Abstract
Although prior research has characterized stress system reactivity (i.e. hypothalamic–pituitary–adrenal axis, HPAA; autonomic nervous system, ANS) in children, it has yet to examine the extent to which biological reactivity predicts concurrent goal-directed behavior. Here, we employed a stressor paradigm that allowed concurrent assessment of both stress system reactivity and performance on a speeded-response task to investigate the links between biological reactivity and cognitive function under stress. We further investigated gender as a moderator given previous research suggesting that the ANS may be particularly predictive of behavior in males due to gender differences in socialization. In a sociodemographically diverse sample of young children (N = 58, M age = 5.38 yrs; 44% male), individual differences in sociodemographic covariates (age, household income), HPAA (i.e. cortisol), and ANS (i.e. respiratory sinus arrhythmia, RSA, indexing the parasympathetic branch; pre-ejection period, PEP, indexing the sympathetic branch) function were assessed as predictors of cognitive performance under stress. We hypothesized that higher income, older age, and greater cortisol reactivity would be associated with better performance overall, and flexible ANS responsivity (i.e. RSA withdrawal, PEP shortening) would be predictive of performance for males. Overall, females performed better than males. Two-group SEM analyses suggest that, for males, greater RSA withdrawal to the stressor was associated with better performance, while for females, older age, higher income, and greater cortisol reactivity were associated with better performance. Results highlight the relevance of stress system reactivity to cognitive performance under stress. Future research is needed to further elucidate for whom and in what situations biological reactivity predicts goal-directed behavior.
Introduction
Stress-response systems have been evolutionarily shaped to meet the environmental demands of acute stressors, followed by a return to homeostatic stability (McEwen, Citation1998; Flinn, Citation2006). Flexible responding of stress-systems, such as the hypothalamic–pituitary–adrenal axis (HPAA) and the autonomic nervous system (ANS), is a key developmental marker linked to outcomes across cognitive and mental health domains (Blair et al., Citation2011; El-Sheikh et al., Citation2009; Graziano & Derefinko, Citation2013; Granger, Weisz, & Kauneckis, Citation1994). A key gap in this research is the ability to map out how reactivity is linked to adaptive function (e.g. goal-directed behavior) within the stressor context, which holds promise for developing more mechanistic models of adjustment (Obradović, Citation2016).
We examined the extent to which children’s HPAA and ANS reactivity predicted concurrent goal-directed behavior, as measured by cognitive performance on a motivationally-salient matching-task paradigm (Roos et al., Citation2017). We assessed the moderating role of gender, given sexually dimorphic biology and gender socialization of stress-responsivity and emotional reactivity (Chaplin, Cole, & Zahn-Waxler, Citation2005; Chaplin & Aldao, Citation2013; Gallucci et al., Citation1993; Hastings & De, Citation2008; Kumsta et al., Citation2007; Obradović, Citation2016).
ANS function
The SNS branch regulates arousal and resource mobilization (e.g. increased heart rate, blood flow, and HPAA stimulation; Gunnar & Quevedo, Citation2007). The PNS branch allows for rapid heart rate changes through brainstem connections via the vagus nerve (Berntson, Cacioppo, & Quigley, Citation1993a), with flexible engagement theorized to reflect mobilization of attentional resources to meet fluctuating demands (Porges, Citation2007).
The few prior studies examining ANS reactivity and cognitive performance in children have examined either reactivity and cognitive performance in separate tasks (e.g. Skowron, Cipriano‐Essel, Gatzke‐Kopp, Teti, and Ammerman, Citation2014) or reactivity during affectively-neutral tasks (Duschek, Muckenthaler, Werner, & del Paso, Citation2009; Zelazo & Carlson, Citation2012). A meta-analysis found an overall small, but significant, effect of greater PNS withdrawal linked to higher cognitive performance (Graziano & Derefinko, Citation2013). In the singular childhood study of SNS reactivity, greater activation predicted children’s subsequent memory of stressor details (Quas, Yim, Rush, & Sumaroka, Citation2012).
