR and 20 patients (20%) had NR.
In the rituximb group, by the end of the third month, 18 patients (36%) achieved CR, 19 patients (38%) achieved PR and 13 patients (26%) achieved NR. The total response was 72%.
The mean value of platelet count at end of weeks 1–4 of rituximab therapy shows statistically significant differences (P-value .001) being higher in patients who achieved CR than who achieved PR or NR. The mean platelet counts for patients who achieved CR, PR and NR are (129.9 ± 200.1), (49.2 ± 40.1), (18.6 ± 10.8), respectively, at the end of week 1 (149.2 ± 218.8), (43.8 ± 26.4), (25.0 ± 19.3), respectively, at the end of week 2 (153.7 ± 123.0), (43.2 ± 35.3), (30.2 ± 23.7), respectively, at the end of week 3 (143.7 ± 65.6), (52.6 ± 41.3), (29.9 ± 20.6), respectively, at the end of week 4 ().
Table 1. Platelets count at the end of week 1, week 2, week 3 and week 4 of rituximab.
Regarding the FcγRIIa gene (H131R) polymorphism distribution in 100 patients; 28 patients (28%) have wild genotype (HH), 41 patients (41%) have heterozygous genotype (HR) and 31 (31%) have mutant homozygous genotype (RR).
Regarding the FcγRIIIa (V158F) gene polymorphism, in our study, 100 ITP patients included showed the wild FF genotype.
Among the 50 patients who were treated with rituximab; out of the 18 patients who achieved CR, 8/18 patients (44.4%) carried the FcγRIIa homozygous mutant genotype (RR) and 7/18 patients (38.9%) carried the heterozygous genotype (HR), compared with 3/18 patients (16.7%) carrying the wild genotype (HH). Regarding the 13 patients who achieved NR, the lowest rate was for patients carrying the mutant homozygous genotype (RR) 3/13 patients (23.1%), compared with 5/18 patients (38.5%) carrying the heterozygous genotype (HR) and 5/18 patients (38.5%) carrying the wild genotype (HH); although better response was on RR arm, it was not statistically significantly different (P-value = .648) ().
Table 2. The distribution of FcγRIIa-131H/R genotypes according to response to rituximab.
No statistically significant difference was found between group I and group II, regarding the response according to FcγRIIa gene polymorphism ().
Table 3. Comparison between the response in both groups regarding FcγRIIa gene polymorphisms.
Discussion
Rituximab is a monoclonal antibody directed against CD20 antigen on the surface of autoreactive B-cell clones through ADCC and simply removes the source of harmful autoantibodies in chronic ITP. The response to rituximab among ITP patients varies; the effectiveness of rituximab is influenced by the process of activation of effector FcγRs [Citation5]. Genetic factors may be involved in determining the response to rituximab. We analyzed FcγRIIa (H131R) and FcγRIIIa (V158F) gene polymorphisms in 100 ITP patients divided into 2 equal groups, one group received rituximab and the other group received non-rituximab second-line therapy. We assessed the response at the end of the third month by direct platelets count and compared the response with genotypes.
Out of the 100 patients included in this study; 28 patients (28%) had wild genotype (HH), 41 patients (41%) had heterozygous genotype (HR) and 31 (31%) had mutant homozygous genotype (RR) of FCγRIIA 131 H/R gene polymorphism.
We analyzed the frequency of FcγRIIa genotypes (131H/H, H/R, R/R) in 50 ITP patients receiving rituximab, we observed that FCγRIIA 131 Rhomo/heterozygous mutant genotypes are associated with a better response to rituximab, however it was not statistically significant (P-value = .648). This may be explained by insignificant difference in the affinity of both FcγRIIa alleles (131 H/H or 131 R/R) for human IgG1 unlike IgG2 which has a higher affinity for the 131 H/H allele than that of the 131 R/R allele [Citation12].
In contrast to our study, Peñalver et al. studied the influence of FCγRIIA 131 H/R gene polymorphism on the response to rituximab in 45 ITP patients, regarding genotype distribution they observed that 11 patients (25%) carry HH genotype, 10 (22%) carry RR genotype and 24 (53%) were heterozygous. They reported a tendency to a better response in FCγRIIA 131H homo- and heterozygous patients (P-value = .08), the response rate was significantly worse in FCγRIIA 131R homozygotes than in 131H carriers (P-value = .028) [Citation19].
Fujimoto et al. observed that the distribution of FcγRIIa H/R polymorphism was not significantly different between ITP patients and controls, and did not correlate with the responsiveness to treatment in a study conducted on 104 adult chronic ITP patients and in 59 healthy control subjects [Citation20].
In our study, the analysis of FCγRIIIA 158 V/f gene polymorphism showed that all 100 ITP patients carry the wild FcγRIIIa (FF) genotype. In contrast to our study, the data obtained by Peñalver et al. found that 8/45 ITP patients (18%) were homozygous for FCγRIIIA 158 V and 21/45 (47%) wild genotype (FF), while 16/45 (35%) were heterozygous [Citation19].
Eyada et al. studied the frequency of FcγRIIIa-158F/V gene polymorphism in 92 Egyptian children with ITP and healthy controls to find out if the polymorphism is a risk factor for ITP, unlike our findings, they reported a high frequency of the V allele, and FcγRIIIa FV heterotype was significantly higher in ITP patients compared with controls [Citation21].
Conclusion
The higher platelet count achieved early (end of weeks 1–4) after rituximab is predictive for a better response later (at end of M3). FCγRIIA polymorphisms did not significantly influence the response rate to rituximab in ITP patients.
