Abstract
Nitrous oxide (N2O) is a gaseous drug with abuse potential. Despite its common clinical use, little is known about whether N2O administration activates the HPA axis and/or the sympathetic adrenomedullary system. The goal of this study was to determine whether 60% N2O alters plasma concentrations of corticosterone (CORT), epinephrine (EPI), and norepinephrine (NE) in male Long-Evans rats. A gas-tight swivel assembly in the lid of a gas administration chamber allowed the remote collection of blood samples from an indwelling jugular vein catheter at four time-points: baseline and at 30, 60, and 120 min during a two-hour administration of 60% N2O. Relative to baseline, plasma CORT (n = 9) was significantly elevated at all three time-points during N2O inhalation (mixed model analysis, p = .001) and plasma EPI and NE levels were each significantly elevated (n = 8, p ≤ .001) at the 30 min assessment. EPI then declined and did not differ from baseline at the 60 and 120 min assessments (p > .05) whereas NE remained elevated (120 min, p = .001). Administration of 60% N2O increases circulating CORT, EPI, and NE, supporting N2O as a physiological stressor. An N2O-induced increase in CORT is consistent with the observation that addictive drugs typically activate the HPA axis causing increased plasma levels of glucocorticoids. Allostatic models of drug addiction typically involve stress systems and the possible role of stress hormones in N2O-induced allostatic dysregulation is discussed.
Introduction
Nitrous oxide (N2O) is a gaseous drug with a long history of clinical use in medicine and dentistry (Becker & Rosenburg, Citation2008; Sun et al., Citation2015). N2O’s low anesthetic potency limits its use as a sole anesthetic agent, although it is often administered in combination with other drugs as part of a balanced anesthetic approach (Sun et al., Citation2015). It is also administered in clinical situations where achieving anesthesia is not the goal. The anxiolytic and modest analgesic effects of subanesthetic N2O concentrations, when administered as “N2O - oxygen sedation,” can benefit many conscious patients undergoing painful or anxiety-provoking medical and dental procedures (Donaldson, Donaldson, & Quarnstrom, Citation2012). N2O is also an inhalant drug of abuse (Balster, Citation1998; Cousaert, Heylens, & Audenaert, Citation2013; Kaar et al., Citation2016; Layzer, Citation1985; van Amsterdam, Nabben, & van den Brink, Citation2015). N2O has positively reinforcing effects for some humans (Kangas & Walker, Citation2008; Walker & Zacny, Citation2001, Citation2002); is self-administered by monkeys (Grubman & Woods, Citation1981; Wood, Grubman, & Weiss, Citation1977) and rats (Ramsay Watson, Leroux, Prall & Kaiyala, Citation2003; Ramsay et al., Citation2015); and increases dopamine in the nucleus accumbens of rats (Sakamoto et al., Citation2006). The adverse consequences from chronic N2O abuse were recently reviewed (Garakani et al., Citation2016). Reviews of N2O’s pharmacological mechanisms of action are also available (Emmanouil & Quock, Citation2007; Sanders, Weimann, & Maze, Citation2008).
Many drugs of abuse activate the hypothalamic-pituitary-adrenal (HPA) axis (Armario, Citation2010; Stephens & Wand, Citation2012) and numerous reviews address the association between stress and addiction (e.g. Koob et al., Citation2014; Lemieux & al'Absi, Citation2016; Piazza & Deroche-Gamonet, Citation2013). However, little is known about whether N2O activates the HPA axis (e.g. increased plasma levels of glucocorticoids) and/or the sympathetic adrenomedullary (SAM) system (e.g. increased plasma levels epinephrine (EPI) and norepinephrine (NE)). Brandt, Frieling, and Pokar (Citation1988) reported that N2O markedly increased plasma cortisol in conscious human volunteers and they suggested that this might result from N2O-induced excitation or emotional stress. In contrast, Gillman, Katzeff, Vermaak, Becker, and Susani (Citation1988) reported that N2O did not significantly increase plasma cortisol, although there was a significant reduction from baseline levels 10 min after the 60 min N2O administration had ended. However, those subjects received different N2O concentrations (range 33–60% N2O, mean = 48.8% N2O) that were individually titrated to produce “subjective feelings of ‘floating’ and relaxation” (p. 751). Fischer, Gilman, and Blank (1987) administered 80% N2O for 60 min to male Sprague-Dawley rats. The rats were then quickly euthanized and blood was collected. Compared to controls, the N2O group had a three-fold increase in plasma CORT levels.
