Abstract
Positive social interactions may protect against stress. This study investigated the beneficial effects of pairing with a social partner on behaviors and neuroendocrine function in response to chronic mild stress (CMS) in 13 prairie vole pairs. Following 5 days of social bonding, male and female prairie voles were exposed to 10 days of CMS (mild, unpredictable stressors of varying durations, for instance, strobe light, white noise, and damp bedding), housed with either the social partner (paired group) or individually (isolated group). Active and passive behavioral responses to the forced swim test (FST) and tail-suspension test (TST), and plasma concentrations of adrenocorticotropic hormone (ACTH) and corticosterone, were measured in all prairie voles following the CMS period. Both female and male prairie voles housed with a social partner displayed lower durations of passive behavioral responses (immobility, a maladaptive behavioral response) in the FST (mean ± SEM; females: 17.3 ± 5.4 s; males: 9.3 ± 4.6 s) and TST (females: 56.8 ± 16.4 s; males: 40.2 ± 11.3 s), versus both sexes housed individually (females, FST: 98.6 ± 12.9 s; females, TST: 155.1 ± 19.3 s; males, FST: 92.4 ± 14.1 s; males, TST: 158.9 ± 22.0 s). Female (but not male) prairie voles displayed attenuated plasma stress hormones when housed with a male partner (ACTH: 945 ± 24.7 pg/ml; corticosterone: 624 ± 139.5 ng/ml), versus females housed individually (ACTH: 1100 ± 23.2 pg/ml; corticosterone: 1064 ± 121.7 ng/ml). These results may inform understanding of the benefits of social interactions on stress resilience.
Lay Summary: Social stress can lead to depression. The study of social bonding and stress using an animal model will inform understanding of the protective effects of social bonds. This study showed that social bonding in a rodent model can protect against behavioral responses to stress, and may also be protective against the elevation of stress hormones. This study provides evidence that bonding and social support are valuable for protecting against stress in humans.
Introduction
Depression is one of the leading health issues in the world today (Mathers & Loncar, Citation2005), and is hypothesized to be influenced by exposure to, and an inability to adequately cope with, psychosocial stressors (Hammen, Citation2005; Sapolsky, Citation2015; Tafet & Bernardini, Citation2003). In particular, stress of a social variety, such as social isolation and feelings of loneliness, may induce depressive symptomology, as well as alter neuroendocrine stress response activation and contribute to physiological dysfunction associated with depression (Cacioppo et al., Citation2015; Ramsay et al., Citation2008; Steptoe et al., Citation2013). Conversely, social support (in humans) and having a partner present (in animal models) can ameliorate many of the negative physiological outcomes of stress exposure (Burkett et al., Citation2016; Gobrogge & Wang, Citation2015; Heinrichs et al., Citation2003; Kiyokawa et al., Citation2004; Steptoe et al., Citation2013; Sun et al., Citation2014; Thorsteinsson et al., Citation1998).
Several studies with animal models have further demonstrated the beneficial effects of social interactions on behavior and physiological health. For instance, when fear-conditioned rats were exposed to the box in which they had previously been shocked, but were paired with a partner, they showed attenuated stress-induced hyperthermia, freezing behavior, and neural activation in the hypothalamic paraventricular nucleus in response to the fearful context (Kiyokawa et al., Citation2004). Similarly, following a period of social defeat stress, male rats that were housed with a female partner displayed lower levels of depression-like behaviors (hypothesized to be represented by a reduction in sucrose consumption) and improved cardiac function and structure versus those that were housed alone (Carnevali et al., Citation2012). This previous research provides evidence for the utility of animal models for the study of behavioral and physiological responses to social stressors.
In addition to studies with rats, previous research with prairie voles (Microtus ochrogaster) indicates that positive social interactions have a protective influence on both behavior and physiology. Prairie voles are rodents that display several features of social monogamy, such as bi-parental care of offspring, living in extended family groups, and the formation of strong male–female social bonds that are not often evident in other rodents such as rats, mice, or comparatively less monogamous vole species (Carter et al., Citation1995; Young et al., Citation2001; Young & Wang, Citation2004). Prairie voles are especially susceptible to social environmental stressors in a manner similar to humans, and previous research indicates that social bonds in this species can protect against behavioral and physiological consequences of stress. For example, social bonding in prairie voles attenuates several responses to stressors, including: (a) reducing responses that have been hypothesized to be operational indices of depression-like behaviors (such as reduced preference for a palatable sucrose solution and increased helpless behavior in a forced swimming task), (b) reducing hypothalamo-pituitary-adrenal (HPA) axis reactivity, (c) preventing or reversing autonomic imbalance, and (d) altering central nervous system production of hormones and peptides (Bosch et al., Citation2009; Grippo et al., Citation2007b; McNeal et al., Citation2014; Smith & Wang, Citation2014; Sun et al., Citation2014).
