Abstract
Heat stress is a problem in laying hens as it decreases egg quality by decreasing eggshell mineralization. Heat stress alters gene expression, hence our aim was to investigate effects of heat stress on gene expression of ion transport elements involving in uterine mineralization (TRPV6, CALB1, ITPR3, SCNN1G, SLC4A4, KCNJ15, SLC4A9, and CLCN2) by real time quantitative PCR. Forty 23-week-old White Leghorn laying hens were housed in two rooms. The control group (n = 20) was maintained at 21–23 °C, and the heat stress group (n = 20) was exposed to 36–38 °C for 8 weeks. All parameters of egg quality including egg weight, surface area, volume, and eggshell weight, thickness, ash weight, and calcium content were decreased in the heat stress group compared to the control group (by 26.9%, 32.7%, 44.1%, 38.4%, 31.7%, 39.4%, and 11.1%, respectively). Total plasma calcium was decreased by 13.4%. Levels of ITPR3, SLC4A4, and SLC4A9 transcripts in the uterine lining were decreased in the heat stress group compared to the control group (by 61.4%, 66.1%, and 66.1%, respectively). CALB1 transcript level was increased (by 34.2 fold) in the heat stress group of hens compared to controls. TRPV6, SCNN1G, KCNJ15, and CLCN2 transcript levels did not significantly differ between control and heat stress groups of laying hens. It is concluded that the down-expression of ITPR3, SLC4A4, and SLC4A9 genes may impair transportation of Cl−, HCO3−, and Na+ in eggshell mineralization during heat stress. Increased CALB1 gene expression may increase resistance of uterine cells to detrimental effects of heat stress.
Introduction
Heat stress is a major concern in the modern poultry industry. Its adverse effect on egg production is well recognized, but the mechanism involved is not clearly understood. The optimal temperature range for birds is between 16 and 25 °C (Song, Liu, Sheikhahmadi, Jiao, & Lin, Citation2012). It has been determined that for every 1 °C increase in temperature between 21 °C and 30 °C, appetite decreases by 1.5%, and for every 1 °C increase in temperature between 32 °C and 38 °C, the reduction is about 4.6% (Song et al. Citation2012). In addition to reduced feed intake, heat stress can generally suppress feed digestibility (Allahverdi, Feizi, Takhtfooladi, & Nikpiran, Citation2013; Mashaly et al., Citation2004; Puthpongsiriporn, Scheideler, Sell, & Beck, Citation2001; Sahin and Kucuk, Citation2001), decrease eggshell quality (Lara and Rostagno, Citation2013; St-Pierre, Cobanov, & Schnitkey, Citation2003), and diminish the ability of enterocytes to absorb calcium in laying hens, which is a critical factor in declining eggshell characteristics (Allahverdi et al., Citation2013; Mahmoud et al., Citation1996; Quinteiro-Filho et al., Citation2010).
Changes in the expression of genes could partially be a reason for the adverse effects of heat stress (Song et al., Citation2012). Many studies have been conducted to evaluate the effect of heat stress on the expression of genes (Sonna, Fujita, Gaffin, & Lilly, Citation2002). Most of these genes play crucial roles in cell functions such as apoptosis, cell adhesion, cell cycle, and cell differentiation (Altan, Pabuçcuoğlu, Altan, Konyalioğlu, & Bayraktar, Citation2003; Mujahid, Yoshiki, Akiba, & Toyomizu, Citation2005; Starkie, Hargreaves, Rolland, & Febbraio, Citation2005).
Biomineralization is a process by which birds form the eggshell through mineralization, via co-precipitation of calcium and carbonate ions. The prerequisite for shell mineralization is the supply of large amounts of these ions in a limited extracellular environment by trans-cellular transport, requiring the presence and activation of ion channels, ion pumps and ion exchangers (Brionne, Nys, Hennequet-Antier, & Gautron, Citation2014; Jonchère, Brionne, Gautron, & Nys, Citation2012). In hens, the uterus is the principle site of eggshell formation, providing the most rapid mineralization processes (Nys, Gautron, Garcia-Ruiz, & Hincke, Citation2004). It has been determined that several proteins contribute in mineralization by transporting the ions (Brionne et al., Citation2014). Calcium is an essential element in the process of calcification of the eggshell, and is continuously supplied from the blood into the uterus against the concentration gradient. Calcium transfer into the uterine fluid involves TRPV6 or other calcium channels for entry into uterine glandular cells, calbindin D28k (CALB1) for intracellular transport, and the calcium output is ensured by Na+/Ca2+ or Ca2+/H+ calcium exchangers. Calcium levels in the cell can be regulated by calcium uptake and release in the endoplasmic reticulum via ATP-dependent calcium pumps (e.g. ATP2A) and Ca2+ channels (e.g. ITPRs) () (Brionne et al., Citation2014; Jonchère et al., Citation2012).
