ABSTRACT
Objectives: This study aimed to evaluate the effects of REGγ knockdown on the proliferation, apoptosis and migration of multiple myeloma (MM) cells, and reveal the potential regulatory mechanisms.
Methods: The expression of REGγ on myeloma cells of 28 MM patients was detected by Western blot. shRNA-REGγ-1 and shRNA-REGγ-2 were constructed to downregulate REGγ in RPMI-8226 cells. The proliferation, apoptosis and migration of transfected cells were analyzed by Cell Counting Kit 8 (CCK8), flow cytometry and transwell chamber, respectively. The expression of phosphorylated p65 (p-p65), p65, NF-kappa-B inhibitor ε (IkBε), matrix metalloproteinase 2 (MMP2), B-cell lymphoma xL (Bcl-xL) and X-linked inhibitor of apoptosis protein (XIAP) in transfected cells was detected by Western blot. Using cycloheximide (CHX), the half-life period of IkBε was detected by Western blot.
Results: The expression of REGγ was positive in myeloma cells. The proliferation and migration of RPMI-8226 cells were significantly inhibited by shRNA-REGγ-1/shRNA-REGγ-2, while the apoptosis rates were significantly increased (p < 0.05). The expression of p-p65 and IkBε was significantly reduced in RPMI-8226 cells transfected with shRNA-REGγ-1/shRNA-REGγ-2. The degradation of IkBε was significantly lower in RPMI-8226 cells transfected with shRNA-REGγ-1 than the control (longer half-life period). Besides, the expression of MMP2, Bcl-xL and XIAP in RPMI-8226 cells was significantly inhibited by shRNA-REGγ-1/shRNA-REGγ-2.
Discussion: Knockdown of REGγ may inhibit the proliferation and migration, and promote the apoptosis of RPMI-8226 cells possibly by downregulating NF-κB signal pathway.
Introduction
Multiple myeloma (MM) is a malignancy of plasma cells characterized by uncontrolled growth of malignant plasma cells in the bone marrow [Citation1]. According to statistics, MM accounts for more than 10% hematological cancers and 2% annual cancer-related death [Citation2]. MM can induce the occurrence of various severe symptomatic complications, such as bleeding, infection, renal failure, pathologic fractures and spinal cord compression [Citation3]. In spite of great advances in the development of novel agents, such as bortezomib, lenalidomide and thalidomide, the prognosis of MM patients remains poor due to the emergence of drug resistance [Citation4]. Following the rapid development of molecular biology, many molecular factors have been revealed to be involved in the pathogenesis of MM, such as vascular endothelial growth factor, transforming growth factor-β (TGF-β), phosphatidylinositol-3-kinase, mitogen-activated protein kinase and nuclear factor κB (NFκB) [Citation5]. Molecular targeted therapy has become an effective potential strategy in the treatment of MM.
REGγ, also known as proteasome activator subunit 3, is an ATP- and ubiquitin-independent proteasome activator [Citation6]. It is evident that REGγ is involved in the progression of cancers [Citation7,Citation8]. REGγ is overexpressed in multiple human cancers, such as breast cancer, thyroid cancer, colorectal cancer, lung and liver cancers [Citation7,Citation9]. The action mechanisms of REGγ in cancers are complex, relating with various molecular factors and pathways. It has been reported that REGγ promotes the growth of, and inhibits, the apoptosis of breast cancer cells by degrading p21 and suppressing the activation of natural killer [Citation10]. The proliferation, motility, and invasion of MCF7 and BT474 breast cancer cells are significantly inhibited by REGγ knockdown, and this process is closely related with the degradation of ERα via ubiquitin-proteasome pathway [Citation11]. Meanwhile, depletion of REGγ influences the proliferation, cell cycle progression, proteasome activity and drug resistance of H1299 cells by altering the cellular response to p53/TGF-β signaling [Citation12]. However, related research works on the specific roles and molecular mechanisms of REGγ in MM are still limited.