Given previous findings indicating a protective role of social interactions on responses to stress in humans and various animal species, the current study used the prairie vole model to determine whether altered behaviors and neuroendocrine activation associated with exposure to chronic stressors are attenuated when experiencing these stressors with a pair-bonded social partner. One tool for investigating long-term stress in rodents is the chronic mild stress (CMS) paradigm, which involves extended exposure to a series of unpredictable mild stressors, such as a tilted cage, strobe light, white noise, or damp bedding. CMS has been hypothesized to induce depression-like behaviors in rodents, such as anhedonia (measured via a reduction in sucrose preference), decreased active coping behaviors (measured via a reduction in active behaviors in a forced swim test [FST]), reduced exploration, and altered physical activity; and has been used previously in rodent studies, including prairie voles, that have focused on depression-relevant research questions (Bielajew et al., Citation2003; Grippo et al., Citation2015; Tannenbaum et al., Citation2002; Willner, Citation2005). Therefore, the goal of the present study was to specifically investigate the potential protective effects of social pairing on behavioral and neuroendocrine responses to CMS in prairie voles. We hypothesized that pairing with a previously bonded partner of the opposite sex would be associated with attenuated behavioral stress-coping responses and plasma concentrations of adrenocorticotropic hormone (ACTH) and corticosterone following exposure to CMS, relative to receiving CMS without the presence of a social partner.
Methods
Animals
Subjects were 26 adult prairie voles (13 male, 13 female), descendants of a wild stock caught near Champaign, Illinois. All prairie voles were bred in-house. Prairie voles remained with the family group until post-natal day 21, at which time they were removed from the family group and housed in same-sex sibling pairs until the commencement of experimentation. For all procedures described here, only one animal from each sibling pair was used. The experiments commenced when all prairie voles reached a sexually mature age; however, all prairie voles were sexually naïve.
Upon commencement of the experiments, males had a mean (± standard error of the mean; SEM) age of 77 ± 3 days, and a body weight of 41 ± 2 g. Females had a mean (± SEM) age of 86 ± 4 days and body weight of 34 ± 1 g. All prairie voles were maintained on a 14 h/10 h light/dark cycle (lights on at 06:30 h and off at 20:30 h), with a mean ± SEM ambient temperature of 25 ± 2 °C and relative humidity of 40 ± 5%. Prairie voles were allowed food (Purina rabbit chow) and water ad libitum, unless otherwise noted. All procedures were conducted according to the National Institutes of Health’s Guide for the Care and Use of Laboratory Animals and approved by the local University Institutional Animal Care and Use Committees. A general timeline of all procedures is displayed in .
Table 1. Timeline of procedures used in the present study.
Initial social bonding
The initial social bonding period consisted of pairing unrelated male and female prairie voles. Each experimental prairie vole was removed from its sibling and paired with an unrelated prairie vole of the opposite sex of similar age and body weight in a clean, neutral cage for 5 days. This time period has been shown to be sufficient for male and female prairie voles to form a social bond (Bosch et al., Citation2009; McNeal et al., Citation2014). Previous research also indicates that prairie voles may mate during this 5-day social bonding period, given that female prairie voles are induced ovulators (females need physical contact with a male to be induced into an estrous cycle) (McNeal et al., Citation2014).
Social isolation/continued pairing period
Following the 5-day social bonding period, approximately half of the male–female pairs (n = 7 males, n = 7 females) were separated into individual cages (without visual, auditory, or olfactory cues from the partner) for 5 additional days; while the remainder of the male–female pairs remained paired during this period (n = 6 males, n = 6 females). This time period is consistent with previous studies showing that 5 days of social isolation from an opposite-sex partner induces alterations in behavior and increased stressor reactivity in prairie voles, compared to social pairing (Bosch et al., Citation2009).
Chronic mild stress
Following the 5-day social isolation/continued pairing period, all prairie voles were exposed to 10 days of CMS while either in the presence (paired group) or in the absence (isolated group) of the social partner. Specifically, all males and females were exposed to CMS; those in the isolated group were exposed to the stressors while they were housed individually (without visual, auditory, or olfactory cues from their respective previous partners), whereas those in the paired group were exposed to the stressors in the same cage as their respective partners. The CMS procedure was a variation of methods described previously (Willner, Citation2005). The prairie voles were exposed to a series of mild stressors on an unpredictable schedule for varying durations (), including: (a) 2 periods of continuous overnight illumination (10 h each); (b) 1 period of water deprivation (6 h) followed by exposure to an empty water bottle (1 h); (c) 2 periods of stroboscopic illumination in a dark room (300 flashes/min; 3 h each); (d) 2 periods of background white noise (90 dB; 2 and 3 h); (e) 3 periods of 40° cage tilt (7 h, 7.5 h, and 16.5 h); (f) 2 periods of damp bedding (100 ml water; 4 and 5 h); and (g) 2 periods of a foreign object in the cage [brick, 15 × 8 × 6cm; 4 and 6 h; this stressor was used in lieu of the paired housing stressor, which is typical in CMS paradigms in other rodents (Willner, Citation2005) but would interfere with the current social housing manipulation].