The present study sought to determine the effect of 60% N2O on plasma CORT, EPI, and NE in rats by collecting blood samples prior to, and at three time-points during, a two-hour N2O administration. Because these hormones are sensitive to the blood collection method as well as to other stressors such as restraint and handling (Vahl et al., Citation2005), the stress of repeated sampling was minimized by collecting samples remotely via flexible tubing connected to a previously placed indwelling jugular vein catheter, allowing rats to move freely without handling during the procedure.
Methods
Subjects
Male Long–Evans rats (n = 9) arrived from Charles River Laboratories weighing approximately 300 g and were housed at 21 ± 1 °C on a 12:12 h light/dark cycle (lights on 07:00–19:00 h) with ad-libitum access to food and water in an AAALAC-accredited animal facility. All procedures were conducted in accordance with an approved protocol from the University of Washington's Institutional Animal Care and Use Committee.
Surgical procedures
While under isoflurane anesthesia, each rat had a single surgery to place an indwelling jugular vein catheter using routine and previously described methods (Al-Noori et al., Citation2008).
Experimental procedures
Following recovery from surgery, rats underwent a habituation procedure in which each rat was placed individually in a clear polycarbonate gas-tight testing chamber (Columbus Instruments, Columbus, OH, internal dimensions 18 x 18 x 31 cm, volume of ∼10 l) with a lid that accommodated an infusion/withdrawal swivel (Instech Laboratories, Plymouth Meeting, PA, Model 375/20) for blood sampling. Habituation sessions, during which rats experienced the same procedures that would take place on the testing day (e.g. handling, familiarization with connection of the blood withdrawal lines, and flushing of catheters to mimic the blood sampling procedure), were performed daily for the 2–3 days before the N2O session.
The N2O test session (≥7 days post-surgery) began at 09:00 h with a two-hour baseline period of control gas (21% oxygen, 79% nitrogen) followed by two-hour (11:00–13:00 h) of N2O (60% N2O, 21% oxygen, 19% nitrogen). The gas flow rate to the chamber was 1.6 l per minute. N2O and oxygen concentrations were measured from the exfluent gas line using an infrared gas analyzer (Normocapoxy, Datex, Helsinki, Finland). Blood samples (1.5 ml per sample) were collected at time t0 (baseline, <10 min prior to N2O delivery) and at t30, t60, and t120 min during N2O delivery. An equal volume of saline replacement was administered intravenously after each of the first three withdrawals to prevent hypovolemia associated with blood collection. Blood sampling and saline replacement were performed remotely via the flexible tubing connected to the jugular vein catheter. Blood for the catecholamine assays (i.e., NE, EPI) was collected in tubes containing EGTA-glutathione (Sigma-Aldrich, St. Louis, MO). CORT samples were collected in tubes containing EDTA plus aprotinin (Sigma-Aldrich, St. Louis, MO). Blood samples were centrifuged and plasma samples were stored at −80 °C until assayed for circulating NE, EPI, and CORT levels. Assays for the first five rats were done using a radioenzymatic assay for determination of plasma EPI and NE and a radioimmunoassay procedure for plasma CORT measurement, respectively. The assays used to analyze the blood samples from these initial five rats were no longer available when the blood samples collected from subsequently tested rats were ready for analysis. Therefore, the assays for the next four rats were performed using commercially available ELISA kits (BA E-5100 for EPI and BA E-5200 for NE, Rocky Mountain Diagnostics, Inc., Colorado Springs, CO; EIA Kit K014-H1 for CORT, Arbor Assays, Ann Arbor, MI). The values obtained using these different assay platforms were comparable () and the data were consequently combined for analysis.