Nuclear factor κB (NF-κB) is an important transcription factor in the regulation of inflammatory response and cellular proliferation [Citation13]. By regulating diverse genes involved in proliferation, apoptosis and drug resistance, NF-κB signal pathway plays a key role in the pathogenesis of various cancers, including MM [Citation14]. Activated NF-κB is usually presented in MM cells, considering as an important prognostic biomarker and therapeutic target of MM [Citation15]. In this study, shRNA-REGγ-1 and shRNA-REGγ-2 were constructed to downregulate REGγ, and the proliferation, apoptosis and migration of transfected RPMI-8226 cells were evaluated. Meanwhile, the expression of several key factors involved in NF-κB signal pathway was detected. Our findings may not only identify the effects of REGγ knockdown on the proliferation, apoptosis and migration of MM cells but also reveal the potential regulatory mechanisms relating with NF-κB signal pathway.
Materials and methods
Patients
A total of 28 MM patients (15 males and 13 females, 18–86 years old) were screened from Guangdong Second General Hospital between May 2014 and May 2016. MM was diagnosed according to the <Diagnostic and therapeutic criteria of hematologic diseases> and <Guidelines for the diagnosis and treatment of multiple myeloma in China (Revised version 2015)> [Citation16]. According to the International staging system (ISS), MM patients included 3 cases of stage I, 10 cases of stage II and 15 cases of stage III (). Bone marrow samples (3–5 ml) of MM patients were collected by the bone marrow aspiration on posterior superior or anterior superior iliac crest using a puncture needle (#16, BM 11/7 GALLINI s.r.l, Italy) (heparin sodium anticoagulation). Normal bone marrow samples were obtained from allogeneic hematopoietic stem cell transplant donors (n = 16). Myeloma and non-myeloma plasma cells were isolated from the bone marrow aspiration of MM patients and donors, respectively. This study was approved by the Ethics committee of Guangdong Second Provincial General Hospital and informed consent was obtained from all subjects.
Table 1. Clinical characteristics of MM patients and normal donors.
Vectors construction and cell transfection
The vectors of shRNA-REGγ-1 and shRNA-REGγ-2 were constructed to downregulate REGγ. The special complementation fragments of REGγ (shREGγ-1: 5′-CAGAAGACUUGGUGGCAAA-3′, shREGγ-2: 5′-GCAUCUUAUCUGGACCAGAUUUCUA-3′; shN: 5′-UUCUCCGAACGUGUCACGUTT-3′) were synthesized by Guangzhou Huada Biological Science and Technology Co., Ltd (Guangzhou, China), and inserted into the lentiviral vector pHBLV-u6-puro (conserved in our laboratory). Human MM cell line RPMI-8226 (ATCC, Rockefeller, MD, U.S.A.) was maintained in the 1640 medium containing 10% fetal bovine serum (FBS) at 37°C with 5% CO2. Logarithmic growth phase cells were used for transfection using liposome 2000 (Invitrogen, Carlsbad, CA, U.S.A.). RPMI-8226 cells transfected with shN were considered as control. The transfection efficiency of shRNA-REGγ-1 and shRNA-REGγ-2 on RPMI-8226 cells was identified by Western blot. After 48 hours of transfection, the cells were used for further assay.
Cell proliferation assay
The proliferation rates of transfected RPMI-8226 cells were detected by a Cell Counting Kit 8 (CCK-8, Beyotime, Beijing, China). RPMI-8226 cells of different groups were seeded in 96-well plates (100 μl, 2000 cells each well) at 24, 48 and 72 hours post-transfection, and 10 μl CCK-8 solution was added into each well. After 4 hours of incubation, the optical density at 450 nm was detected by an ultraviolet spectrophotometer (Bio-Rad, Hercules, CA, U.S.A.).