The limited research investigating gender differences in ANS and cognitive function has shown blunted ANS function (both PNS, SNS) to be linked to disinhibited behavior and lower prosocial behavior in males (Beauchaine, Hong, & Marsh, Citation2008; Beauchaine, Citation2001). Given that males tend to exhibit more negative behavior under stress, ANS regulation may be more necessary for goal-directed behavior (Boyce et al., Citation2001; Chaplin & Aldao, Citation2013).
HPAA function
The HPAA responds to demands that necessitate sustained resource mobilization in the context of unpredictable and uncontrollable challenges, with facilitated vigilance having possible beneficial effects on cognitive performance (Del Giudice, Ellis, & Shirtcliff, Citation2011; Sapolsky, Romero, & Munck, Citation2000). However, because engagement is metabolically taxing, there is believed to be a trade-off such that HPAA responsivity is useful for meeting environmental demands, but only for contexts in which the faster-acting (and less costly) ANS response is insufficient (Del Giudice et al., Citation2011).
In the limited research examining links between HPAA reactivity and cognitive performance, some studies have examined reactivity to laboratory visits that involve affectively-neutral cognitive performance tasks with mixed results (e.g. peg-tapping, digit-span, vocabulary knowledge; Blair, Granger, and Razza, Citation2005; Piccolo, Salles, Falceto, Fernandes, and Grassi-Oliveira, Citation2016). Some studies document higher HPAA reactivity predicts lower cognitive performance (Piccolo et al., Citation2016), while others report the opposite (Blair et al., Citation2005). In contexts that are challenging, but not inherently stressful, the link between HPAA reactivity could reflect a misappraisal of resource mobilization needs or child characteristics such as high anxiety. Alternatively, HPAA reactivity could be facilitative of arousal in other tasks. HPAA reactivity to validated stressors has also been linked to higher performance on separate cognitive tasks (e.g. Gunnar, Talge, and Herrera, Citation2009; Quas et al., Citation2012). In such scenarios, HPAA reactivity could be theorized to reflect an accurate appraisal of “stressful” situations or a trait-level ability to allocate resources (metabolic, physiological, cognitive) to meet environmental demands.
There has been minimal research examining gender differences in HPAA functioning in young children. Males have been documented to exhibit higher reactivity than females post-puberty, with no differences pre-puberty (reviewed in Ordaz & Luna, Citation2012). Gender differences have been theorized to reflect a key interaction between the HPAA and sex hormones that increase rapidly during puberty (Young & Altemus, Citation2004). Alternatively, females may respond to stressors with a “befriending” response to maintain social bonds that can involve the release of oxytocin, which downregulates HPAA (Taylor, Citation2006).
The present study
We employed a stressor paradigm that allowed us to concurrently assess HPAA, PNS, and SNS reactivity, in addition to behavioral performance, during a speeded-response task. In this paradigm, children performed a cognitively-challenging matching-task in a limited period of time in order to win a desired prize (Kryski, Smith, Sheikh, Singh, & Hayden, Citation2011; Roos et al., Citation2017). This stressor is designed to push children’s capacity to rapidly execute complex cognitive processes and motor responses thus increasing arousal and resource mobilization, with prior research documenting that children, on average, exhibited HPAA and ANS reactivity (Roos et al., Citation2017).
Because the paradigm was established to elicit stress-system reactivity in a non-clinical sample (Roos et al., Citation2017), it was hypothesized that greater flexibility (i.e. HPAA reactivity, SNS engagement, PNS withdrawal) would be linked to higher performance. ANS reactivity was theorized to be more relevant for males given the aforementioned emotional reactivity differences. For cortisol, reactivity was expected to predict higher performance, with exploratory gender-based analyses. Characterizing how stress-system reactivity predicts concurrent cognitive performance may have important implications for understanding how these systems support children’s function in real-world stressful settings that require maintaining regulated behavior (e.g. academic test-taking, interpersonal conflict).