Table 1. Descriptive data according to type of hormone assay: Radioimmunoassay (RIA) for corticosterone (CORT); a Radioenzymatic Assay (RA) for epinephrine (EPI), and norepinephrine (NE); or commercial ELISA kits for CORT, EPI and NE.Table Footnotea
Data analysis
Omnibus tests for the significance of the within-subjects hormone data were conducted with the linear mixed model in SPSS (version 24, IBM Corp., Somers, NY) with comparisons of individual time-points to baseline using LSD confidence interval adjustment. Significance was established at p < .05, two tailed. Data are reported as mean ± SE unless otherwise indicated.
Results
Plasma CORT, EPI, and NE increased significantly above baseline levels during the two-hour administration of 60% N2O (). Plasma EPI returned toward baseline after the 30 min time-point whereas CORT and NE exhibited more sustained increases. Specifically, plasma CORT (n = 9) was significantly elevated above baseline levels at all three time-points during N2O inhalation (mixed model analysis, p = .001). Plasma NE (n = 8) was elevated throughout the 120 min N2O exposure with the values being significantly greater than baseline at 30 and 120 min (p < .001). Plasma EPI (n = 8) was significantly elevated above baseline at the 30 min time-point (p ≤ .001) but did not differ from baseline at the 60 and 120 min time-points (p > .05). The EPI and NE data from a single rat were excluded from the dataset because the assay results were extreme statistical outliers (i.e. the baseline EPI and NE values for this rat were >5 standard deviations above the mean baseline value of the remaining eight rats).
Figure 1. Plasma corticosterone (CORT; n = 9), plasma epinephrine (EPI; n = 8), and norepinephrine (NE; n = 8) at baseline (t = 0 min) and during initial 60% N2O inhalation (t = 30, 60, and 120 min) in rats. Values are mean ± SE and significance was determined by mixed model analysis. Time-points that differ significantly from baseline are indicated above bars. Note that EPI levels resolve more rapidly whereas CORT and NE exhibit more sustained increases.
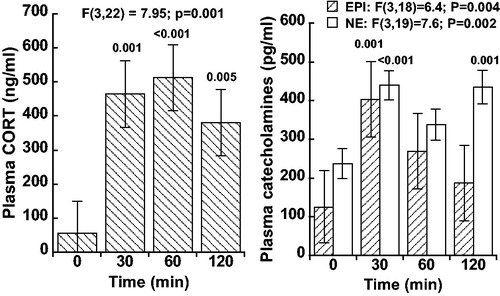
Discussion
Administration of 60% N2O to N2O-naïve male Long-Evans rats significantly increases circulating levels of CORT, EPI, and NE. Both CORT and NE levels remained elevated throughout the two-hour N2O administration. In contrast, although EPI levels were initially increased, they returned toward baseline values during the continued drug administration. Strengths of the current study include repeated blood sampling over time, lack of co-administration of other drugs with N2O, and minimization of other potentially co-occurring stressful procedures or pharmacological effects that could impact hormone levels. A limitation of our study is the lack of a control group to demonstrate that the significant changes from baseline observed during N2O inhalation are not due to factors such as the passage of time in the testing apparatus or the repeated blood sampling method and resulting decrease in erythrocytes.
The current finding that plasma CORT increases during 60% N2O inhalation is congruent with a previous report in which rats were administered 80% N2O (Fischer et al., Citation1987), and with the finding of Brandt et al. (Citation1988) that N2O caused a marked increase in plasma cortisol in conscious human subjects. However, our finding that NE and EPI levels increase during 60% N2O does not fit with Baughman, Hoffman, Miletich, and Albrecht (Citation1990), in which 70% N2O was administered to male Sprague-Dawley rats for 60 min and plasma EPI and NE were measured at 15 and 60 minutes during the N2O exposure. Compared to controls, N2O had no effect on EPI or NE plasma levels at either time point (Baughman et al., Citation1990). However, the rats began testing after two hours of recovery from isoflurane anesthesia and surgical placement of catheters (left ventricle via the right carotid artery, both femoral arteries) and insertion of a rectal thermistor taped to the tail. Thus, the stress associated with recent anesthesia and surgery combined with the presence a rectal probe throughout the N2O administration may have influenced the results.