Cell apoptosis assay
The apoptosis rates of transfected RPMI-8226 cells were detected by an annexin V-PI Kit (Beyotime, Beijing, China) on flow cytometer (BD, Franklin Lake, NJ, U.S.A.). RPMI-8226 cells of different groups were resuspended in 1 × binding buffer at 48 post-transfection, and stained with 5 μL annexin V-fluorescein isothiocyanate and 2.5 μL PI. After 10 minutes of incubation on ice in dark, the apoptosis rates of those cells were analyzed within 30 minutes using a flow cytometer.
Cell migration assay
The migration of transfected RPMI-8226 cells was detected using Transwell chambers (CORNING, Corning, NY, U.S.A.). RPMI-8226 cells of different groups were resuspended in a serum-free RPMI-1640 medium at 48 post-transfection, and added into the upper compartment of the chamber. RPMI-1640 containing 15% FBS was placed in the lower compartment of the chemotaxis chamber as a chemoattractant source. After incubated at 37°C for 4 hours, non-migrated cells on the upper surface of the filter were removed with cotton swabs, and those on the lower surface were fixed in 5% formaldehyde and stained with 1% hematoxylin. The stained cells were counted using a cell imaging system (EVOS f1, Thermofisher, Waltham, MA, U.S.A.) (5 randomly fields at 200× magnifications).
Quantitative real-time PCR
Total RNAs were extracted from RPMI-8226 cells in different groups using a TRizol reagent (Invitrogen), and reverse transcripted using a RevertAidTM First-Strand cDNA Synthesis Kit (Fermentas, Hangover, MD, U.S.A.). qRT-PCR was performed using a SYBR Green PCR kit (Applied Biosystem, Foster City, CA, U.S.A.) with special primers (IkBε, forward: 5′-CCTCAAAAGTGGGCTGAG-3′; reverse: 5′-CAAGGAACCACAGGAGAAGG-3′). GAPDH was used as an internal control (GAPDH, forward: 5′-CCA TCT ACG AGG GCT ACG C-3′; reverse: 5′-GGA AGG AGG GCT GGA ACA-3′). The PCR program included 94°C for 3 minutes, 30 cycles at 94°C for 1 minutes, 56°C for 1 minutes and 72°C for 2 minutes, and a final 2 minutes extension at 72°C. The relative expression of IkBε mRNA was normalized to GAPDH using the 2−ΔΔCt method [Citation17].
Western blot
Total proteins of RPMI-8226 cells in different groups were extracted by a lysis buffer. After 15 minutes of centrifugation (12 000 rpm) at 4°C, these proteins were separated by 10% SDS-polyacrylamide gel electrophoresis, and then transferred to a polyvinylidene fluoride membrane (Millipore, Billerica, MA, U.S.A.). The membrane was blocked with 5% skim milk for 1 hour, and special diluted primary antibodies (anti-p-p65, p65, matrix metalloproteinase 2 (MMP2), Bcl-xL and X-linked inhibitor of apoptosis protein (XIAP), 1:1000, cell signaling technology, U.S.A.; anti-IkBε and αREGγ, 1:1000, Abcam, Cambridge, England) were added, followed by incubation overnight at 4°C. After washed with TBST (tris-buffered saline + 0.1% tween 20) for three times, HRP-conjugated secondary antibody (1:5000, Abcam) was added and incubated for 1 hour at 25°C. The protein brands were visualized using a UV gel imager (FluorChem M, Globalebio, Beijing, China). Besides, cycloheximide (CHX) (1 mg/ml) was used to treat on transfected RPMI-8226 cells for 3 and 6 hours, and the half-life period of IkBε was detected by Western blot.
Statistical analyses
All experiments were performed with three replications. All data were expressed as mean ± SD. Statistical analysis was performed by SPSS version 17.0 (SPSS Inc., Chicago, IL, U.S.A.). Different groups were compared by t-test (two groups) or one-way ANOVA (more than two groups). A p-value less than 0.05 was significantly different.