Tail-suspension test
A 5-min tail-suspension test (TST) was used as an index of a passive (i.e. maladaptive) behavioral response to a short-term stressor in all prairie voles (paired and isolated). The procedure was adapted from Steru et al. (Citation1985), and has been used to measure stress-coping behaviors in prairie voles (Bosch et al., Citation2009). Following the final day of CMS, each prairie vole was suspended by its tail using adhesive tape attached to a metal bar (5 mm diameter), in the middle of an opaque plastic box (32 × 28 × 29cm) approximately 25 cm above the apparatus floor. Care was taken to ensure that the tail was appropriately affixed to the bar without compromising the tail itself during attachment, the 5-min test, or detachment, by attaching the tape to the middle of the tail (equidistant between the tip and base of the tail; the tail is approximately 3 cm in length). The apparatus was cleaned thoroughly prior to testing each prairie vole. The prairie vole was returned to its home cage (paired or isolated) immediately following the test. Exposure to the TST occurred in all male–female pairs (whether in the paired or isolated group) at the same time in separate locations without visual, auditory, or olfactory cues, to ensure consistency between the groups.
Durations of behaviors during the TST were recorded with a digital video camera, and scored manually by three trained, experiment-blinded coders. Behaviors were defined as: (a) active coping behaviors, involving active movements such as contortions of the body and/or flailing of the limbs; or (b) immobility, involving no movements beside those required for respiration; these are mutually exclusive and exhaustive categories. Active coping behaviors were defined as adaptive responses to the stressor, whereas immobility was defined as a passive (i.e. maladaptive) behavioral response, and has been hypothesized to be related to a depressive phenotype according to previous tests of validity and reliability (Bosch et al., Citation2009; Cryan et al., Citation2005a). Durations of each behavioral category were averaged among the three coders.
Forced swim test
Investigation of swimming behavior in the FST was used as an additional index of a passive (i.e. maladaptive) behavioral response to a short-term stressor. Twenty-four hours following the TST, all prairie voles were exposed to a 5-min period of forced swimming using procedures described previously in rodents (Cryan et al., Citation2005b). A clear, cylindrical Plexiglas tank (height 46 cm; diameter 20 cm) was filled to a depth of 18 cm with tap water at room temperature (25–26 °C). Each vole was placed individually into the tank for 5 min. The tank was cleaned thoroughly and filled with clean water prior to testing each vole. The prairie vole was returned to its home cage (paired or isolated) immediately following the swim period, and was given a heat lamp for 15 min. Exposure to the FST occurred in all male–female pairs (whether in the paired or isolated group) at the same time in separate locations, without visual, auditory, or olfactory cues.
Durations of behaviors during the swim test were recorded using a digital video camera, and scored manually by three trained, experiment-blinded coders. Behaviors were defined as: (a) swimming, involving movements of the fore- and hind-limbs without breaking the surface of the water; (b) struggling, involving fore-limbs breaking the surface of water; (c) climbing, involving attempts to climb the walls of the tank; and (d) immobility, involving no limb or body movements (passive floating) or using limbs solely to remain afloat without corresponding trunk movements; these are mutually exclusive and exhaustive categories. Struggling, climbing, and swimming were summed to provide one index of active coping behaviors, and were defined as adaptive behavioral responses; immobility was defined as a passive (i.e. maladaptive) behavioral response, and has been hypothesized to be related to a depressive phenotype according to previous tests of validity and reliability (Bielajew et al., Citation2003; Cryan et al., Citation2005b). Durations of each behavioral category were averaged among the three coders.
Collection of blood
Seventy-two hours following the FST, all prairie voles were anesthetized with a combination of ketamine and xylazine (ketamine, 67 mg/kg, NLS Animal Health, Owings Mills, MD; xylazine, 13.33 mg/kg, NLS Animal Health; a ratio of 5:1 ketamine:xylazine injected subcutaneously at a volume of approximately 0.07 ml) during the light period (2.5–4.5 h following light onset, from 09:00 h to 11:00 h). Blood was collected from all male–female pairs (whether in the paired or isolated group) at the same time in separate locations, without visual, auditory, or olfactory cues. Blood collection in all prairie voles was conducted across a total period not exceeding 2 h, to reduce variability owing to circadian rhythmicity of hormone levels. Each prairie vole was anesthetized within 1 min of being removed from the housing room. Blood was sampled within 2 min of the anesthetic injection, from the periorbital sinus via a heparinized capillary tube, and collected during a period not exceeding 1.5 min (which resulted in approximately 0.5–1 ml of blood from each animal), to avoid possible rapid fluctuations in hormone levels. Blood was placed immediately on ice, centrifuged at 4 °C, at 1100 g, for 15 min to obtain plasma. Plasma aliquots were stored at −80 °C.