Table 1. Functions of uterine genes potentially involved in eggshell formation.
Carbonate is another major component of the shell. In the uterine glandular cells, CO2 is hydrated by carbonic anhydrases to produce bicarbonate. Low levels of bicarbonate are transferred into the cell by transporters such as a Na+/HCO3− co-transporter (e.g. coded by SLC4A4). Bicarbonate is transferred from uterine cells into the uterine fluid via a Cl−/HCO3− exchanger, such as that encoded by SLC4A9. The transfer of calcium and bicarbonate from the blood to the uterine fluid involves additional ion transfer to maintain cell homeostasis. There are exchanges of Na+, K+, and Cl− ions to maintain physiological ionic concentrations in the cell. In addition, there are transporters for K+ (e.g. KCNJ15), Cl− (e.g. CLCN2), and Na+ (e.g. subunit coded by SCNN1G) in the plasma membranes of uterine glandular cells (; ) (Brionne et al., Citation2014; Jonchère et al., Citation2012). The present study was designed to investigate the effects of heat stress on the gene expression of many ion transport elements (TRPV6, CALB1, ITPR3, SCNN1G, SLC4A4, KCNJ15, SLC4A9, and CLCN2) involving in eggshell formation. We hypothesized that impairment of eggshell formation in hens during heat stress would partially be due to defect in the gene expression of uterine ion transporters/channels.
Methods
Birds and treatments
The Institutional Animal Care and Use Committee of the University of Shahrekord approved all procedures throughout this experiment. Forty 23-week-old White Leghorn laying hens were housed in individual cages (40 × 40 × 40 cm) under ambient temperatures and were provided 16 h of artificial light/day (06:00 h–22:00 h). Hens were fed ad libitum with commercial layer feed, formulated according to NRC (Citation1994). Birds were divided into two groups (n = 20 each) and transferred to one of two rooms. Both rooms provided similar environmental conditions (21–23 °C, 35% humidity). The laying hens in the control group were maintained under these conditions during the period of the experiment (9 weeks) while the laying hens of the treated group (heat stress group) were exposed to high temperature after one week. The room temperature in this group was daily increased by 3 °C to 36–38 °C, after Mashaly et al. (Citation2004) with slight modification. This high temperature was maintained during the rest of the experimental period. Any mortality of hens was recorded in the two groups during the experiment.
Measurement of egg quality parameters
Egg-laying times of hens in both experimental groups were checked daily and recorded. The time of laying in the two groups was around 07:00 h–10:00 h. The eggs of laying hens were collected in the last week of the experiment (at the peak of production; egg production by ≥95% of the flock). Parameters of egg quality i.e. egg weight, egg volume, egg surface area, eggshell weight, eggshell thickness, eggshell ash weight, and percent of eggshell calcium were estimated. All dimensions of egg or eggshell were measured with a digital caliper. To obtain eggshell ash, egg shells were heated at 550 °C for 6 hours. Eggshell calcium was measured via the method of calcium titration using potassium permanganate (Berger, Citation1955). To estimate egg volume (V), the following formula was used: V = (0.6057 − 0.0018B) LB2, in which L is the egg length in millimeters, and B is the maximum egg breadth in millimeters. Egg surface area (S) was calculated as S = (3.155 − 0.0136L + 0.0115B) LB (De Witt, Kuleile, Van Der Merwe, & Fair, Citation2009; Narushin, Citation2005).
Measurement of plasma calcium
Laying hens (10 birds per group) were randomly selected for blood collection at the end of the experiment. Blood samples, 3 ml per bird, were collected from the brachial vein of conscious birds in heparinized syringes and centrifuged at 2500 g for 10 min to obtain plasma. The total amount of calcium in plasma was quantified by using a Calcium (Arsenazo) kit (Pars Azmoon co., Tehran, Iran) following the manufacturer’s instructions. The Calcium (Arsenazo) kit is a quantitative test, determining total calcium via spectrophotometry. This kit has an intra-assay variation <14% and inter-assay variation <13.8%. The assay limit of this kit is 0.4 mg/dl.