Our interest in the stress-related neuroendocrine effects of N2O is linked to N2O’s role as a drug of abuse and the potentially pathogenic role that stress systems are believed to play in the etiology of drug addiction. Koob et al. (Citation2014) provide a recent view of stress within an allostatic model of addiction; “… drug addiction represents an excessive and prolonged engagement of homeostatic brain regulatory mechanisms …. This cascade of overactivation of the stress axis represents more than simply a transient homeostatic dysregulation; it also represents a dynamic homeostatic dysregulation that has been termed allostasis” (p. 379). Dysregulation is considered an important attribute of addiction (Koob & Le Moal, Citation2001; Lemieux & al'Absi, Citation2016; Picetti et al., Citation2013) and a common feature of allostasis (Ramsay & Woods, Citation2014). Allostasis (Sterling & Eyer, Citation1988) was originally used in the context of the effects of stress on regulation and contemporary views emphasize the importance of central stress effects and peripheral stress hormones as mediators of allostasis (Ganzel, Morris, & Wethington, Citation2010; McEwen & Wingfield, Citation2010). Glucocorticoids have been specifically implicated as an important factor influencing addictive vulnerability (Blaine & Sinha, Citation2017; Piazza & Deroche-Gamonet, Citation2013; Picetti et al., Citation2013; Stephens & Wand, Citation2012). For example, Koob et al. (Citation2014) have hypothesized that, “activation of the HPA response may be an early dysregulation associated with excessive drug taking” (p. 376). Understanding individual differences in addictive vulnerability is likely to be informed by research on individual differences in stress, a topic hypothesized to receive increasing research attention (Armario, Citation2015). Importantly, preclinical research models have an important role to play in understanding individual differences relevant to psychopathology (Armario & Nadal, Citation2013).
Research involving repeated administrations of N2O to rats provides compelling evidence of drug-induced allostasis development. Dysregulatory overcorrections (sign-reversals) develop while inhaling 60% N2O despite the maintenance of stable N2O concentrations, implying that the observed sign-reversal is not a withdrawal phenomenon (Kaiyala, Chan, & Ramsay, Citation2012; Kaiyala, Woods, & Ramsay, Citation2014; Ramsay Kaiyala, Leroux, & Woods, Citation2005). The dysregulated inefficient concurrent activation of opposing behavioral and autonomic effectors during N2O inhalation gradually develops over repeated N2O administrations (Ramsay et al., Citation2014a). Furthermore, the effect of the motivated behavior offsets the dysregulated overactive autonomic effector activity that is responsible for the sign-reversal thereby providing evidence of the motivational significance of allostasis (Ramsay, Woods, & Kaiyala, Citation2014b). Emphasizing the relevance of drug-induced dysregulation to addiction, individual differences that predict allostasis development to N2O have also been shown to predict individual differences in N2O self-administration (Ramsay et al., Citation2015). It is an open question whether stress hormone changes during N2O administration are involved in the development of N2O-induced allostasis/dysregulation as suggested by the allostatic model of addiction. Considerable evidence supports the view that prolonged or repeated intermittent exposure to stressors can lead to maladaptive dysregulated physiological processes that result in a wide-range of pathologies (Herman, Citation2013). Based on the present findings that N2O increases CORT, NE, and EPI, it is reasonable to consider N2O as a physiological stressor.
The finding that an acute administration of 60% N2O increases CORT, EPI, and NE provides a strong rationale for investigating whether these hormone levels change over repeated N2O administrations. Future research should identify whether changes in any of these hormone levels are associated with the development of N2O-induced allostasis and/or predict individual differences in N2O self-administration.
Acknowledgements
The authors thank the following individuals: Christopher W. Prall for providing technical support during data acquisition and drug delivery; The Taborsky and Lattemann research labs at the VA Puget Sound Health Care System for conducting the initial set of hormone assays; and Ms. Jessie Zou for assisting in the lab during data collection.
Disclosure statement
No potential conflict of interest was reported by the authors.