Methods
A total of 58 children (n = 33 female) and their mothers who were recruited from a socioeconomically diverse community to participate in a study examining links between biology, stress, and behavior. An additional 26 families were recruited as “controls,” but are not reported here, because they did not participate in the social stressor task (described in Roos et al., Citation2017). Participants (aged 4.20–6.71 years, M = 5.38, SD = 0.65) represented a range of household incomes (median = $25,000–$29,999; range <$4999–$100,000+) and maternal education (median = some college or associate’s degree; range < high school-graduate or professional degree), with no significant sociodemographic (age, household income, maternal education) differences between genders (all p > 0.05).
Participants completed one 2-hour laboratory visit (start times 9 am–3 pm; with children awakening a least 2 hours prior and not eating for 1 hour prior). Visits included mother and child watching a 5-minute peaceful ocean video to assess baseline ANS (45 minutes post lab-entry; Piferi, Kline, Younger, and Lawler, Citation2000). The mother then left the room, and children completed one cognitive task (a Go/No-Go task, not reported here) followed by the Stressor (60 minutes post-lab-entry) 10 minutes later and additional assessments (not reported here). Saliva samples were collected following consent and immediately, 20, 40, and 50 minutes post-stressor. At the end of the laboratory visit, children were debriefed about the nature of the stressor task in that they were not given sufficient time to complete the matching task and that they did go fast enough to win their desired prize. Notably, the stressor task had previously been described to parents, and they all consented for their children to participate in the stressful protocol.
Stressor matching task
This stressor was modified from a previous study (Kryski et al., Citation2011) and has been validated (in the present sample) to induce significant HPA and ANS reactivity compared to a control condition with highly similar motor and cognitive demands (described in Roos et al., Citation2017; stressor task materials available upon request). In this paradigm, children played a game in which they were instructed to match colored stickers to transportation types on a worksheet with 30 squares using a key. Prior to beginning the task, the assessor explained the instructions and demonstrated that each sticker of a given color should be matched with a certain transportation type (e.g. orange stickers go with cars, blue goes with boats, etc.) using the “key.” Next, the assessor correctly placed the first sticker on the child’s sheet, and reviewed any questions the child had. After the task began, the assessor could repeat the instructions if the child asked, but did not correct any incorrect sticker placement or confirm that child had placed a sticker correctly. Performance ranged from 5 to 69 (M = 34.69; SD = 15.73) correct trials.
Prior to beginning the task, children picked a desired prize they were told they could win for successfully completing a worksheet. The assessor used flat affect and a non-reinforcing demeanor while also using multiple prompts to increase the socially-evaluative nature including: the phrase that most children your age have no problem completing the task and repeated (2 times per trial) indications of performance failure (e.g. you’re not going fast enough; if you don’t go faster, you won’t earn your prize). Children were given three consecutive two-minute trials (two minutes is insufficient for children of this age to complete the worksheet). All children engaged in the task for the three trials, although some children stopped performing for periods of time. If a child stopped responding, the assessor continued task administration and verbal cues. The assessor operated a stoplight that was set to green (90 seconds), to yellow (30 seconds), and finally to red, accompanied with a loud beep, signaling the end of a trial. After three failures, children were told they failed to earn their prize. Next, the assessor left the room and a friendly, familiar assessor returned. Children received the desired prize at the end of the visit (60 minutes post-stressor).
Cortisol
Salivettes were used to collect saliva (Sarstedt, Inc., Newton, NC), with samples frozen (−20 °C) and sent to University of Trier to be assayed in duplicate [coefficients of variance for inter-assay (7.1–9.0%) and intra-assay (4.0–6.7%)]. Time-from-awakening was calculated as the difference between children’s first cortisol sample and the time they awoke (M = 5.28 hours; SD = 2.91). Six participants were excluded due to refusal, eating/drinking <60 min prior to lab visit, or oral infection, resulting in 52 participants with cortisol data. Missingness was not significantly related to sociodemographic variables or matching task performance.
Cortisol reactivity was calculated by subtracting the participants’ cortisol 20 minutes post-stressor from their baseline levels (assessed 0 minutes post-stressor and established to reflect the lowest, in-laboratory measurement of cortisol ∼60 minutes following lab entry in which there were no differences between stressor and control groups; Roos et al., Citation2017). Extreme values (> 3SDs condition mean; 7 samples out of 260) were winsorized.