Total RNA extraction and cDNA preparation
At the end of the experiment, 10 hens per group were decapitated under barbiturate anesthesia (Pentobarbital sodium, 50 mg/mL; dose of 30 mg/kg, IV), then samples of uterine tissue were obtained. Time of sampling was 10 hours before the recorded time of laying, when the eggshell was being formed in the uterus. The lining surface (glands + epithelium) of the uterine samples was scraped and then was frozen in liquid nitrogen and stored at −70 °C. Frozen samples were homogenized in a liquid nitrogen bath. Total RNA was isolated from homogenized samples (100 mg) using RNX-Plus (Sinaclon Bioscience, Karaj, Iran) as per the manufacturer’s recommendations and according to Ahmadipour et al. (Citation2015). The extracted RNA was treated with DNase (Sinaclon Bioscience, Karaj, Iran) to remove contaminating DNA. Agarose gel electrophoresis was performed for extracted RNA, and its quality was verified by demonstration of intact 28 S and 18 S ribosomal bands in addition to absorbance ratios (A260/280) of 1.8 to 2.0.
cDNA was prepared from total RNA using a PrimeScript™ RT Reagent Kit (Takara Bio Inc., Japan). According to the manufacturer of the kit, following reverse transcription, the mix was heated to 85 °C for 5 s to denature the residual RNA and inactivate reverse transcriptase. The cDNA was stored at −20 °C until real time PCR was performed (Ahmadipour et al., Citation2015).
Real-time quantitative PCR
PCR reactions were performed in a real-time PCR cycler (Rotor Gene Q 6000, Qiagen, USA) to analyze mRNA expression of the TRPV6, CALB1, ITPR3, SCNN1G, SLC4A4, KCNJ15, SLC4A9, and CLCN2 genes in the control group and the group exposed to heat stress. To normalize the input load of cDNA between samples, the expression of β-actin was used as an endogenous stable reference. The gene for β-actin was selected as the most stable housekeeping gene by using the BestKeeper program between four candidate reference genes for β-actin, TBP, 18SrRNA, and RPL32. These genes had been already used in laying hens or chickens as stable reference genes (Bagés, Estany, Tor, & Pena, Citation2015; Brionne et al., Citation2014; Jonchère et al., Citation2012; Hassanpour Afzali, Fatemi Tabatabaie, Torabi, & Alavi, Citation2016). According to this program, an index is estimated by the geometric mean of the cycle threshold values for all candidate genes. Then, they are compared by Pearson correlation coefficients in a pair-wise fashion to that index. The ranking based on the correlation coefficient in this program was β-actin 0.83, 18SrRNA 0.73, RPL32 0.15, and TBP 0.14.
Real-time PCR details have been published previously (Ahmadipour et al., Citation2015; Hassanpour, Khosravi Alekoohi, Madreseh, Bahadoran, & Nasiri, Citation2016). In brief, Real-time PCR was performed using SYBR green Premix Ex Taq II (Takara Bio Inc, Shiga, Japan). The full name and function of target genes are dedicated in . The sequence of specific primers of the mentioned genes was determined with Primer-BLAST (https://www.ncbi.nlm.nih.gov/tools/primer-blast/). Details of the primers are presented in . The expected PCR products of primers were examined in Nucleotide BLAST (https://blast.ncbi.nlm.nih.gov/Blast.cgi?PROGRAM=blastn&PAGE_TYPE=BlastSearch&LINK_LOC=blasthome), which found no similarity with other genes of Gallus gallus species. The PCR products were attained by a program consisting of 40 cycles 94 °C for 30 seconds, 61 °C to 63 °C for 31 seconds, and 72 °C for 25 seconds. To assess possible contamination of the PCR mix, no-template control and no-reverse-transcriptase control were used. The melt curve analysis of PCR products was considered to assess whether single and specific product of genes had been produced (). LinRegPCR software version 2012.0 (Amsterdam, Netherlands) was used to analyze threshold cycle number (Ct) and to give mean efficiency values (E) for each gene. The quantitative assessment of genes per β-actin values was made by Ct and E according to Hassanpour, Afzali, et al. (Citation2016). The mRNA level of each target gene relative to β-actin was estimated for each sample in two experimental groups using the following formula:
Figure 2. Specificity of real time PCR amplification. Melting curves (dissociation curves) of the 8 target genes and 1 reference gene (β-actin) amplicons after the real time PCR reactions, all showing one peak. X-axis (horizontal): temperature (°C); Y-axis (vertical): negative derivative of fluorescence over temperature (−dF/dT).
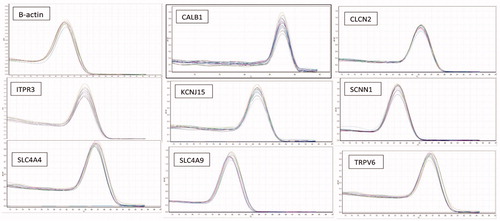
Table 2. Primers used for quantitative real time PCR analysis of mRNAs in laying hen uterus.