Disclosure statement
No potential conflict of interest was reported by the authors.
References
- Schwartz RS. Immune thrombocytopenic purpura from agony to agonist. N Engl J Med. 2007;357(22):2299–2301. doi: 10.1056/NEJMe0707126
- Provan D, Stasi R, Newland AC, et al. International consensus report on the investigation and management of primary immune thrombocytopenia. Blood. 2010;115:168–186. doi: 10.1182/blood-2009-06-225565
- Reff ME, Carner K, Chambers KS, et al. Depletion of B cells in vivo by a chimeric mouse human monoclonal antibody to CD20. Blood. 1994;83:435–445.
- Koulova L, Alexandrescu D, Dutcher JP, et al. Rituximab for the treatment of refractory idiopathic thrombocytopenic purpura (ITP) and thrombotic thrombocytopenic purpura (TTP): report of three cases. Am J Hematol. 2005;78(1):49–54. doi: 10.1002/ajh.20243
- Weng WK, Levy R. Two immunoglobulin G fragment C receptor polymorphisms independently predictresponse to rituximab in patients with follicular lymphoma. J Clin Oncol. 2003;21(21):3940–3947. doi: 10.1200/JCO.2003.05.013
- Hulett MD, Hogarth PM. Molecular basis of Fc receptor function. Adv Immunol. 1994;57:1–127. doi: 10.1016/S0065-2776(08)60671-9
- Radaev S, Sun P. Recognition of immunoglobulins by Fcγ receptors. Mol Immunol. 2002;38:1073–1083. doi: 10.1016/S0161-5890(02)00036-6
- Van der Pol WL, Van de Winkel JGJ. Receptor polymorphisms: risk factors for disease. Immunogenetics. 1998;48:222–232. doi: 10.1007/s002510050426
- van Sorge NM, van der Pol WL, van de Winkel JG. Fc gamma R polymorphisms: implications for function, disease susceptibility and immunotherapy. Tissue Antigens. 2003;61:189–202. doi: 10.1034/j.1399-0039.2003.00037.x
- Mellor JD, Brown MP, Irving HR, et al. A critical review of the role of Fc gamma receptor polymorphisms in the response to monoclonal antibodies in cancer. J Hematol Oncol. 2013;6:1. doi: 10.1186/1756-8722-6-1
- Wu J, Edberg JC, Redecha PB, et al. A novel polymorphism of Fc-gamma-RIIIa (CD16) alters receptor function and predisposes to autoimmune disease. J Clin Invest. 1997;100:1059–1070. doi: 10.1172/JCI119616
- Parren PW, Warmerdam PA, Boeije LC, et al. On the interaction of IgG subclasses with the low affinity Fc-gamma-RIIa (CD32) on human monocytes, neutrophils, and platelets: analysis of a functional polymorphism to human IgG2. J Clin Invest. 1992;90:1537–1546. doi: 10.1172/JCI116022
- Warmerdam PA, van de Winkel JG, Vlug A, et al. A single amino acid in the second Ig-like domain of the human Fc gamma receptor II is critical for human IgG2 binding. J Immunol. 1991;147(4):1338–1343.
- Ghesquieres H, Cartron G, Seymour JF, et al. Clinical outcome of patients with follicular lymphoma receiving chemoimmunotherapy in the PRIMA study is not affected by FCGR3A and FCGR2A polymorphisms. Blood. 2012;120(13):2650–2657. doi: 10.1182/blood-2012-05-431825
- Dornan D, Spleiss O, Yeh RF, et al. Effect of FCGR2A and FCGR3A variants on CLL outcome. Blood. 2010;116(20):4212–4222. doi: 10.1182/blood-2010-03-272765
- Rodeghiero F, Stasi R, Gernsheimer T, et al. Standardization of terminology, definitions and outcome criteria in immune thrombocytopenic purpura of adults and children: report from an international working group. Blood. 2009;113:2386–2393. doi: 10.1182/blood-2008-07-162503
- Israelsson E, Vafa M, Maiga B, et al. Differences in Fcgamma receptor IIa genotypes and IgG subclass pattern of anti-malarial antibodies between sympatric ethnic groups in Mali. Malar J. 2008;15:7–175.
- Koene HR, Kleijer M, Algra J, et al. Fc gammaRIIIa-158 V/F polymorphism influences the binding of IgG by natural killer cell Fc gammaRIIIa, independently of the Fc gammaRIIIa-48L/R/H phenotype. Blood. 1997;90(3):1109–1114.
- Peñalver J, Vilches C, Jarque I, et al. Influence of the FCGR3A-158 V/F and FCGR2A-131 H/R polymorphism on the response to rituximab therapy in immune thrombocytopenic purpura and autoimmune hemolytic Anemia. Blood. 2007;110(11):2097.
- Fujimoto TT, Inoue M, Shimomura T, et al. Involvement of Fc gamma receptor polymorphism in the therapeutic response of idiopathic thrombocytopenic purpura. Br J Haematol. 2001;115:125–130. doi: 10.1046/j.1365-2141.2001.03109.x
- Eyada TK, Farawela HM, Khorshied MM, et al. FcγRIIa and FcγRIIIa genetic polymorphisms in a group of pediatric immune thrombocytopenic purpura in Egypt. Blood Coagul Fibrinolysis. 2012;23(1):64–68. doi: 10.1097/MBC.0b013e32834ddf2f