Abstract
Medical education is a time of high stress and anxiety for many graduate students in medical professions. In this study, we sought to investigate the effect of academic stress on cortical excitability and plasticity by using transcranial magnetic stimulation (TMS). We tested two groups (n = 13 each) of healthy graduate medical students (mean age 33.7 ± 3.8 SE). One group was tested during a final exam week (High-stress group) while the other group was tested after a break, during a week without exams (Low-stress group). Students were required to fill the Perceived Stress Scale-10 (PSS) questionnaire. We investigated resting motor threshold (RMT), motor evoked potential (MEP) amplitude and cortical silent period (CSP). The paired-pulse stimulation paradigm was used to assess short interval intracortical inhibition (SICI) and intracortical facilitation (ICF). Long-term potentiation (LTP)-like plasticity was evaluated with paired associative stimulation (PAS-25). There was no between-group difference in cortical excitability. On the contrary, during examination period, levels of perceived stress were significantly higher (p= .036) and the amount of cortical plasticity (60 min after PAS) was significantly lower (p = .029). LTP-like plasticity (60 min after PAS) was inversely correlated with perceived stress in the High-stress group. The present study showed LTP-like plasticity was reduced by examining stress in graduate students. Our results provide a new opportunity to objectively quantify the negative effect of academic and examination stress on brain plasticity.
Introduction
Medical education (Allopathic and Osteopathic Medical Schools, Colleges of Podiatry and Schools of Health Sciences) is considered a time of high stress with remarkable challenges to personal well-being for students (Ludwig et al., Citation2015). Academic stress describes the prevalence and implications of study-related stress among students and the negative impact that it has on their performance (Struthers et al., Citation2000). A number of factors including academic pressure, large course workload, financial concerns, sleep deprivation, exposure to patients’ suffering and deaths have been identified as stressor factors that negatively influence students (Dyrbye et al., Citation2006). Several studies have shown increased levels of stress during periods of academic examinations that may lead to anxiety, emotional distress and impairment of recalling information skills, attention, working memory and executive functions (Mogg et al., Citation1994).
At the cellular level, long-term potentiation (LTP) is one of the main mechanism underlying learning, memory formation and neuroplasticity (Rioult-Pedotti et al., Citation2000). Transcranial magnetic stimulation (TMS) provides a noninvasive method to study cortical circuitries in the human brain. Previous work has identified LTP-like plastic changes induced using paired associative stimulation (PAS) in humans (Stefan et al., Citation2000). This neurophysiological paradigm comprises of electrical stimulation of a peripheral nerve followed by TMS of the contralateral motor cortex (M1) (Stefan et al., Citation2000). Repeated pairing of the stimuli over an extended period tends to increase or decrease the excitability of corticospinal projections from M1 in a manner that depends on the interstimulus interval (ISI). With a 25 ms interstimulus interval between paired stimuli, PAS induces an increase in corticomotor excitability in the target muscle through an LTP-like process (Stefan et al., Citation2000). LTP-like plasticity has characteristics similar to LTP in vitro and can be used to test human’s ability to adapt to its surroundings on basis of experience (Ziemann et al., Citation2004).
A number of studies in animal models have demonstrated that stress disrupts LTP, learning and memory (McEwen et al., Citation2015). Furthermore, it was reported that LTP-like plasticity in human M1 is regulated by endogenous cortisol levels (Sale et al., Citation2008). Nonetheless, to date no studies have been performed assessing the effect of stress on LTP-like plasticity in a naturalistic environment.
The aim of the present study is to investigate the effect of academic examination stress on cortical excitability and plasticity in the attempt to improve the field’s understanding of the mechanisms involved in the effect of real-life stressors in college students.
Methods
Subjects and ethical approval
We studied two groups (n = 13 each) of right-handed healthy students (first year, podiatry students mean age 33.7 ± 3.8 SE). One group was tested during a final exam week (High stress group) while the other group was tested after a break, during a week without exams (Low stress group). As PAS is influenced by the time of day (Sale et al., Citation2008), all subjects were tested in the afternoon (4 PM) in order to minimize the effects of differences in circadian endogenous cortisol secretion. None of the participants had a history of neurological or psychiatric disorders. The study was conducted in accordance with the Declaration of Helsinki and was approved by the Institutional Review Board of the New York College of Podiatric Medicine. Subjects were comfortably seated on a chair.