The fold change of genes was calculated by dividing the relative gene expression of the heat stress group to the control group.
Statistical analysis
Data are presented as mean ± SE. Comparisons were made for the relative expression of each gene in the uterus between the control and heat stress groups using t-tests. Statistical analysis was done using Prism version 6.07 software (GraphPad Software, Inc., CA). p Values less than .05 were considered significant.
Results
Assessment of egg quality
The egg quality parameters were evaluated in laying hens of control (n = 20) and heat stress groups (n = 17) in the last week of the experiment. All parameters of egg quality including egg weight, surface area, volume, and eggshell weight, thickness, ash weight, and calcium content were significantly decreased in the heat stress group compared to the control group The percentage decrease was 26.9% for egg weight (p = .031, t-test), 32.7% for surface area (p = .029, t-test), 44.1% for volume (p = .025, t-test), 38.4% for eggshell weight (p = .013, t-test), 31.7% for thickness (p = .018, t-test), 39.4% for ash weight (p = .009, t-test), and 11.1% (p = .010, t-test) for calcium content ().
Table 3. Parameters of egg quality in last week of experiment.
Assessment of plasma calcium
Total calcium concentration was measured in plasma samples from laying hens in the last week of the experiment. Plasma calcium concentration was significantly decreased (by 13.4%) in the heat stress group of hens (n = 10, 15.37 ± 0.07 mg/dl) compared to the control group (n = 10, 17.43 ± 0.11 mg/dl) (p = .011, t-test).
Expression of ion transporter/channel genes in the uterus
Expression of TRPV6, CALB1, ITPR3, SCNN1G, SLC4A4, KCNJ15, SLC4A9, and CLCN2 genes was studied using real time quantitative PCR (RT-qPCR) in the uterus of laying hens after being exposed to heat stress, or normal temperature for 8 weeks. RT-qPCR results are shown in . The expression of the β-actin gene was detected in all samples. The TRPV6, CALB1, ITPR3, SCNN1G, SLC4A4, KCNJ15, SLC4A9, and CLCN2 genes were expressed in the uterus of control and heat stressed laying hens. The relative amounts of ITPR3, SLC4A4 and SLC4A9 mRNAs expressed in the uterus were significantly lower in the heat stress group (n = 10) of hens than in control hens (n = 10) (p = .044, .038, and .032, respectively; t-test). The percentage decrease was 61.4% for ITPR3, 66.1% for SLC4A4, and 66.1% for SLC4A9. The relative amount of CALB1 mRNA expression in the uterus was significantly (p = .044, t-test) increased (34.2 fold) in the heat stress group (n = 10) compared to control hens (n = 10). The relative amounts of TRPV6, SCNN1G, KCNJ15, and CLCN2 in the uterus did not significantly differ between the control and heat stress groups of laying hens ().
Table 4. Relative gene expression of ion transporters in the uterine lining (glands + epithelium) of laying hens.
Mortality rate
The mortality rate in heat stress group of hens was 15% while there was no mortality in the control group.
Discussion
This study examined the effect of heat stress on gene expression of ion transport elements involved in uterine mineralization in laying hens. We have shown that defects of eggshell formation in the hens during heat stress is associated with impairment in the gene expression of uterine ion transporters/channels such as ITPR3, SLC4A4 and SLC4A9 and CALB1 which have critical roles in the transportation of Cl−, HCO3−, and Na+ for eggshell mineralization in the hen uterus during the peak of egg production.
Impairment of poultry reproduction during heat stress, is due to its effect on the hypothalamic–pituitary–gonad axis (Rozenboim, Tako, Gal-Garber, Proudman, & Uni, Citation2007; Sun et al., Citation2015). In chickens, many gene transcripts are up- or down-regulated by heat stress. These genes are related to the heat shock protein family, cell adhesion, transcription, development, and apoptosis genes (Wang et al., Citation2013). In the laying hen ovary, heat stress also causes considerable reduction in the transcripts for cytochrome P450 and 17-α hydroxylase, leading to ovarian failure (Rozenboim et al., Citation2007).
The present research investigated the plasma calcium, egg quality and mRNA levels of many ion transporters/channels (TRPV6, CALB1, ITPR3, SCNN1G, SLC4A4, KCNJ15, SLC4A9, and CLCN2) in the uterine tissue of laying hens exposing to heat stress for 8 weeks. This study was performed while the hens were at the maximum level of egg production (egg production by ≥95% of the flock) and the egg was present in the uterus at the stage of the beginning of active calcification. As documented in laying hens, heat stress causes a considerable defect of calcium absorption in the intestinal cells, leading to hypocalcemia and defects in egg production and eggshell characteristics (Allahverdi et al., Citation2013; Mahmoud et al., Citation1996; Mashaly et al., Citation2004). In the present study, hypocalcemia was also found in the heat stress group of hens which was probably due to reduction of Ca2+ absorption in the intestinal enterocytes. Calcium metabolism is a critical process in laying hens. During eggshell formation, the uterine cells must secrete about 2.3 g Ca2+ for each egg. This amount of calcium is equivalent to 10% of the total body calcium. The failure to provide this amount of calcium could negatively influence eggshell mineralization (Ebeid, Suzuki, & Sugiyama, Citation2012).