Assessment of pregnancy
Each prairie vole was euthanized via cervical dislocation followed by decapitation immediately after the blood collection. All females were assessed for pregnancy by visual inspection of the uterine horns. A prairie vole was determined to be pregnant if embryos were evident.
Analysis of circulating hormones
Plasma concentrations of ACTH and corticosterone were measured using commercial enzyme-linked immunosorbent assay kits, according to the kit instructions (ACTH, EK-001-21, Phoenix Pharmaceuticals, Burlingame, CA; corticosterone, Enzo Life Sciences, ADI-900-097, Farmingdale, NY). Plasma was diluted in assay buffer as necessary (1:7 for ACTH; 1:500 for corticosterone) to yield results reliably within the linear portion of the standard curve. The minimum detection limits are 80 pg/ml for ACTH and 0.027 ng/ml for corticosterone. Inter- and intra-assay coefficients of variation are <5% for both ACTH and corticosterone. Cross-reactivity with other steroids or peptides is <0.1% for ACTH and <1.7% for corticosterone.
Data analyses
Data are presented as means ± SEM for all analyses and figures. A value of p < .05 was considered to be statistically significant. For behavioral and plasma data, independent-groups analyses of variance (ANOVA) were used for comparisons between housing (paired or isolated) and sex, followed by Student’s t-tests with a Bonferroni correction for multiple comparisons. Results of t-tests indicate statistically significant effects after the Bonferroni correction was applied. A Fisher’s exact test was used to test for a difference in pregnancy rate between housing groups (paired or isolated).
Because the active and passive behavioral categories in the TST and FST are mutually exclusive and exhaustive for each test, the duration of passive/maladaptive response of immobility during each 5-min test period is the dependent measure that is presented in the results and figures; the remainder of the 5 min is comprised of the active/adaptive responses.
Results
Tail-suspension test responses
A main effect of housing was observed for immobility duration (i.e. passive, maladaptive behavioral response) during the TST [F(1,21) = 35.11, p < .001; ]. The main effect of sex and the housing by sex interaction were not statistically significant (main effect of sex, p > .6; housing by sex interaction, p > .99); no follow-up tests were conducted.
Figure 2. Duration of immobility (passive/maladaptive behavioral response) during a 5-min tail-suspension test (Panel A) and a 5-min forced swim test (Panel B). Tests were performed following 10 days of chronic mild stress. Groups are isolated male (n = 7), isolated female (n = 7), paired male (n = 6), and paired female prairie voles (n = 6). Data are mean ± standard error of the mean, SEM. *p < .05 versus paired groups (main effect of housing, mixed-design ANOVA). Note: because active and passive behavioral responses were mutually exclusive and exhaustive behavioral categories, active coping behaviors comprised the remainder of the 5-min period for each test.
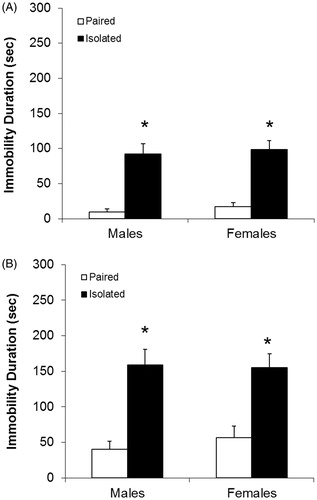
Forced swim test responses
A main effect of housing was observed for immobility duration (i.e. passive, maladaptive behavioral response) during the FST [F(1,21) = 50.43, p < .001; ]. The main effect of sex and the housing by sex interaction were not statistically significant (main effect of sex, p > .6; housing by sex interaction, p > .5); no follow-up tests were conducted.
Plasma adrenocorticotropic hormone concentration
A main effect of housing [F(1,21) = 9.74, p < .005], sex [F(1,21) = 9.45, p < .006], and housing by sex interaction [F(1,21) = 25.14, p < .001] were observed for plasma ACTH concentrations (). Isolated females displayed significantly higher concentrations of ACTH versus isolated males [t(12) = 5.93, p < .001], paired males [t(11) = 4.75, p < .001], and paired females [t(11) = 4.56, p < .001]. There were no significant differences in ACTH concentrations among isolated males, paired males, or paired females (isolated males vs. paired males, p > .06; isolated males vs. paired females, p > .5; paired males vs. paired females, p > .1).
Figure 3. Plasma adrenocorticotropic hormone (ACTH; Panel A) and corticosterone concentrations (Panel B). Samples were collected following 10 days of chronic mild stress. Groups are isolated male (n = 7), isolated female (n = 7), paired male (n = 6), and paired female prairie voles (n = 6). Values are mean ± standard error of the mean, SEM. *p < .05 versus both paired groups; ^p < .05 versus isolated male group (main effect of sex, housing, and housing by sex interaction, mixed-design ANOVA; t-tests with a Bonferroni correction).