Results
REGγ was positive expressed in myeloma cells of MM patients
The expression of REGγ in myeloma cells of MM patients was detected by Western blot. The results showed that the expression of REGγ was positive in myeloma cells from MM patients and negative in non-myeloma plasma cells from normal donors (positive rates: 89.29 vs. 0%, p < 0.01) (Fig. S1).
The growth of RPMI-8226 cells was inhibited by shRNA-REGγ
To evaluate the effects of REGγ knockdown on RPMI-8226 cells, shRNA-REGγ-1 and shRNA-REGγ-2 were constructed. Both shRNA-REGγ-1 and shRNA-REGγ-2 could inhibit the expression of REGγ effectively, and a significantly higher inhibitory rate was revealed on shRNA-REGγ-2 than on shRNA-REGγ-1 (83 vs. 75%). The proliferation and apoptosis of transfected RPMI-8226 cells were evaluated. As shown in (A), the proliferation of RPMI-8226 cells was significantly inhibited by shRNA-REGγ-1/shRNA-REGγ-2 in a time-dependent manner (p < 0.05). The inhibitory degree of shRNA-REGγ-2 on the proliferation of RPMI-8226 cells was stronger than that of shRNA-REGγ-1 ((A)). In addition, the apoptosis rates were significantly higher in RPMI-8226 cells transfected with shRNA-REGγ-1/shRNA-REGγ-2 than those of the control (p < 0.05, (B,C)). However, no significant differences were revealed on the apoptosis rates of RPMI-8226 cells transfected with either shRNA-REGγ-1 or shRNA-REGγ-2 ((B,C)).
Figure 1. The proliferation and apoptosis of RPMI-8226 cells transfected with shRNA-REGγ-1 and shRNA-REGγ-2 (n = 3). (A) The proliferation rates of RPMI-8226 cells transfected with shRNA-REGγ-1 and shRNA-REGγ-2 by CCK8. (B) The apoptosis of RPMI-8226 cells transfected with shRNA-REGγ-1 and shRNA-REGγ-2 by flow cytometry. (C) The apoptosis rates of RPMI-8226 cells transfected with shRNA-REGγ-1 and shRNA-REGγ-2. *Represent significantly different at p < 0.05 when compared with the control (RPMI-8226 cells transfected with shN).
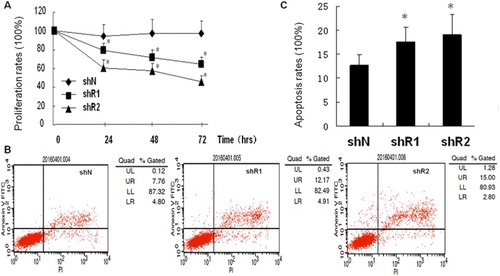
The migration of RPMI-8226 cells was inhibited by shRNA-REGγ
The migration of RPMI-8226 cells was further evaluated. As shown in , the number of migrated cells was significantly lower in cells transfected with shRNA-REGγ-1/shRNA-REGγ-2 when compared with the control (p < 0.01). However, no significant differences were revealed on the number of migrated cells between shRNA-REGγ-1 and shRNA-REGγ-2 group ().
Figure 2. The migration of RPMI-8226 cells transfected with shRNA-REGγ-1 and shRNA-REGγ-2 by transwell assay (n = 3). (A) Microscopic observation of migrated RPMI-8226 cells. (B) The number of migrated cells. **Represent significantly different at p < 0.01 when compared with the control (RPMI-8226 cells transfected with shN).