In our experiment, we also evaluated parameters of egg quality to confirm the harmful effects of induced heat stress in the reproductive system of hens. Hence the experimental status of this study was appropriate for induction of heat stress to impair the calcification process in the uterus and to reduce egg quality. As noted, several ion transporters/channels contribute in the uterine calcification process to form the eggshell (Jonchère et al., Citation2012). Jonchère et al. (Citation2012) identified 33 ion transporters/channels that are involved in the uterine mineralization of the avian eggshell. These uterine ion transporters/channels are categorized into six families (based on their function), according to the ion regulated, including: (1) Ca2+, e.g. TRPV6, CALB1 and ITPR3; (2) Na+, e.g. SCNN1G and SLC4A4; (3) K+, e.g. KCNJ15; (4) HCO3−, e.g. SLC4A4 and SLC4A9; (5) H+, e.g. CLCN2, and (6) Cl−, e.g. CLCN2 and SLC4A9 (Jonchère et al., Citation2012). Jonchère et al. (Citation2012) confirmed that genes of many ion transporters/channels (12 genes) are overexpressed in the uterus of hens and associated with eggshell calcification. These transporters/channels included, for Ca2+: CALB1, ATP2A3, and ATP2B1/2; for Na+: SCNN1G and ATP1A1; for K+: KCNJ2, SLC26A9, KCNJ15, and KCNMA1; for HCO3− (production and transport): SLC26A9; for Cl−: CFTR and SLC26A9. They also reported that expression of the following genes did not change in the uterus of hens with egg production, for Ca2+: TRPV6, ITPR1/2/3, SLC8A1/3 and ATP2A2; for Na+: SCNN1A/B, ATP1B1, SLC8A1/3, SLC4A4/5/10; for HCO3− (production and transport): ATP1B1 and KCNJ16; CA4/7 and SLC4A4/5/10; for Cl−: CLCN2 and CLCN5; for H+: ATP6V1B2. In the present study, 8 genes were evaluated in the heat stress condition while the hens were at the maximum level of their egg production. Heat stress diminished the expression of genes such as ITPR3, SLC4A4 and SLC4A9 in the active uterus. ITPR3 as one of the inositol 1, 4, 5-trisphosphate receptors is an intracellular Ca2+ channel, localized in the endoplasmic reticulum and leading the release of calcium ion from this organelle (Narayanan, Adebiyi, & Jaggar, Citation2012). The decrease of ITPR3 expression may reduce the involvement of the endoplasmic reticulum in intracellular Ca2+ buffering in the uterine glandular cells and down-regulate intracellular calcium (Brionne et al., Citation2014; Jonchère et al., Citation2012). SLC4A4 as a Na+/HCO3− co-transporter causes the electroneutral uptake of Na+ and HCO3− across the basolateral membrane of uterine glandular cells (Gholami, Muniandy, & Salleh, Citation2014). Apparently, this transporter has little importance in eggshell formation because the majority of HCO3− in calcification is supplied from blood CO2 and only a minor fraction of this anion is provided through SLC4A4 (Jonchère et al., Citation2012). However, the decreased expression of this transporter may partially disturb the eggshell calcification in the hen uterus during heat stress. It has been demonstrated that SLC4A9 as a Cl−/HCO3− exchanger, is expressed in the gastric surface mucous and duodenal villus cells to secrete HCO3− into the lumen and neutralize gastric acidity (Xu et al., Citation2003). Our study also confirmed the expression of the SLC4A9 gene in the uterus, supporting the hypothesis of the involvement of this transporter in the supply of HCO3− for eggshell formation. The decrease of this transporter may negatively affect mineralization in the uterus. Jonchère et al. (Citation2012) reported that the SLC4A9 gene is not expressed in the uterus of hens while it was clearly detected and analyzed in the present study. This conflicting result might be related to technical differences and design of the primers. However, our finding confirmed that harmful effects of heat stress in the uterine calcification of the eggshell could partially be due to defect in the expression of many ion transporters/channels.