Autonomic physiology
An 11-electrode configuration was used for assessment of respiratory sinus arrhythmia [RSA; parasympathetic activity (PNS)] and pre-ejection period [PEP; sympathetic activity (SNS)]. Three electrodes in a lead II arrangement assessed electrocardiogram (ECG). An 8-electrode tetrapolar montage recorded cardiovascular impedance. Data were acquired via Biopac wireless transmitters (Biopac Systems Inc, Goleta, CA). RSA was derived from natural log-transformed values of high frequency (0.24–1.04 Hz) ECG spectral power. PEP was calculated from the first-order derivative of impedance as the Q-to-B interval (Berntson, Lozano, Chen, & Cacioppo, Citation2004).
Data were processed using Mindware software (Gahanna, OH). ECG signals were inspected to confirm heart beats in 30 second epochs. PEP processing involved visual inspection to verify Q and B placement. Epochs were averaged across baseline, first-half matching task, and second-half matching task for RSA and PEP. First-half and second-half matching task were examined separately because stress-inducing qualities (i.e. repeated failure) of the matching task were exaggerated in the second-half. Of 58 participants, 11 participants’ ECG values and 14 participants’ Z0 values were missing due to child electrode refusal or technical problems (< 50% artifact free for each of baseline, first-half matching task, and second-half matching task). This resulted in 47 participants with RSA data and 44 participants with PEP data. Missingness was not significantly related to sociodemographic variables or matching task performance. As previously reported, PEP and RSA reactivity were calculated by subtracting baseline from mean reactivity to the stressor (i.e. mean PEP and mean RSA) during the second-half of the matching task, which was determined to reflect greater stress-system activation, relative to the first-half of the matching task when children were unaware that they were actually performing inadequately (Roos et al., Citation2017).
Analysis plan
First, preliminary bivariate correlational analyses were performed to examine sociodemographic and baseline biological predictors associated with matching task performance. Next, t-tests on both covariate and biological reactivity measures were conducted between genders to examine possible differences linked to cognitive performance. Significant covariate and baseline biological predictors were then incorporated in multivariate structural equation models (SEM) along with measures of biological (i.e. PEP, RSA, Cortisol) reactivity to investigate the relative importance of predictors to matching task performance. Using Mplus 7.0, multigroup (males and females) SEM analyses were performed to examine gender differences in the association between biological predictors and cognitive performance. The built-in full information maximum likelihood function in Mplus allows inclusion of the total sample without losing participants due to listwise deletion.
All paths in the model were allowed to vary across genders, following the hypothesis that there would be gender differences in predictors of cognitive performance. This unconstrained model was compared to a model in which biological predictors of cognitive performance were constrained to be equal across genders. A significant chi-square difference between the unconstrained and constrained model would suggest significant gender differences in links between biological predictors and cognitive performance. All 58 participants were included in SEM analyses and a bootstrapping procedure (10,000 samples) was used as a more conservative estimator of confidence intervals given the limited sample size in the present study (Efron & Tibshirani, Citation1993).
Results
Preliminary analyses
Preliminary analyses examining correlations between matching task performance with sociodemographic (child age, caregiver education, household income), baseline biological (RSA, PEP, cortisol), and biological reactivity variables are presented in . Significant associations with higher matching task performance were found with older child age. Greater cortisol reactivity was also significantly linked to higher matching task performance.
Table 1. Descriptive statistics and bivariate correlations.
Independent sample t-tests revealed no significant gender differences in sociodemographic, biological baseline, or biological reactivity measures (all ps > 0.05; descriptive statistics reported in ). Matching task performance did differ between genders with males [M(SD) = 28.64 (12.23)] performing significantly worse than females [M(SD) = 39.27 (16.69); (t(56) = −2.68, p = 0.01], even after controlling for child age, F(1,55) = 5.10, p = 0.028.