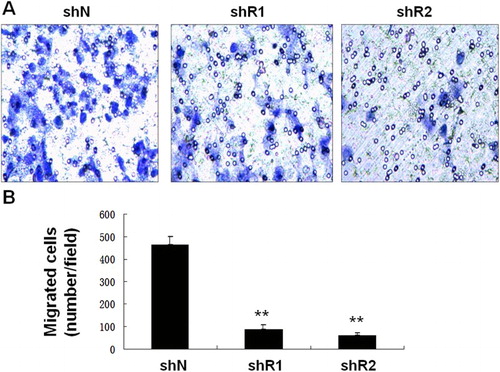
The NF-κB signal pathway was inhibited by shRNA-REGγ
In order to reveal the potential regulatory mechanisms of REGγ on RPMI-8226 cells, the expression of several key factors involved in NF-κB signal pathway was detected. Western blot showed that the expression of p-p65 was significantly reduced in RPMI-8226 cells transfected with shRNA-REGγ-1/shRNA-REGγ-2 ((A)). The expression of IkBε in protein level was significantly reduced in RPMI-8226 cells transfected with shRNA-REGγ-1/shRNA-REGγ-2, while the mRNA level of IkBε was not significantly changed ((A,B)). Meanwhile, the degradation of IkBε was significantly slower in RPMI-8226 cells transfected with shRNA-REGγ-1 than the control (longer half-life period) ((C)). Besides, the expression of MMP2, Bcl-xL and XIAP in RPMI-8226 cells was significantly inhibited by shRNA-REGγ-1/shRNA-REGγ-2 ((D)).
Figure 3. The potential regulatory mechanisms of REGγ on RPMI-8226 cells. (A) The expression of p-p65, p65, IkBε and αREGγ in RPMI-8226 cells transfected with shRNA-REGγ-1 and shRNA-REGγ-2 by Western blot (n = 3). (B) The expression of IkBε in RPMI-8226 cells transfected with shRNA-REGγ-1 and shRNA-REGγ-2 by RT-PCR. (C) The degradation of IkBε (half-life period) in RPMI-8226 cells transfected with shRNA-REGγ-1. (D) The expression of REGγ, MMP2, Bcl-xL and XIAP in RPMI-8226 cells transfected with shRNA-REGγ-1 and shRNA-REGγ-2 by Western blot.
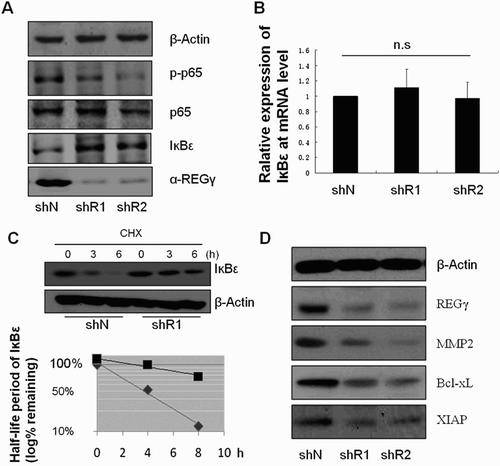
Discussion
REGγ plays an important role in the progression of cancers, while related research studies on its roles in MM are still limited. In this study, REGγ was positive expressed in myeloma cells. The knockdown of REGγ significantly inhibited the proliferation and migration of, and promoted, the apoptosis of RPMI-8226 cells. Moreover, the regulatory roles of REGγ on RPMI-8226 cells were closely related with the downregulation of NF-κB signal pathway.
As a member of the 11S family of proteasomal activators, REGγ is overexpressed in various cancers, such as breast cancer [Citation18], lung cancer [Citation19] and colorectal cancer [Citation20]. In this study, REGγ was positive expressed in myeloma cells of MM patients. Our finding is consistent with previous studies, and further illustrates the oncogenic role of REGγ in MM. At the cellular level, overexpression of REGγ enhances the proliferation and migration of lung cancer cells, and increases the cell numbers in the S+G2/M phase [Citation19]. On the contrary, silencing of REGγ inhibits the proliferation, migration and invasion of endometrial cancer cells [Citation21]. Consistent results were also revealed in this study. The inhibited proliferation and migration, and promoted apoptosis of RPMI-8226 cells suggest us that the knockdown of REGγ may be an effective strategy for the treatment of MM in clinic.