Electromyographic recording
Electromyography (EMG) activity was recorded from the relaxed right abductor pollicis brevis (APB) muscle using surface electrodes (belly-tendon configuration). Data were band-pass filtered (20–2000 Hz), amplified and digitized at 5 kHz using a MICRO1401 data acquisition unit (Cambridge Electronic Design Ltd., Cambridge, UK). Data were analyzed using Signal Software version 3 (Cambridge Electronic Design Ltd., Cambridge, UK).
Transcranial magnetic stimulation
A Magstim-200 stimulator generated single-pulse stimuli, delivered through a figure-of-eight coil (9 cm) placed tangentially to the scalp with the handle pointing backward, 45° away from the midline. We then mapped the motor strip and detected the most excitable site in the motor cortex that elicited the maximum MEP response (highest MEP amplitude) in the right APB after single TMS pulse (hotspot). We tested resting motor threshold (RMT), defined as the minimum stimulation intensity necessary to evoke MEPs of 50 mV in 50% of 10 consecutive trials while the targeted hand was relaxed (Rossini et al., Citation1994). We then recorded MEP amplitude using a stimulation intensity of 130% RMT. Cortical Silent Period (CSP) was tested at a stimulus intensity of 130% RMT during APB contraction (50% of maximum voluntary force). Ten repeats were recorded (Rossini et al., Citation2015). Short interval intracortical inhibition (SICI) and intracortical-facilitation (ICF) were tested by paired-TMS (Kujirai et al., Citation1993). A conditional stimulus (CS) was set at 80% RMT delivered 2 ms before a test stimulus (SICI), while we used an ISI of 10 ms for the induction of ICF. The test stimulus was adjusted to induce consistent MEP amplitude of ∼1 mV. Twenty MEPs were recorded for each condition and SICI and ICF were expressed as percentage of conditioned/unconditioned MEP. Thus, values below 100% correspond to inhibition and values above 100% correspond to facilitation. To clarify a possible spinal or peripheral contribution on motor cortical excitability, spinal motorneuron and neuromuscular excitability were tested by using F-wave amplitude and M-max, using supra-maximal electrical stimulation of the median nerve at the wrist (Kujirai et al., Citation1993).
Paired associative stimulation
Cortical plasticity was evaluated by using a well-characterized protocol (PAS-25, 200 paired stimuli at 0.25 Hz) that induced long-lasting MEPs potentiation (Ziemann et al., Citation2004). Briefly, peripheral electric stimulation of the median nerve at the wrist of the right hand was applied through a Digitimer DS7 electrical stimulator (Digitimer Ltd., Welwyn Garden City, Hertfortshire, UK) using a bipolar electrode with the cathode proximal. The intensity of the peripheral stimulation was set at three times the perception threshold (constant current square wave pulses with duration of 1000 μs). TMS intensity was set to obtain a MEP amplitude of about 1 mV. During PAS, the subjects were asked to look at the stimulated hand and to count the number of stimuli.
Perceived stress scale (PSS-10)
Prior to the TMS experiments, students were required to fill the Perceived Stress Scale-10 (PSS) questionnaire. PSS-10 is widely used to measure the perception of stress. It consists of 10 items designed to assess the degree to which common life situations are deemed stressful, referencing to the past month. Responses are made on a 5-point Likert scale ranging from 1 (never) to 5 (very often), with higher scores reflecting greater perceived stress (Cohen et al., Citation1983).
Statistical analysis
Between-group differences in cortical, spinal excitability parameters and PSS-10 score were analyzed with ANOVA. The effects of PAS-25 on MEP amplitude was assessed using a mixed model repeated-measure design with main effect “time” as within subject (two levels T0, T60); main effect “group” (two levels, Low and High stress) as between subject factors. The Greenhouse–Geisser correction and a pairwise comparison with the Bonferroni correction were performed when appropriate. The relationship between the MEP amplitude at T60 after PAS and the PSS-10 score was tested using the Sperman’s Rho correlation coefficient. Data were analyzed using SPSS Version 20 (IBM Corporation, Chicago, IL). Statistical significance was set at 0.05. Mean are ± standard error (SE).