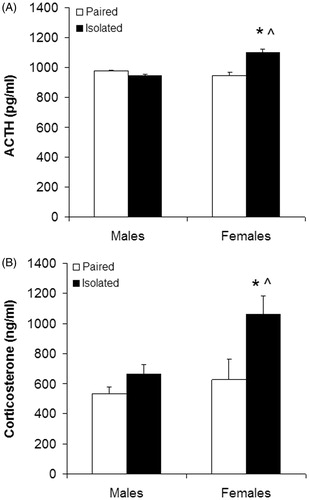
Plasma corticosterone concentration
A main effect of housing [F(1,21) = 8.22, p < .009], sex [F(1,21) = 6.32, p < .020], and a housing by sex interaction [F(1,21) = 11.73, p < .002] were observed for plasma corticosterone concentrations (). Isolated females displayed significantly higher concentrations of corticosterone versus isolated males [t(12) = 2.90, p < .007], paired males [t(11) = 3.83, p < .001], and paired females [t(11) = 2.39, p < .018]. There were no significant differences in corticosterone concentrations among isolated males, paired males, or paired females (isolated males vs. paired males, p > .08; isolated males vs. paired females, p > .4; paired males vs. paired females, p > .27).
Pregnancy status of females
Analysis of pregnancy status at the end of the study indicated that a total of 5/6 (83%) of the female prairie voles in the paired group were pregnant, compared to a total of 2/7 (29%) in the isolated group. These values were slightly, but not significantly, different according to a Fisher’s exact test (p > .10); however this lack of a statistically significant difference is likely due to the small sample sizes in the current study.
Discussion
The present study used a prairie vole model to specifically investigate the potential protective role of social pairing on behavioral and neuroendocrine responses to CMS. The findings indicate that stress-coping behavioral responses to CMS are attenuated in both male and female prairie voles by pairing with a social partner of the opposite sex (vs. social isolation), as indicated by a reduction in passive behavioral responses to both the TST and the FST. Further, the pituitary-adrenal axis responses to CMS are protected by social pairing in females, but not males, as indicated by a reduction in plasma concentrations of ACTH and corticosterone in females that were paired with male partners (vs. females that were isolated from their respective male partners). These findings contribute to understanding of the protective effects of social bonding on behavioral and physiological responses to CMS.
Specifically, the current study employed the CMS paradigm to investigate whether social pairing in a socially monogamous rodent species can serve a protective role against stress-coping behaviors and associated neuroendocrine activation. The CMS paradigm is hypothesized to be a useful rodent model associated with depression, designed to provide a realistic simulation of the stressors of daily life via chronic exposure to a variety of rather minor environmental manipulations (Willner, Citation2005). This paradigm has been shown to reliably induce behavioral changes that are hypothesized to be relevant to a depressive phenotype, and several correlated physiological changes, in other rodent species (Bouzinova et al., Citation2012; Li et al., Citation2008; Willner, Citation2005). Exposure to CMS also produced increases in helpless behavior in a FST in both paired and isolated prairie voles (compared to unstressed controls in each housing condition), and further exacerbated these behavioral consequences in socially isolated prairie voles (Grippo et al., Citation2015). The results of the present study provide additional insight into the interactions of the social environment and CMS, by demonstrating that both male and female prairie voles that experienced CMS in the presence of an opposite-sex socially bonded partner displayed lower levels of passive behavioral responses in the TST and FST, compared to prairie voles that experienced CMS in the absence of a previous social partner. Given that an inability to cope with chronic stressors appears to be a precipitating factor in the development of depression-relevant behaviors (Gobinath et al., Citation2014; Hammen, Citation2005), the results of the present study may provide insight into the importance of emphasizing stress-coping strategies and social support for protecting against depression in both men and women.
In addition to protecting against behavioral disturbances in the TST and FST, social pairing in female prairie voles also attenuated the circulating concentrations of ACTH and corticosterone following CMS, compared to social isolation. There was a significant effect of sex on circulating stress hormones, mediated by the elevated levels in isolated female prairie voles relative to paired females, paired males, and isolated males. Considering the effects of CMS generally in prairie voles, this observed increase in circulating stress hormone concentrations only in females is consistent with research in rats demonstrating that, although both males and females exposed to CMS displayed behavioral disruptions (vs. unstressed controls), an increase in corticosterone level was observed only in female rats (Dalla et al., Citation2005). By contrast, other studies have observed increases in HPA axis reactivity to CMS in male rats (females were not specifically studied) (Bielajew et al., Citation2002; Grippo et al., Citation2005a). However, there is also evidence that CMS does not produce elevations in ACTH or corticosterone secretion in either male or female rats (Grippo et al., Citation2005b). It has been suggested that genetic factors, individual differences in stress susceptibility, and/or the duration of CMS may mediate some of the inconsistencies in sex differences in neuroendocrine reactivity to CMS, at least in rats (Bielajew et al., Citation2002; Dalla et al., Citation2005; Grippo et al., Citation2005b). In addition to these hypotheses, it is also possible that the duration and timing of HPA axis reactivity differ between male and female prairie voles, such that the collection of blood following the end of the CMS paradigm allowed for the detection of ACTH and corticosterone changes in females, but not in males. Further investigation of the duration of HPA axis elevations and (potential) recovery over time in response to CMS is warranted.