Predictors of matching task performance
Structural equation modeling with bootstrapped confidence intervals was conducted to examine the relative importance of biological reactivity measures (RSA, PEP, cortisol) to matching task performance for males and females, controlling for age. We examined a model in which all paths between predictors and matching task performance were unconstrained across genders (χ2(6) = 5.27, p = 0.51, CFI = 1.00, TLI = 1.03, RMSEA = 0.00). Next, we examined a model in which links between biological reactivity predictors and the outcome of matching task performance were constrained to be equal across males and females (χ2(9) = 13.65, p = 0.14, CFI = 0.87, TLI = 0.89, RMSEA = 0.13). Chi-square difference tests indicated that the unconstrained two-group model was significantly different from the constrained model (Δχ2 (3) = 8.38, p = 0.039), suggesting that there are gender differences in the association between biological predictors and cognitive performance.
In the unconstrained two-group model, matching task performance in males was predicted by greater RSA withdrawal [Estimate(SE) = –10.37(4.16), p = 0.013, 95% CI: –16.14, –2.84)]. In females, higher performance was predicted by greater cortisol reactivity [Estimate(SE) = 4.70(1.86), p = 0.012, 95% CI: 1.92, 7.84]. For illustrative purposes only, the associations of RSA reactivity and cortisol reactivity with cognitive performance are presented in , with results split by gender. Full SEM results from the unconstrained model are presented in .
Figure 1. Predictors of matching task performance, by gender. The visual RSA outlier in the “females” group was not statistically below our 3SD minimum. To ensure this participant was not biasing results, they were temporarily removed, and all results remained consistent.
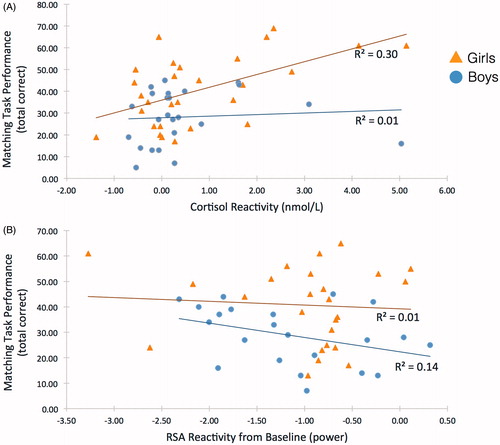
Table 2. Predictors of matching task performance across constrained and unconstrained models.
Discussion
Our findings suggest that children’s flexible engagement of the ANS (PNS branch, specifically) and HPAA components of the biological stress response is predictive of concurrent cognitive performance on a speeded matching task during a stressful laboratory paradigm. Importantly, there were different predictors of matching task performance based on gender. Findings from the two-group analysis indicated that for males, PNS function measured by RSA withdrawal was predictive of performance, while for females, cortisol reactivity was predictive of performance. These findings build on previous research demonstrating that stress-response system reactivity is associated with cognitive performance in separate contexts, indicating that links between ANS and HPAA function with cognition extend beyond shared trait-level regulatory capacity. This suggests that, despite a lack of differences in absolute magnitude of reactivity (generally consistent with previous literature; Graziano & Derefinko, Citation2013; Ordaz & Luna, Citation2012), gender may be an important dimension to consider as a moderator of the relative strength of associations.
The stressful context of the matching task paradigm in which cognitive performance was assessed may help explain why males exhibited overall poorer cognitive performance than females. In early childhood, males tend to have lower levels of frustration tolerance and more externalizing behaviors under stressful conditions, which may have led to deficits in self-regulation, a skill required for effective matching task performance (Chaplin & Aldao, Citation2013). Prior stress research has generally used paradigms (e.g. Trier Social Stress Test, Kirschbaum, Pirke, and Hellhammer, Citation1993) that preclude the evaluation of concurrent cognitive performance or goal-directed behavior, so the consequences of disinhibited responses to social-evaluative stress have not been examined.
An alternate explanation for the observed gender differences is related to the aforementioned tendency of females to “befriend” and build social bonds under stress (Taylor et al., Citation2000). This may have resulted in females being more invested in meeting the socially-affiliative performance expectations of the assessor (i.e. completing a matching task worksheet). Consequently, females may have had higher motivation to persevere in the face of repeated failure and achieve higher performance.