NF-κB is an important regulatory factor in the pathogenesis of MM [Citation14]. The activation of NF-κB promotes the growth and survival, and inhibits the apoptosis, of MM cells [Citation14,Citation22]. In this study, the expression of p-p65 and IkBε in RPMI-8226 cells was significantly inhibited by shRNA-REGγ, indicating that the knockdown of REGγ could inhibit NF-κB signal pathway in MM cells. In general, inactive NF-κB is present in the cytoplasm by interaction with inhibitory IκB [Citation23]. The inactivated NF-кB signaling inhibits the proliferation and survival, and induces the apoptosis, of MM cells [Citation24,Citation25,Citation26]. In line with these research works, our findings further indicated that the inhibitory effects of shRNA-REGγ on MM cells were closely related with the downregulation of NF-κB signal pathway. Meanwhile, the degradation of IκBϵ was slower in RPMI-8226 cells transfected with shRNA-REGγ-1 than the control. A previous study showed that the elevated expression of REGγ promotes a reciprocal regulatory loop with NFκB involved in ubiquitin-independent degradation of IκBϵ [Citation27]. Our finding is consistent with this research, and further implies that the regulatory role of REGγ on NF-κB signal pathway was attributed to the prolonged half-life period of IκBϵ.
As a key zinc-dependent enzyme involved in epithelial mesenchymal transition, MMP2 plays an important role in cancer metastasis [Citation28]. The expression of MMP2 was high in MM, and could be reduced by the anti-angiogenic treatment [Citation29,Citation30]. In this study, the expression of MMP2 was significantly inhibited by shRNA-REGγ, which indicates that the knockdown of REGγ may inhibit the migration of RPMI-8226 cells by downregulating MMP2. Besides, XIAP and Bcl-xL are two important apoptotic inhibitors in tumors [Citation31]. The decreased cell viability of MM cells induced by mangiferin is attributed to the decreased expression of XIAP and Bcl-xL via inhibition of NF-κB pathway [Citation32]. DETT inhibits NFκB transcription activity and downregulates NFκB-targeted genes, including Bcl-2, Bcl-xL and XIAP, resulting in MM cell apoptosis [Citation33]. In this study, the expression of Bcl-XL and XIAP was significantly inhibited by shRNA-REGγ. In line with the above research studies, we suspect that REGγ knockdown-induced downregulation of NFκB signal pathway may directly contribute to the reduced expression of Bcl-XL and XIAP.
In conclusion, knockdown of REGγ could inhibit the proliferation and migration, and promote the apoptosis of RPMI-8226 cells possible through downregulating NF-κB signal pathway. Meanwhile, this process may be closely related with the downregulation of MMP2, Bcl-xL and XIAP. However, this study was still limited in cell experiment. Further research works on the roles and related regulatory mechanisms of REGγ on MM on animal model are still needed.
Supplementary_material.zip
Download Zip (282.3 KB)Disclosure statement
No potential conflict of interest was reported by the authors.
ORCID
Hui-juan Shen http://orcid.org/0000-0002-3714-5348
Qing Zhang http://orcid.org/0000-0001-8785-6626
Additional information
Funding
References
- Fairfield H, Falank C, Avery L, et al. Multiple myeloma in the marrow: pathogenesis and treatments. Ann N Y Acad Sci. 2016;1364:32–51. doi: 10.1111/nyas.13038
- Bianchi G, Kumar S, Ghobrial IM, et al. Cell trafficking in multiple myeloma. Open J Hematol. 2011;48:S8–S.