Considering the effects of CMS more specifically in combination with social bonding in prairie voles, the current observation that only females were protected against pituitary-adrenal axis activation following CMS is consistent with previous findings showing sex differences in HPA axis reactivity in prairie voles exposed to a combination of different social conditions and additional stressors. For instance, although no differences in circulating ACTH and corticosterone were observed in prairie voles of either sex following only social pairing with (vs. social isolation from) a same-sex sibling, when paired or isolated voles were exposed to a novel resident-intruder stressor, the short-term ACTH and corticosterone responses were buffered by social pairing only in females, but not in males (Grippo et al., Citation2007a). However, other findings indicate a lack of sex differences in the protective effects of social pairing on basal and acute stressor-induced HPA axis responses in prairie voles. Both male and female prairie voles paired with an opposite-sex partner (vs. isolated males and females) displayed attenuated short-term ACTH and corticosterone elevations following a novel acute swim stressor (McNeal et al., Citation2014). Further, lower levels of basal corticotropin-releasing factor and corticosterone were observed in male prairie voles paired with a socially bonded female partner versus those that were housed in isolation (females were not specifically investigated) (Bosch et al., Citation2009; Sun et al., Citation2014). Taken together, these previous findings indicate that HPA axis responses may differ by sex in prairie voles as a function of the type of social relationship (e.g. sibling pairing vs. opposite-sex partner pairing), as well as presence or absence of additional stressors (e.g. social pairing alone vs. social pairing coupled with either an acute stressor or a series of chronic stressors).
When considering previous evidence from studies that focus specifically on CMS, along with those that focus specifically on social bonding, the sex difference in HPA axis reactivity to CMS in the present study may have resulted from a particular combination of behavioral and endocrine benefits of social bonding. For instance, Dalla et al. (Citation2005) suggest that sex differences in the HPA responses to CMS are associated with differential actions of sex hormones in females versus males, as well as disruption of the hypothalamic-pituitary-gonadal axis specifically in females. Consistent with this hypothesis, CMS produces disruptions in estrous cycling in female rats (Dalla et al., Citation2005; Grippo et al., Citation2005b). Behavioral and physiological changes in females as a function of CMS may be associated with cyclical changes in the secretion of estrogen and progesterone, whereas the lack of physiological changes in males may indicate that either CMS does not alter testicular function or that male behavior is less dependent on testicular hormones (Dalla et al., Citation2005). The present study design does not allow for specific conclusions regarding the role of sex hormones in behavioral and HPA responses to CMS. However, paired females were more likely than isolated females to be pregnant at the end of the protocol, which may provide indirect support for the hypothesis of Dalla et al. (Citation2005). For instance, while both groups were paired first for 5 days during which time mating may have occurred, the paired group had an additional 15 days for possible fertilization to occur. By contrast, a combination of social isolation and CMS may have disrupted the estrous cycle in female prairie voles, preventing fertilization in some isolated females. This is a limitation of the current study. It is possible that the lower levels of ACTH and corticosterone observed in paired females resulted from pregnancy, rather than from social contact with a male partner. Given the small sample sizes in the current study, it is not possible to investigate this hypothesis by statistically controlling for pregnancy; however, some research in other mammalian systems has found that the sympathetic nervous system and HPA axis responses to stressors are attenuated from mid-pregnancy through lactation (Slattery & Neumann, Citation2008). In contrast, other research indicates that basal circulating levels of ACTH, cortisol, and catecholamines are elevated during pregnancy (de Weerth & Buitelaar, Citation2005). Therefore, future studies should investigate the interactions of the HPA/hypothalamic-pituitary-gonadal axes, as well as the specific influence of pregnancy on responses to CMS in prairie voles.
To further understand the sex differences observed in HPA axis reactivity to CMS in the present study, future studies may also consider specifically investigating the role of oxytocin. Not only do concentrations of this neuropeptide shift during pregnancy (Slattery & Neumann, Citation2008), but also oxytocin has been shown to play an important role in mediating physiological responses to social stressors and social bonding (Gobrogge & Wang, Citation2015; Hurlemann & Scheele, Citation2016). For example, oxytocin has been shown to interact with positive social interactions both in humans (Heinrichs et al., Citation2003) and prairie voles (Burkett et al., Citation2016; Smith & Wang, Citation2014) to facilitate adaptive responses to stress. Therefore, alterations in central and/or peripheral oxytocin function may mediate responses to CMS in a sex-dependent manner.