Results
None of the participants had any adverse event during the study and there was no difference between the groups in the number of magnetic stimuli counted during the induction of the LTP-like cortical plasticity (p > .05). Levels of perceived stress were significantly higher during the final examination period [(F(1, 24) = 17.3, p = .0003)] (). There were no differences in RMT between the two groups [Low stress: 42 ± 2%, High stress: 44.8 ± 2.4%; F(1, 24) = 0.77, p = .39]. Stress did not have a significant effect on MEP amplitude [Low stress: 2.2 ± 0.13 mV, High stress: 2.1 ± 0.12 mV; F(1, 24) = 0.2, p =.66] and CSP duration [Low stress: 157.4 ± 16.9 ms, High stress: 146.3 ± 15.2 ms; F(1, 24) = 0.56, p = .45]. Similar results were obtained during the paired-pulse paradigm for SICI [Low stress: 40 ± 4.22%, High stress: 37.7 ± 5.1%; F(1, 24) = 0.19, p = .66] and for ICF [Low stress: 140 ± 6.2%, High stress: 148 ± 5.4%: F(1, 24) = 0.96, p = .34). Furthermore, no significant differences were found in spinal cord excitability [Mmax, Low stress: 14.6 ± 1.9%, High stress: 15.1 ± 1.2%, F(1, 24) = 0.6, p = .71; F-wave, Low stress: 223.4 ± 28.4%, High stress: 211.6 ± 25.1%, F(1, 24) = 0.8, p = .87].
Figure 1. Perceived stress scale (PSS). Perceived stress levels were significantly higher in the High stress group compared with the Low stress group. Error bars represent standard error of the mean. **p<.01.
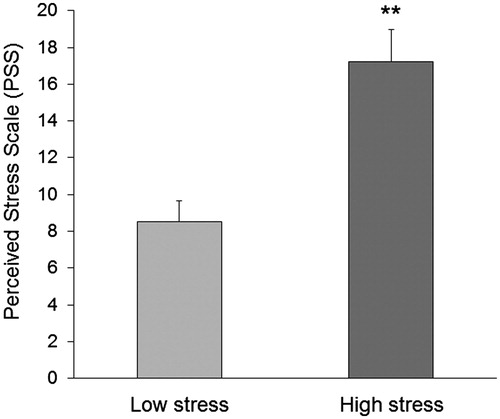
In changes in the MEP amplitudes before and after PAS are plotted. A repeated measures ANOVA with main factors “Time” (baseline, 0 and 60 minutes after PAS) and “Group” (Low stress and High stress) showed a significant “Time”×”Group” interaction [F(2,48) = 7.52, p = .029] indicating that the Low-stress group showed a larger increase in MEP size after PAS. Post-hoc analysis indicated that after PAS MEP increased in both groups (Low stress: compared to baseline, T0: p < .0001, T60: p < .0001; High stress: compared to baseline, T0: p = .0071, T60: p = .0001). Furthermore, pairwise comparison showed that there was no difference between groups in baseline MEP size (p > .05) whereas the Low-stress group showed higher increased MEP size after PAS both at T0 (p = .02) and T60 (p = .0005). Furthermore, the High-stress group demonstrated a weak inverse correlation between the MEP size at T60 and PSS-10 mean score, indicating a relationship between stress perception and long-term cortical plasticity (Spearman’s rank correlation: High stress p = .04) (); on the contrary, no correlation was found in the Low stress group (p = .6).
Figure 2. Paired associative stimulation (PAS). MEP amplitudes were measured in both groups at baseline, immediately (T0) and 60 minutes after PAS (T 60). There was no significant difference between groups at baseline. At T0 and T60 the Low stress group showed significant higher increased MEP size. Error bars represent standard error of the mean. *p<.05; **p<.01.
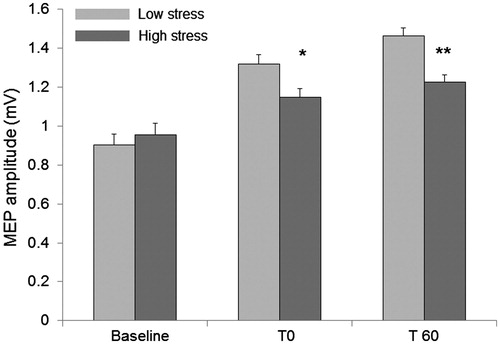
Discussion
In this study we found that students undergoing final examinations exhibited decreased cortical LTP-like plasticity compared to students tested in a less stressful time during the semester. In addition, perceived stress levels were significantly higher in the High-stress group and inversely correlated with the amount of cortical plasticity.
A number of studies have documented the effects of work demands on psychological and physiological processes. Our results are consistent with previous reports indicating that examinations are the most important stressors for medical students (Crego et al., Citation2016). As previously suggested by Ali et al. (Citation2015), the higher levels of perceived stress reported by us might be related to apprehension and frustration associated with possible academic failure (Ali et al., Citation2015). Our results are different from a previous report that showed no increase in PSS-10 score during examination week (Murphy et al., Citation2010). In our study, students were tested before a gross anatomy final exam that is typically perceived as one of the most demanding and stressful exam during the entire medical curriculum across medical professions. Thus, the different study population and curriculum demand (undergraduate vs graduate medical college) could account for these discordant results. Future work should aim to continue to untangle the interaction between academic demand and perceived stress.