- Dispenzieri A, Kyle RA. Multiple myeloma: clinical features and indications for therapy. Best Pract Res Clin Haematol. 2005;18:553–568. doi: 10.1016/j.beha.2005.01.008
- Mimura N, Hideshima T, Anderson KC. Novel therapeutic strategies for multiple myeloma. Exp Hematol. 2015;43:732–741. doi: 10.1016/j.exphem.2015.04.010
- Lambert KE, Huang H, Mythreye K, et al. The type III transforming growth factor-β receptor inhibits proliferation, migration, and adhesion in human myeloma cells. Mol Biol Cell. 2011;22:1463–1472. doi: 10.1091/mbc.e10-11-0877
- Zhang M, Gan L, Ren GS. REGγ is a strong candidate for the regulation of cell cycle, proliferation and the invasion by poorly differentiated thyroid carcinoma cells. Braz J Med Biol Res. 2012;45:459. doi: 10.1590/S0100-879X2012007500035
- Jing H, Long C, Yu Z, et al. REGγ is associated with multiple oncogenic pathways in human cancers. BMC Cancer. 2012;12:3971.
- Mao I, Liu J, Li X, et al. REGgamma, a proteasome activator and beyond? Cell Mol Life Sci. 2008;65:3971–3980. Epub 2008/08/06. doi: 10.1007/s00018-008-8291-z
- Chai F, Liang Y, Bi J, et al. High expression of REGγ is associated with metastasis and poor prognosis of patients with breast cancer. Int J Clin Exp Pathol. 2014;7:7834.
- Tian M, Xiaoyi W, Xiaotao L, et al. Proteasomes reactivator REG gamma enchances oncogenicity of MDA-MB-231 cell line via promoting cell proliferation and inhibiting apoptosis. Cell Mol Biol. 2009;55(Suppl):OL1121–OL1131.
- Chai F, Liang Y, Bi J, et al. REGgamma regulates ERalpha degradation via ubiquitin-proteasome pathway in breast cancer. Biochem Biophys Res Commun. 2015;456:534–540. Epub 2014/12/10. doi: 10.1016/j.bbrc.2014.11.124
- Ali A, Wang Z, Fu J, et al. Differential regulation of the REGgamma-proteasome pathway by p53/TGF-beta signalling and mutant p53 in cancer cells. Nat Commun. 2013;4:2667. Epub 2013/10/26. doi: 10.1038/ncomms3667
- D’Ignazio L, Batie M, Rocha S, et al. Hypoxia and inflammation in cancer, focus on HIF and NF-kappaB. Biomedicines. 2017;5:21–44. Epub 2017/05/26. doi: 10.3390/biomedicines5020021
- Matthews GM, de Matos Simoes R, Dhimolea E, et al. NF-kappaB dysregulation in multiple myeloma. Semin Cancer Biol. 2016;39:68–76. Epub 2016/08/22. doi: 10.1016/j.semcancer.2016.08.005
- Xiang Y, Remilywood ER, Oliveira V, et al. Monitoring a nuclear factor-κB signature of drug resistance in multiple myeloma. Mol Cell Proteomics. 2011;10:M110.005520. doi: 10.1074/mcp.M110.005520
- Chen WM. [The guidelines for the diagnosis and management of multiple myeloma in China (2015 revision): interpretation of the treatment of relapsing and refractory multiple myeloma]. Zhonghua Nei Ke Za Zhi. 2016;55:93–94.
- Livak KJ, Schmittgen TD. Analysis of relative gene expression data using real-time quantitative PCR and the 2(-delta C(T))method. Methods. 2001;Dec;25(4):402–408. doi: 10.1006/meth.2001.1262
- Chai F, Liang Y, Bi J, et al. High expression of REGgamma is associated with metastasis and poor prognosis of patients with breast cancer. Int J Clin Exp Pathol. 2014;7:7834–7843. Epub 2015/01/01.
- Qin Q, Guo FC, Luo ST, et al. [REGgamma promotes malignant behaviors of lung cancer cells]. Sichuan Da Xue Xue Bao Yi Xue Ban. 2014;45:304–308. Epub 2014/04/23.