In conclusion, social pairing with an opposite-sex partner attenuates the behavioral effects of CMS in both male and female prairie voles, and may attenuate HPA axis reactivity to CMS in females (but not males). These findings highlight the importance of effective coping strategies, including social support, for dealing with mild daily stressors in humans. These data also shed light on the influence of social bonding on behavioral and physiological responses to stressors in males and females, and may provide insight into previously reported gender differences in human stress reactivity in the context of social experiences and depression (Kiecolt-Glaser & Newton, Citation2001; Kim et al., Citation2013; Rivera-Torres et al., Citation2013). The neurobiological and behavioral mechanisms underlying the beneficial effects of social bonding, and the mechanistic underpinnings of possible sex differences in response to stress and social support, deserve further attention. Future studies involving the use of translational animal models can inform the understanding of mechanisms through which social experiences have protective benefits for humans.
Disclosure statement
Aside from providing funding, the funding sources had no further role in the study design, data collection, analysis and interpretation of data, writing of the report, or decision to submit the paper for publication. The authors have no additional conflicts of interest to declare.
Additional information
Funding
References
- Bielajew C, Konkle AT, Kentner AC, Baker SL, Stewart A, Hutchins AA, Barbagallo LS, Fouriezos G. (2003). Strain and gender specific effects in the forced swim test: effects of previous stress exposure. Stress 6:269–80.
- Bielajew C, Konkle ATM, Merali Z. (2002). The effects of chronic mild stress on male Sprague-Dawley and Long Evans rats: I. biochemical and physiological analyses. Behav Brain Res 136:583–92.
- Bosch OJ, Nair HP, Ahern TH, Neumann ID, Young LJ. (2009). The CRF system mediates increased passive stress-coping behavior following the loss of a bonded partner in a monogamous rodent. Neuropsychopharmacology 34:1406–15.
- Bouzinova EV, Moller-Nielsen N, Boedtkjer DB, Broegger T, Wiborg O, Aalkjaer C, Matchkov VV. (2012). Chronic mild stress-induced depression-like symptoms in rats and abnormalities in catecholamine uptake in small arteries. Psychosom Med 74:278–87.
- Burkett JP, Andari E, Johnson ZV, Curry DC, de Waal FBM, Young LJ. (2016). Oxytocin-dependent consolation behavior in rodents. Science 351:375–8.
- Cacioppo S, Grippo AJ, London S, Goossens L, Cacioppo JT. (2015). Loneliness: clinical import and interventions. Perspect Psychol Sci 10:238–49.
- Carnevali L, Mastorci F, Graiani G, Razzoli M, Trombini M, Pico-Alfonso MA, Arban R, et al. (2012). Social defeat and isolation induce clear signs of a depression-like state, but modest cardiac alterations in wild-type rats. Physiol Behav 106:143–50.
- Carter CS, DeVries AC, Getz LL. (1995). Physiological substrates of mammalian monogamy: the prairie vole model. Neurosci Biobehav Rev 19:303–14.
- Cryan JF, Mombereau C, Vassout A. (2005a). The tail suspension test as a model for assessing antidepressant activity: review of pharmacological and genetic studies in mice. Neurosci Biobehav Rev 29:571–625.
- Cryan JF, Valentino RJ, Lucki I. (2005b). Assessing substrates underlying the behavioral effects of antidepressants using the modified rat forced swimming test. Neurosci Biobehav Rev 29:547–69.
- Dalla C, Antoniou K, Drossopoulou G, Xagoraris M, Kokras N, Sfikakis A, Papadopoulou-Daifoti Z. (2005). Chronic mild stress impact: are females more vulnerable? Neuroscience 135:703–14.
- de Weerth C, Buitelaar JK. (2005). Physiological stress reactivity in human pregnancy – a review. Neurosci Biobehav Rev 29:295–312.
- Gobinath AR, Mahmoud R, Galea LA. (2014). Influence of sex and stress exposure across the lifespan on endophenotypes of depression: focus on behavior, glucocorticoids, and hippocampus. Front Neurosci 8:420.
- Gobrogge K, Wang Z. (2015). Neuropeptidergic regulation of pair-bonding and stress buffering: lessons from voles. Horm Behav 76:91–105.
- Grippo AJ, Francis J, Beltz TG, Felder RB, Johnson AK. (2005a). Neuroendocrine and cytokine profile of chronic mild stress-induced anhedonia. Physiol Behav 84:697–706.
- Grippo AJ, Gerena D, Huang J, Kumar N, Shah M, Ughreja R, Carter CS. (2007a). Social isolation induces behavioral and neuroendocrine disturbances relevant to depression in female and male prairie voles. Psychoneuroendocrinology 32:966–80.
- Grippo AJ, Lamb DG, Carter CS, Porges SW. (2007b). Social isolation disrupts autonomic regulation of the heart and influences negative affective behaviors. Biol Psychiatry 62:1162–70.