CALB1 as an intracellular transporter is able to transport Ca2+ through the cell and acts as a Ca2+ sensor and cytoplasmic Ca2+ buffer, preventing intracellular Ca2+ concentrations increasing to toxic levels (O'Toole, Citation2011). CALB1 is expressed widely in different tissues such as uterus, retina, brain, kidney, intestine, bone and pancreas (Geng et al., Citation2015). As mentioned, the uterine expression of this protein is considerably increased during eggshell calcification and is a crucial factor in egg formation. However, CALB1 maintains low intracellular calcium ion level in different cells to avoid cell death and apoptosis. Increase of CALB1 in prostate cancer cells during heat stress has been observed (Li et al., Citation2011) which may relate to its compensatory and protective effects against abnormal mineralization and apoptosis. In the hen uterus, a positive association between CALB1 and egg quality has been confirmed (Bar, Citation2009). In the study of Ebeid et al. (Citation2012), laying hens were exposed to high temperature (30–33 °C) for 4 weeks, then immunohistochemistry was performed to evaluate the amount of CALB1 protein in the uterine glandular cells. They found that this protein was lower in the heat stress group than in the control group. As noted, these findings contrast with our data. This contrast may be due to the duration and temperature range of heat stress. Hence, longer (8 weeks) and more severe (36–38 °C) heat stress may lead the uterine cells to undergo apoptosis, and increasing CALB1 gene expression may protect against this. However, transcription and translation processes are complicated, and increased transcription of a gene does not always increase the production of the related protein.
It must be noted that heat stress is associated with changes in secretion of many hormones such as estrogen, glucocorticoids, and catecholamines. These hormones could differently affect the gene expression of CALB1 in the tissues (Ebeid et al., Citation2012; Lacopino and Christakos, Citation1990; Rozenboim et al., Citation2007). In addition, hypocalcemia and parathyroid hormone can also change the gene expression of CALB1 (Cao, Bolt, Wei, Meyer et al., Citation1992; Sitrin, & Li, Citation2002). Perhaps, our data (increased expression of CALB1) are the resultant of these effects. However, a limitation of the present study is that we did not assess protein expression and localization of the studied mRNAs in the uterus, so further studies are also needed to evaluate post-transcription and post-translation changes of uterine ion transport elements during heat stress.
In summary, our data show that genes for the ion transporters/channels, TRPV6, CALB1, ITPR3, SCNN1G, SLC4A4, KCNJ15, SLC4A9, and CLCN2 are normally expressed in the laying hen uterine tissue. It is proposed that the observed down-expression of some of the genes for ion transporters/channels (ITPR3, SLC4A4 and SLC4A9) impairs mineralization of the eggshell during heat stress. However, up-expression of the CALB1 gene may increase resistance of uterine cells against detrimental effects of heat stress. Practically, improving the expression of the mentioned genes may be helpful in promoting eggshell mineralization during heat stress in laying hens.
Disclosure statement
There are no conflicts of interest that could have inappropriately influenced the study or the reporting of the findings. This work had been supported by the funds granted by the Applied Research Centre, Vice Chancellor for Research of Shahrekord University.
References
- Ahmadipour, B., Hassanpour, H., Asadi, E., Khajali, F., Rafiei, F., & Khajali, F. (2015). Kelussia odoratissima Mozzaf – A promising medicinal herb to prevent pulmonary hypertension in broiler chickens reared at high altitude. Journal of Ethnopharmacology, 159, 49–54. doi:10.1016/j.jep.2014.10.043
- Allahverdi, A., Feizi, A., Takhtfooladi, H.A., & Nikpiran, H. (2013). Effects of heat stress on acid-base imbalance, plasma calcium concentration, egg production and egg quality in commercial layers. Global Veterinaria, 10, 203–207. doi:10.5829/idosi.gv.2013.10.2.7286
- Altan, O., Pabuçcuoğlu, A., Altan, A., Konyalioğlu, S., & Bayraktar, H. (2003). Effect of heat stress on oxidative stress, lipid peroxidation and some stress parameters in broilers. British Poultry Science, 44, 545–550. doi:10.1080/00071660310001618334
- Bagés, S., Estany, J., Tor, M., & Pena, R.N. (2015). Investigating reference genes for quantitative real-time PCR analysis across four chicken tissues. Gene, 561, 82–87. doi:10.1016/j.gene.2015.02.016
- Bar, A. (2009). Differential regulation of calbindin in the calcium transporting organs of birds with high calcium requirements. The Journal of Poultry Science, 46, 267–285. doi:org/10.2141/jpsa.46.267
- Berger, E.Y. (1955). Calcium determination in biologic material. Clinical Chemistry, 1, 249–252.