- Chen D, Yang X, Huang L, et al. The expression and clinical significance of PA28 gamma in colorectal cancer. J Investig Med. 2013;61:1192–1196. Epub 2013/10/12. doi: 10.2310/JIM.0000000000000001
- Wang H, Bao W, Jiang F, et al. Mutant p53 (p53-R248Q) functions as an oncogene in promoting endometrial cancer by up-regulating REGgamma. Cancer Lett. 2015;360:269–279. Epub 2015/02/24. doi: 10.1016/j.canlet.2015.02.028
- Gilmore TD. Multiple myeloma: lusting for NF-κB. Cancer Cell. 2007;12:95–97. doi: 10.1016/j.ccr.2007.07.010
- Napetschnig J, Wu H. Molecular basis of NF-κB signaling. Ann Rev Biophys. 2013;42:443–468. doi: 10.1146/annurev-biophys-083012-130338
- Xu J, Sun HY, Xiao FJ, et al. SENP1 inhibition induces apoptosis and growth arrest of multiple myeloma cells through modulation of NF-kappaB signaling. Biochem Biophys Res Commun. 2015;460:409–415. Epub 2015/03/21. doi: 10.1016/j.bbrc.2015.03.047
- Li Z, Yang Z, Peng X, et al. Nuclear factor-kappaB is involved in the protocadherin-10-mediated pro-apoptotic effect in multiple myeloma. Mol Med Rep. 2014;10:832–838. Epub 2014/06/04. doi: 10.3892/mmr.2014.2285
- Hideshima T, Neri P, Tassone P, et al. MLN120B, a novel IkappaB kinase beta inhibitor, blocks multiple myeloma cell growth in vitro and in vivo. Clin Cancer Res. 2006;12:5887–5894. Epub 2006/10/06. doi: 10.1158/1078-0432.CCR-05-2501
- Xu J, Lei Z, Lei J, et al. The REGγ-proteasome forms a regulatory circuit with IκBϵ and NFκB in experimental colitis. Nat Commun. 2016;7:10761. doi: 10.1038/ncomms10761
- Choi YA, Lim HK, Kim JR, et al. Group IB secretory phospholipase A2 promotes matrix metalloproteinase-2-mediated cell migration via the phosphatidylinositol 3-kinase and Akt pathway. J Biol Chem. 2004;279:36579–6585. Epub 2004/06/29. doi: 10.1074/jbc.M314235200
- Bolkun L, Lemancewicz D, Sobolewski K, et al. The evaluation of angiogenesis and matrix metalloproteinase-2 secretion in bone marrow of multiple myeloma patients before and after the treatment. Adv Med Sci. 2013;58:118–125. Epub 2013/01/22. doi: 10.2478/v10039-012-0048-0
- Urbaniak-Kujda D, Kapelko-Slowik K, Prajs I, et al. Increased expression of metalloproteinase-2 and -9 (MMP-2, MMP-9), tissue inhibitor of metalloproteinase-1 and -2 (TIMP-1, TIMP-2), and EMMPRIN (CD147) in multiple myeloma. Hematology. 2016;21:26–33. Epub 2015/08/14. doi: 10.1179/1607845415Y.0000000043
- Kunze D, Kraemer K, Erdmann K, et al. Simultaneous siRNA-mediated knockdown of antiapoptotic BCL2, Bcl-xL, XIAP and survivin in bladder cancer cells. Int J Oncol. 2012;41:1271–1277. Epub 2012/07/17.
- Takeda T, Tsubaki M, Kino T, et al. Mangiferin enhances the sensitivity of human multiple myeloma cells to anticancer drugs through suppression of the nuclear factor kappaB pathway. Int J Oncol. 2016;48:2704–2712. Epub 2016/04/02. doi: 10.3892/ijo.2016.3470
- Chen G, Han K, Xu X, et al. An anti-leishmanial thiadiazine agent induces multiple myeloma cell apoptosis by suppressing the nuclear factor kappaB signalling pathway. Br J Cancer. 2014;110:63–70. Epub 2013/11/16. doi: 10.1038/bjc.2013.711