In this study we used several TMS parameters to assess both cortical excitability and LTP-like plasticity. Previous studies demonstrated that cortical excitability and LTP-like plasticity can be modulated differently by neurodegenerative processes and experimental manipulations (drugs, motor tasks, behaviors) (Eldaief et al., Citation2013). Our results indicate that there were no between-group differences in cortical excitability whereas the use of PAS to investigate cortical LTP-like processes (Stefan et al., Citation2000) demonstrated a significant reduction in the amount of MEP potentiation in the High-stress group. As LTP-like plasticity is quantifiable and it is less subjective than self-report, it might represent a new biomarker. We can only speculate about the possible mechanisms underlying these results. Previous studies demonstrated that academic and examination stress significantly increase cortisol serum levels (Murphy et al., Citation2010). As MEP facilitation after PAS shows circadian fluctuation and is influenced by oral hydrocortisone administration (Sale et al., Citation2008), it is conceivable that the reduction of LTP-like plasticity observed in the High-stress group might be linked to the increase of circulating cortisol levels. Furthermore, sustained stress alters the physiologic noradrenergic activity and exerts negative effects on attention (Robbins & Arnsten, Citation2009) and cognitive processes (Roozendaal et al., Citation2006). The pharmacological modulation of LTP-like plasticity with noradrenergic drugs still need to be further explored (Heidegger et al., Citation2010). Nonetheless, previous studies demonstrated a link between LTP-like plasticity, attention and alertness (Stefan et al., Citation2004). Thus, we cannot rule out that attention and arousal might have played a role in the impairment of LTP-like cortical plasticity.
A complex relationship exists between excitatory neurotransmitters, adaptive and deleterious effects of stressors in the brain (Sapolsky, Citation2003). For instance, it is well known that stress inhibits LTP induction and facilitates LTD generation in animal models (Foy et al., Citation1987). LTP-like plasticity in humans follows Hebbian rules and is modulated by glutamate signaling and postsynaptic cell depolarization through N-methyl-d-aspartate (NMDA) receptor (Stefan et al., Citation2002) and we can assume that abnormal glutamatergic neurotransmission might have had a role in reducing LTP-like neuroplasticity in highly stressed students. In addition, stress-induced behaviors such as sleep deprivation known to influence immune functioning might have had a negative indirect influence on cortical physiology (Crupi et al., Citation2010). Thus, poor sleep behavior and daytime sleepiness occurring in highly stressed students might have had a detrimental effect on mood, cognitive performance and overall ability to respond to challenges. All these factors should be taken into account in the interpretation of our findings.
Our work has some limitations that should be addressed in future studies. The mechanistic explanations of the results are mostly speculative at present. It would be advisable to confirm our results with a larger sample, to test additional biological biomarkers (i.e. salivary cortisol, heart rate variability, immune system activation) and to evaluate the relationship between academic assessment, psychological distress and LTP-like cortical plasticity. In addition, the contribution of sleep deprivation to LTP-like plasticity impairment in graduate students should be addressed in future studies.
Conclusions
In conclusion, the present study showed for the first time that examination stress reduces LTP-like cortical plasticity. Our results indicated that LTP-like plasticity might be a potential safe and inexpensive biomarker to objectively quantify the effects of behavioral, stress-coping interventions aimed to prevent the negative effect of high stress period during the semester in students.
Acknowledgments
The authors thank the students who participated in the study
Disclosure statement
The authors report no conflicts of interest. The authors alone are responsible for the content and writing of the paper
References
- Ali M, Asim H, Edhi AI, Hashmi MD, Khan MS, Naz F, Qaiser KN, et al. (2015). Does academic assessment system type affect levels of academic stress in medical students? A cross-sectional study from Pakistan. Med Educ Online 20:27706.
- Cohen S, Kamarck T, Mermelstein R. (1983). A global measure of perceived stress. J Health Soc Behav 24:385–96.
- Crego A, Carrillo-Diaz M, Armfield JM, Romero M. (2016). Stress and academic performance in dental students: the role of coping strategies and examination-related self-efficacy. J Dent Educ 80:165–72.