- Grippo AJ, Moffitt JA, Henry MK, Firkins R, Senkler J, McNeal N, Wardwell J, et al. (2015). Altered Connexin 43 and Connexin 45 protein expression in the heart as a function of social and environmental stress in the prairie vole. Stress 18:107–14.
- Grippo AJ, Sullivan NR, Damjanoska KJ, Crane JW, Carrasco GA, Shi J, Chen Z, et al. (2005b). Chronic mild stress induces behavioral and physiological changes, and may alter serotonin 1A receptor function, in male and cycling female rats. Psychopharmacology (Berl) 179:769–80.
- Hammen C. (2005). Stress and depression. Annu Rev Clin Psychol 1:293–319.
- Heinrichs M, Baumgartner T, Kirschbaum C, Ehlert U. (2003). Social support and oxytocin interact to suppress cortisol and subjective responses to psychosocial stress. Biol Psychiatry 54:1389–98.
- Hurlemann R, Scheele D. (2016). Dissecting the role of oxytocin in the formation and loss of social relationships. Biol Psychiatry 79:185–93.
- Kiecolt-Glaser JK, Newton TL. (2001). Marriage and health: his and hers. Psychol Bull 127:472–503.
- Kim J, Richardson V, Park B, Park M. (2013). A multilevel perspective on gender differences in the relationship between poverty status and depression among older adults in the United States. J Women Aging 25:207–26.
- Kiyokawa Y, Kikusui T, Takeuchi Y, Mori Y. (2004). Partner’s stress status influences social buffering effects in rats. Behav Neurosci 118:798–804.
- Li S, Wang C, Wang W, Dong H, Hou P, Tang Y. (2008). Chronic mild stress impairs cognition in mice: from brain homeostasis to behavior. Life Sci 82:934–42.
- Mathers CD, Loncar D. (2005). Updated projections of global mortality and burden of disease, 2002–2030: data sources, methods and results. Geneva: World Health Organization.
- McNeal N, Scotti ML, Wardwell J, Chandler DL, Bates SL, LaRocca MA, Trahanas DM, Grippo AJ. (2014). Disruption of social bonds induces behavioral and physiological dysregulation in male and female prairie voles. Autonom Neurosci Basic Clin 180:9–16.
- Ramsay S, Ebrahim S, Whincup P, Papacosta O, Morris R, Lennon L, Wannamethee SG. (2008). Social engagement and the risk of cardiovascular disease mortality: results of a prospective population-based study of older men. Ann Epidemiol 18:476–83.
- Rivera-Torres P, Araque-Padilla RA, Montero-Simo MJ. (2013). Job stress across gender: the importance of emotional and intellectual demands and social support in women. Int J Environ Res Public Health 10:375–89.
- Sapolsky RM. (2015). Stress and the brain: individual variability and the inverted-U. Nat Neurosci 18:1344–6.
- Slattery DA, Neumann ID. (2008). No stress please! Mechanisms of stress hyporesponsiveness of the maternal brain. J Physiol 586:377–85.
- Smith AS, Wang Z. (2014). Hypothalamic oxytocin mediates social buffering of the stress response. Biol Psychiatry 15:281–8.
- Steptoe A, Shankar A, Demakakos P, Wardle J. (2013). Social isolation, loneliness, and all-cause mortality in older men and women. Proc Natl Acad Sci USA 110:5797–801.
- Steru L, Chermat R, Thierry B, Simon P. (1985). The tail suspension test: a new method for screening antidepressants in mice. Psychopharmacology (Berl) 85:367–70.
- Sun P, Smith AS, Lei K, Liu Y, Wang Z. (2014). Breaking bonds in male prairie vole: long-term effects on emotional and social behavior, physiology, and neurochemistry. Behav Brain Res 265:22–31.
- Tafet GE, Bernardini R. (2003). Psychoneuroendocrinological links between chronic stress and depression. Prog Neuropsychopharmacol Biol Psychiatry 27:893–903.
- Tannenbaum B, Tannenbaum GS, Sudom K, Anisman H. (2002). Neurochemical and behavioral alterations elicited by a chronic intermittent stressor regimen: implications for allostatic load. Brain Res 953:82–92.
- Thorsteinsson EB, James JE, Gregg ME. (1998). Effects of video-relayed social support on hemodynamic reactivity and salivary cortisol during laboratory-based behavioral challenge. Health Psychol 17:436–44.
- Willner P. (2005). Chronic mild stress (CMS) revisited: consistency and behavioural-neurobiological concordance in the effects of CMS. Neuropsychobiology 52:90–110.
- Young LJ, Lim MM, Gingrich B, Insel TR. (2001). Cellular mechanisms of social attachment. Horm Behav 40:133–8.
- Young LJ, Wang Z. (2004). The neurobiology of pair bonding. Nat Neurosci 7:1048–54.