Additional information
Funding
References
- Al-Noori, S., Sanders, N.M., Taborsky, G.J., Wilkinson, C.W., Zavosh, A., West, C., … Figlewicz, D.P. (2008). Recurrent hypoglycemia alters hypothalamic expression of the regulatory proteins FosB and synaptophysin. American Journal of Physiology-Regulatory, Integrative and Comparative Physiology, 295, R1446–R1454. doi:10.1152/ajpregu.90511.2008
- Armario, A. (2010). Activation of the hypothalamic-pituitary-adrenal axis by addictive drugs: different pathways, common outcome. Trends in Pharmacological Sciences, 31, 318–325. doi:10.1016/j.tips.2010.04.005
- Armario, A. (2015). Studying chronic stress in animals: purposes, models and consequences. In J.A. Russell & M.J. Shipston (Eds.), Neuroendocrinology of Stress (pp. 143–168). Chichester, UK: John Wiley & Sons, Ltd.
- Armario, A., & Nadal, R. (2013). Individual differences and the characterization of animal models of psychopathology: A strong challenge and a good opportunity. Frontiers in Pharmacology, 4, 137. doi:10.3389/fphar.2013.00137
- Balster, R.L. (1998). Neural basis of inhalant abuse. Drug and Alcohol Dependence, 51, 207–214. doi:10.1016/S0376-8716(98)00078-7
- Baughman, V.L., Hoffman, W.E., Miletich, D.J., & Albrecht, R.F. (1990). Cerebrovascular and cerebral metabolic effects of N2O in unrestrained rats. Anesthesiology, 73, 269–272. doi:10.1097/00000542-199008000-00014
- Becker, D.E., & Rosenberg, M. (2008). Nitrous oxide and the inhalation anesthetics. Anesthesia Progress, 55, 124–131. doi:10.2344/0003-3006-55.4.124
- Blaine, S.K., & Sinha, R. (2017). Alcohol, stress, and glucocorticoids: From risk to dependence and relapse in alcohol use disorders. Neuropharmacology, 122, 136–147. doi:10.1016/j.neuropharm.2017.01.037
- Brandt, L., Frieling, B., & Pokar, H. (1988). Plasma cortisol in experimental anesthesia with halothane, enflurane, isoflurane and nitrous oxide. Anaesthesist, 37, 84–90.
- Cousaert, C., Heylens, G., & Audenaert, K. (2013). Laughing gas abuse is no joke. An overview of the implications for psychiatric practice. Clinical Neurology and Neurosurgery, 115, 859–862. doi:10.1016/j.clineuro.2013.04.004
- Donaldson, M., Donaldson, D., & Quarnstrom, F.C. (2012). Nitrous oxide–oxygen administration: When safety features no longer are safe. The Journal of the American Dental Association, 143, 134–143. doi:10.14219/jada.archive.2012.0123
- Emmanouil, D.E., & Quock, R.M. (2007). Advances in understanding the actions of nitrous oxide. Anesthesia Progress, 54, 9–18. doi:10.2344/0003-3006(2007)54[9:AIUTAO]2.0.CO;2
- Fischer, G.J., Gilman, S.C., & Blank, C. (1987). Corticosterone response underlying tolerance to stress induced by a gaseous (nitrous oxide) environment. Perceptual and Motor Skills, 64, 799–808. doi:10.2466/pms.1987.64.3.799
- Ganzel, B.L., Morris, P.A., & Wethington, E. (2010). Allostasis and the human brain: integrating models of stress from the social and life sciences. Psychological Review, 117, 134–174. doi:10.1037/a0017773
- Garakani, A., Jaffe, R.J., Savla, D., Welch, A.K., Protin, C.A., Bryson, E.O., & McDowell, D.M. (2016). Neurologic, psychiatric, and other medical manifestations of nitrous oxide abuse: A systematic review of the case literature. The American Journal on Addictions, 25, 358–369. doi:10.1111/ajad.12372
- Gillman, M.A., Katzeff, I., Vermaak, W.J.H., Becker, P.J., & Susani, E. (1988). Hormonal responses to analgesic nitrous oxide in man. Hormone and Metabolic Research, 20, 751–754. doi:10.1055/s-2007-1010939
- Grubman, J., & Woods, J.H. (1981). Schedule-controlled behavior maintained by nitrous oxide delivery in the rhesus monkey. In S. Saito & T. Yanagita (Eds.), Learning and memory: Drugs as reinforcers (pp. 259–274). International Congress Series 620. Amsterdam: Excerpta Medica.