- Brionne, A., Nys, Y., Hennequet-Antier, C., & Gautron, J. (2014). Hen uterine gene expression profiling during eggshell formation reveals putative proteins involved in the supply of minerals or in the shell mineralization process. BMC Genomics, 15, 220. doi:10.1186/1471-2164-15-220
- Cao, L.P., Bolt, M.J.G., Wei, M., Sitrin, M.D., & Li, Y.C. (2002). Regulation of calbindin-D9k expression by 1,25 dihydroxyvitamin D and parathyroid hormone in mouse primary renal tubular cells. Archives of Biochemistry and Biophysics, 400, 118–124. doi:10.1006/abbi.2002.2775
- De Witt, F.H., Kuleile, N.P., Van Der Merwe, H.J., & Fair, M.D. (2009). Effect of limestone particle size on egg production and eggshell quality of hens during late production. South African Journal of Animal Science, 39, 37–40. doi:org/10.4314/sajas.v39i1.61171
- Ebeid, T.A., Suzuki, T., & Sugiyama, T. (2012). High ambient temperature influences eggshell quality and calbindin-D28k localization of eggshell gland and all intestinal segments of laying hens. Poultry Science, 91, 2282–2287. doi:10.3382/ps.2011-01898
- Geng, Y., Lin, H.T., Chen, W., Liu, Z.C., Xiang, W., & Chen, W.R. (2015). Age-related reduction in calbindin-D28K expression in the Sprague-Dawley rat lens . Molecular Medicine Reports, 11, 422–426. doi:10.3892/mmr.2014.2672
- Gholami, K., Muniandy, S., & Salleh, N. (2014). Modulation of sodium-bicarbonate co-transporter (SLC4A4/NBCe1) protein and mRNA expression in rat's uteri by sex-steroids and at different phases of the oestrous cycle. Research in Veterinary Science, 96, 164–170. doi:10.1016/j.rvsc.2013.11.005
- Hassanpour, H., Afzali, A., Fatemi Tabatabaie, R., Torabi, M., & Alavi, Y. (2016). Cardiac renin-angiotensin system (gene expression) and plasma angiotensin II in chickens with T3-induced pulmonary hypertension. British Poultry Science, 57, 444–450. doi:10.1080/00071668.2016.1162284
- Hassanpour, H., Khosravi Alekoohi, Z., Madreseh, S., Bahadoran, S., & Nasiri, L. (2016). Variation of heat shock protein gene expression in the brain of cold-induced pulmonary hypertensive chickens. British Poultry Science, 57, 636–642. doi:10.1080/00071668.2016.1196340
- Jonchère, V., Brionne, A., Gautron, J., & Nys, Y. (2012). Identification of uterine ion transporters for mineralisation precursors of the avian eggshell. BMC Physiology, 12, 1–17. doi:10.1186/1472-6793-12-10
- Lacopino, A.M., & Christakos, S. (1990). Corticosterone regulates calbindin-D28k mRNA and protein levels in rat hippocampus. The Journal of Biological Chemistry, 265, 10177–10180.
- Lara, L.J., & Rostagno, M.H. (2013). Impact of heat stress on poultry production. Animals: An Open Access Journal from Mdpi, 3, 356–369. doi:10.3390/ani3020356
- Li, Y., Zeng, Y., Mooney, S.M., Yin, B., Mizokami, A., Namiki, M., & Getzenberg, R.H. (2011). Resistance to paclitaxel increases the sensitivity to other microenvironmental stresses in prostate cancer cells. Journal of Cellular Biochemistry, 112, 2125–2137. doi:10.1002/jcb.23134
- Mahmoud, K.Z., Beck, M.M., Scheideler, S.E., Forman, M.F., Anderson, K.P., & Kachman, S.D. (1996). Acute high environmental temperature and calcium-estrogen relationships in the hen. Poultry Science, 75, 1555–1562. doi:org/10.3382/ps.0751555
- Mashaly, M.M., Hendricks, G.L., Kalama, M.A., Gehad, A.E., Abbas, A.O., & Patterson, P.H. (2004). Effect of heat stress on production parameters and immune responses of commercial laying hens. Poultry Science, 83, 889–894. doi:org/10.1093/ps/83.6.889
- Meyer, J., Fullmer, C.S., Wasserman, R.H., Barry, S., Komm, B.S., & Haussler, M.R. (1992). Dietary restriction of calcium, phosphorus, and vitamin D elicits differential regulation of the mRNAs for avian intestinal calbindin-D28k and the 1,25-dihydroxyvitamin D, receptor. Journal of Bone and Mineral Research, 7, 441–448. doi:10.1002/jbmr.5650070412
- Mujahid, A., Yoshiki, Y., Akiba, Y., & Toyomizu, M. (2005). Superoxide radical production in chicken skeletal muscle induced by acute heat stress. Poultry Science, 84, 307–314. doi:org/10.1093/ps/84.2.307
- Narayanan, D., Adebiyi, A., & Jaggar, J.H. (2012). Inositol trisphosphate receptors in smooth muscle cells. American Journal of Physiology. Heart and Circulatory Physiology, 302, H2190–H2210. doi:10.1152/ajpheart.01146.2011
- Narushin, V.G. (2005). Egg geometry calculation using the measurements of length and breadth. Poultry Science, 84, 482–484. doi:org/10.1093/ps/84.3.482
- NRC. (1994). Nutrient requirements of poultry. National Academies Press.