- Crupi R, Cambiaghi M, Spatz L, Hen R, Thorn M, Friedman E, Vita G, Battaglia F. (2010). Reduced adult neurogenesis and altered emotional behaviors in autoimmune-prone B-cell activating factor transgenic mice. Biol Psychiatry 67:558–66.
- Dyrbye LN, Thomas MR, Shanafelt TD. (2006). Systematic review of depression, anxiety, and other indicators of psychological distress among U.S. and Canadian medical students. Acad Med 81:354–73.
- Eldaief MC, Press DZ, Pascual-Leone A. (2013). Transcranial magnetic stimulation in neurology: a review of established and prospective applications. Neurol Clin Pract 3:519–26.
- Foy MR, Stanton ME, Levine S, Thompson RF. (1987). Behavioral stress impairs long-term potentiation in rodent hippocampus. Behav Neural Biol 48:138–49.
- Heidegger T, Krakow K, Ziemann U. (2010). Effects of antiepileptic drugs on associative LTP-like plasticity in human motor cortex. Eur J Neurosci 32:1215–22.
- Kujirai T, Caramia MD, Rothwell JC, Day BL, Thompson PD, Ferbert A, Wroe S, et al. (1993). Corticocortical inhibition in human motor cortex. J Physiol (Lond) 471:501–19.
- Ludwig AB, Burton W, Weingarten J, Milan F, Myers DC, Kligler B. (2015). Depression and stress amongst undergraduate medical students. BMC Med Educ 15:141.
- McEwen BS, Bowles NP, Gray JD, Hill MN, Hunter RG, Karatsoreos IN, Nasca C. (2015). Mechanisms of stress in the brain. Nat Neurosci 18:1353–63.
- Mogg K, Bradley BP, Hallowell N. (1994). Attentional bias to threat: roles of trait anxiety, stressful events, and awareness. Q J Exp Psychol A 47:841–64.
- Murphy L, Denis R, Ward CP, Tartar JL. (2010). Academic stress differentially influences perceived stress, salivary cortisol, and immunoglobulin-A in undergraduate students. Stress 13:365–70.
- Rioult-Pedotti MS, Friedman D, Donoghue JP. (2000). Learning-induced LTP in neocortex. Science 290:533–6.
- Robbins TW, Arnsten AF. (2009). The neuropsychopharmacology of fronto-executive function: monoaminergic modulation. Annu Rev Neurosci 32:267–87.
- Roozendaal B, Okuda S, de Quervain DJ, McGaugh JL. (2006). Glucocorticoids interact with emotion-induced noradrenergic activation in influencing different memory functions. Neuroscience 138:901–10.
- Rossini PM, Barker AT, Berardelli A, Caramia MD, Caruso G, Cracco RQ, et al. (1994). Non-invasive electrical and magnetic stimulation of the brain, spinal cord and roots: basic principles and procedures for routine clinical application. Report of an IFCN committee. Electroencephalogr Clin Neurophysiol 91:79–92.
- Rossini PM, Burke D, Chen R, Cohen LG, Daskalakis Z, Di Iorio R, Di Lazzaro V, et al. (2015). Non-invasive electrical and magnetic stimulation of the brain, spinal cord, roots and peripheral nerves: Basic principles and procedures for routine clinical and research application. An updated report from an I.F.C.N. Committee. Clin Neurophysiol 126:1071–107.
- Sale MV, Ridding MC, Nordstrom MA. (2008). Cortisol inhibits neuroplasticity induction in human motor cortex. J Neurosci 28:8285–93.
- Sapolsky RM. (2003). Stress and plasticity in the limbic system. Neurochem Res 28:1735–42.
- Stefan K, Kunesch E, Benecke R, Cohen LG, Classen J. (2002). Mechanisms of enhancement of human motor cortex excitability induced by interventional paired associative stimulation. J Physiol 543(Pt2):699–708.
- Stefan K, Kunesch E, Cohen LG, Benecke R, Classen J. (2000). Induction of plasticity in the human motor cortex by paired associative stimulation. Brain 123(Pt 3):572–84.
- Stefan K, Wycislo M, Classen J. (2004). Modulation of associative human motor cortical plasticity by attention. J Neurophysiol 92:66–72.
- Struthers CV, Perry RP, Menec VH. (2000). An examination of the relationship among academic stress, coping, motivation, and performance in college. Res High Educ 41:581–92.
- Ziemann U, Ilic TV, Pauli C, Meintzschel F, Ruge D. (2004). Learning modifies subsequent induction of long-term potentiation-like and long-term depression-like plasticity in human motor cortex. J Neurosci 24:1666–72.