- Herman, J.P. (2013). Neural control of chronic stress adaptation. Frontiers in Behavioral Neuroscience, 7, 61. doi:10.3389/fnbeh.2013.00061
- Kaar, S.J., Ferris, J., Waldron, J., Devaney, M., Ramsey, J., & Winstock, A.R. (2016). Up: The rise of nitrous oxide abuse. An international survey of contemporary nitrous oxide use. Journal of Psychopharmacology, 30, 395–401. doi:10.1177/0269881116632375
- Kaiyala, K.J., Chan, B., & Ramsay, D.S. (2012). Robust thermoregulatory overcompensation, rather than tolerance, develops with serial administrations of 70% nitrous oxide to rats. Journal of Thermal Biology, 37, 30–40. doi:10.1016/j.jtherbio.2011.10.004
- Kaiyala, K.J., Woods, S.C., & Ramsay, D.S. (2014). Persistence of a hyperthermic sign-reversal during nitrous oxide inhalation despite cue-exposure treatment with and without a drug-onset cue. Temperature, 1, 268–275. doi:10.4161/23328940.2014.944811
- Kangas, B.D., & Walker, D.J. (2008). An adjusting-dose procedure for assessing the reinforcing effects of nitrous oxide with humans. Pharmacology Biochemistry and Behavior, 91, 104–108. doi:10.1016/j.pbb.2008.06.017
- Koob, G.F., Buck, C.L., Cohen, A., Edwards, S., Park, P.E., Schlosburg, J.E., … George, O. (2014). Addiction as a stress surfeit disorder. Neuropharmacology, 76, 370–382. doi:10.1016/j.neuropharm.2013.05.024
- Koob, G.F., & Le Moal, M. (2001). Drug addiction, dysregulation of reward, and allostasis. Neuropsychopharmacology, 24, 97–129. doi:10.1016/S0893-133X(00)00195-0
- Layzer, R.B. (1985). Nitrous oxide abuse. In E. I. Eger II (Ed.), Nitrous oxide/N2O (pp. 249–257). New York, NY: Elsevier Science Publishing Company Inc.
- Lemieux, A., & Al'absi, M. (2016). Stress psychobiology in the context of addiction medicine: From drugs of abuse to behavioral addictions. Progress in Brain Research, 223, 43–62. doi:10.1016/bs.pbr.2015.08.001
- McEwen, B.S., & Wingfield, J.C. (2010). What’s in a name? Integrating homeostasis, allostasis and stress. Hormones and Behavior, 57, 105–111. doi:10.1016/j.yhbeh.2009.09.011
- Piazza, P.V., & Deroche-Gamonet, V. (2013). A multistep general theory of transition to addiction. Psychopharmacology, 229, 387–413. doi:10.1007/s00213-013-3224-4
- Picetti, R., D., Schlussman, S., Zhou, Y., Ray, B., Ducat, E., Yuferov, V., J., & Kreek, M. (2013). Addictions and stress: Clues for cocaine pharmacotherapies. Current Pharmaceutical Design, 19, 7065–7080. doi:10.2174/13816128113199990610
- Ramsay, D.S., Al-Noori, S., Shao, J., Leroux, B.G., Woods, S.C., & Kaiyala, K.J. (2015). Predicting addictive vulnerability: Individual differences in initial responding to a drug’s pharmacological effects. PloS One, 10, e0124740. doi:10.1371/journal.pone.0124740
- Ramsay, D.S., Kaiyala, K.J., Leroux, B.G., & Woods, S.C. (2005). Individual differences in initial sensitivity and acute tolerance predict patterns of chronic drug tolerance to nitrous-oxide-induced hypothermia in rats. Psychopharmacology, 181, 48–59. doi:10.1007/s00213-005-2219-1
- Ramsay, D.S., Watson, C.H., Leroux, B.G., Prall, C.W., & Kaiyala, K.J. (2003). Conditioned place aversion and self-administration of nitrous oxide in rats. Pharmacology Biochemistry and Behavior, 74, 623–633. doi:10.1016/S0091-3057(02)01048-1