- Nys, Y., Gautron, J., Garcia-Ruiz, J.M., & Hincke, M.T. (2004). Avian eggshell mineralization: Biochemical and functional characterization of matrix proteins. Comptes Rendus Palevol, 3, 549–562. doi:org/10.17226/2114
- O'Toole, J.F. (2011). Disorders of calcium metabolism. Nephron Physiology, 118, p22–p27.
- Puthpongsiriporn, U., Scheideler, S.E., Sell, J.L., & Beck, M.M. (2001). Effects of vitamin E and C supplementation on performance, in vitro lymphocyte proliferation, and antioxidant status of laying hens during heat stress. Poultry Science, 80, 1190–1200. doi:org/10.1093/ps/80.8.1190
- Quinteiro-Filho, W.M., Ribeiro, A., Ferraz-de-Paula, V., Pinheiro, M.L., Sakai, M., Sá, L.R., … Palermo-Neto, J. (2010). Heat stress impairs performance parameters, induces intestinal injury, and decreases macrophage activity in broiler chickens. Poultry Science, 89, 1905–1914. doi:org/10.3382/ps.2010-00812
- Rozenboim, I., Tako, E., Gal-Garber, O., Proudman, J.A., & Uni, Z. (2007). The effect of heat stress on ovarian function of laying hens. Poultry Science, 86, 1760–1765. doi:org/10.3382/ps.2013-03572
- Sahin, K., & Kucuk, O. (2001). Effects of vitamin C and vitamin E on performance, digestion of nutrients and carcass characteristics of Japanese quails reared under chronic heat stress (34 degrees C)). Journal of Animal Physiology and Animal Nutrition, 85, 335–341. doi:10.1046/j.1439-0396.2001.00339.x
- Song, Z., Liu, L., Sheikhahmadi, A., Jiao, H., & Lin, H. (2012). Effect of heat exposure on gene expression of feed intake regulatory peptides in laying hens. Journal of Biomedicine and Biotechnology, 2012. doi:10.1155/2012/484869
- Sonna, L.A., Fujita, J., Gaffin, S.L., & Lilly, C.M. (2002). Invited review: Effects of heat and cold stress on mammalian gene expression. Journal of Applied Physiology (Bethesda, Md. : 1985), 92, 1725–1742. doi:10.1152/japplphysiol.01143.2001
- St-Pierre, N.R., Cobanov, B., & Schnitkey, G. (2003). Economic losses from heat stress by US livestock industries. Journal of Dairy Science, 86, E52–E77. doi:10.3168/jds.S0022-0302(03)74040-5
- Starkie, R.L., Hargreaves, M., Rolland, J., & Febbraio, M.A. (2005). Heat stress, cytokines, and the immune response to exercise. Brain, Behavior, and Immunity, 19, 404–412. doi:10.1016/j.bbi.2005.03.005
- Sun, H., Jiang, R., Xu, S., Zhang, Z., Xu, G., Zheng, J., & Qu, L. (2015). Transcriptome responses to heat stress in hypothalamus of a meat-type chicken. Journal of Animal Science and Biotechnology, 6, 6–12. doi:10.1186/s40104-015-0003-6
- Wang, S.H., Cheng, C.Y., Tang, P.C., Chen, C.F., Chen, H.H., Lee, Y.P., & Huang, S.Y. (2013). Differential gene expressions in testes of L2 strain Taiwan country chicken in response to acute heat stress. Theriogenology, 79, 374–382. doi:10.1016/j.theriogenology.2012.10.010
- Xu, J., Barone, S., Petrovic, S., Wang, Z., Seidler, U., Riederer, B., … Soleimani, M. (2003). Identification of an apical Cl-/HCO3-exchanger in gastric surface mucous and duodenal villus cells. American Journal of Physiology – Gastrointestinal and Liver Physiology, 285, G1225–G1234. doi:10.1152/ajpgi.00236.2003