- Ramsay, D.S., & Woods, S.C. (2014). Clarifying the roles of homeostasis and allostasis in physiological regulation. Psychological Review, 121, 225–247. doi:10.1037/a0035942
- Ramsay, D.S., Woods, S.C., & Kaiyala, K.J. (2014a). Repeated nitrous oxide exposure in rats causes a thermoregulatory sign-reversal with concurrent activation of opposing thermoregulatory effectors. Temperature, 1, 257–267. doi:10.4161/23328940.2014.944809
- Ramsay, D.S., Woods, S.C., & Kaiyala, K.J. (2014b). Drug-induced regulatory overcompensation has motivational consequences: Implications for homeostatic and allostatic models of drug addiction. Temperature, 1, 248–256. doi:10.4161/23328940.2014.944802
- Sakamoto, S., Nakao, S., Masuzawa, M., Inada, T., Maze, M., Franks, N.P., & Shingu, K. (2006). The differential effects of nitrous oxide and xenon on extracellular dopamine levels in the rat nucleus accumbens: A microdialysis study. Anesthesia & Analgesia, 103, 1459–1463. doi:10.1213/01.ane.0000247792.03959.f1
- Sanders, R.D., Weimann, J., & Maze, M. (2008). Biologic effects of nitrous oxide: A mechanistic and toxicologic review. The Journal of the American Society of Anesthesiologists, 109, 707–722. doi:10.1097/ALN.0b013e3181870a17
- Stephens, M.A.C., & Wand, G. (2012). Stress and the HPA axis: Role of glucocorticoids in alcohol dependence. Alcohol Research: Current Reviews, 34, 468–483.
- Sterling, P., & Eyer, J. (1988). Allostasis: A new paradigm to explain arousal pathology. In S. Fisher & J. T. (Eds.), Handbook of Life Stress, Cognition, and Health (pp. 629–649). New York, NY: Wiley, Chichester.
- Sun, R., Jia, W.Q., Zhang, P., Yang, K., Tian, J.H., Ma, B., … Kuriyama, A. (2015). Nitrous oxide‐based techniques versus nitrous oxide‐free techniques for general anaesthesia. Cochrane Database of Systematic Reviews, (11), CD008984. doi:10.1002/14651858.CD008984.pub2
- Vahl, T.P., Ulrich-Lai, Y.M., Ostrander, M.M., Dolgas, C.M., Elfers, E.E., Seeley, R.J., … Herman, J.P. (2005). Comparative analysis of ACTH and corticosterone sampling methods in rats. American Journal of Physiology-Endocrinology and Metabolism, 289, E823–E828. doi:10.1152/ajpendo.00122.2005
- van Amsterdam, J., Nabben, T., & van den Brink, W. (2015). Recreational nitrous oxide use: Prevalence and risks. Regulatory Toxicology and Pharmacology, 73, 790–796. doi:10.1016/j.yrtph.2015.10.017
- Walker, D.J., & Zacny, J.P. (2001). Within-and between-subject variability in the reinforcing and subjective effects of nitrous oxide in healthy volunteers. Drug and Alcohol Dependence, 64, 85–96. doi:10.1016/S0376-8716(01)00234-9
- Walker, D.J., & Zacny, J.P. (2002). Analysis of the reinforcing and subjective effects of different doses of nitrous oxide using a free-choice procedure. Drug and Alcohol Dependence, 66, 93–103. doi:10.1016/S0376-8716(01)00188-0
- Wood, R.W., Grubman, J., & Weiss, B. (1977). Nitrous oxide self-administration by the squirrel monkey. The Journal of Pharmacology and Experimental Therapeutics, 202, 491–499.