PNS function was significantly predictive of cognitive performance for males. Specifically, greater RSA withdrawal during the matching task was linked to higher performance. This pattern is consistent with prior meta-analytic research demonstrating that RSA withdrawal is linked to higher cognitive function in developmental samples across cognitive, social, and negative mood/stressor paradigms (Graziano & Derefinko, Citation2013). It is also consistent with research that has noted the increased relevance of PNS function for predicting regulated behavior (i.e. fewer disinhibited behavior problems) in males, versus females (Beauchaine et al., Citation2008).
Given that stressful contexts may exaggerate gender differences in emotional reactions (Chaplin & Aldao, Citation2013), males may be particularly dependent on high physiological regulatory capacity to achieve successful goal-directed behavior, despite similar levels of overall PNS reactivity across genders. The early childhood age examined here reflects only the beginning of relatively stable ANS function (Beauchaine, Citation2001); thus, it is also possible that the more limited emotion-regulatory socialization of males may make them particularly vulnerable (Chaplin et al., Citation2005). In contrast to our predictions, sympathetic reactivity was not linked to performance in males and, in fact, longer PEP was marginally predictive of higher performance. However, given that this association is non-significant and only present when controlling for other factors in the SEM, we refrain from substantial interpretation. Given that PEP reactivity has been conceptualized as a marker of both reward sensitivity and approach motivation (Beauchaine & Gatzke-Kopp, Citation2012) and that children were told they could win a prize for their performance in the current task, the lack of association between behavior and PEP values may be due to ceiling effects on SNS reactivity, or confounding influences beyond resource mobilization for speeded responding.
Examinations of cortisol reactivity suggest that HPAA engagement was predictive of higher cognitive performance for females but not males. Prior gender differences research on HPAA function has predominantly quantified mean differences in the magnitude of reactivity between genders, with no evidence for pre-pubertal gender differences (Ordaz & Luna, Citation2012). However, there has been little research on whether links between cortisol reactivity and behavior are moderated by gender. Post-puberty, females tend to exhibit higher negative affect in response to psychosocial stress, despite lower overall cortisol output (Ordaz & Luna, Citation2012). This has been theoretically linked to evolutionary pressures that placed differential demands on the peripheral physiological responses required by stressful situations for males versus females. Females are believed to have faced lower pressure for metabolic resource mobilization linked to HPAA activation (consistent within “tend and befriend” versus fight-or-flight strategies; Taylor, Citation2006). Instead, females are theorized to have developed heightened subjective awareness to stress responsivity (e.g. HPAA activity), which is supported by higher corticolimbic neural reactivity to stress in females (versus males; Ordaz & Luna, Citation2012). Although speculative, we suggest that in our sample, young females’ higher cortisol responses may be linked to higher subjective awareness of the cognitive and psychosocial demands of the matching task stressor, leading to better performance. If this were the case, it is possible that when using a different stressor task in which males were similarly motivated to perform well, cortisol reactivity would be similarly associated with performance across genders. Another possible explanation is that, females with high HPAA flexibility could also have high cognitive capacity leading to a non-mechanistic association between cortisol reactivity and performance.
Limitations of this study include a relatively small sample size in which to examine two-group models of gender differences. However, results from the bootstrapping approach suggest that the estimates were reasonably robust, despite the small sample size. Although the matching task was designed to promote children’s maximal effort in obtaining high performance, differences in strategies (e.g. children with the lowest scores likely withdrew from the task), which are largely unavoidable in behavioral assessments of young children, could have resulted in different engagement of stress-response systems that are not reflective of children’s inherent capacities. Interpreting the direction of effects, such as whether differential biological responsivity facilitates cognitive performance and vice-versa, is also a limitation. It is possible that children who found the matching task easier, and therefore achieved higher performance, experience differential function of stress physiology or different demands on these systems during the stressor paradigm employed here. Finally, the poor performance and associated limited range of cognitive performance in male children could contribute to the lack of association between cognitive performance and cortisol reactivity.
Future research should focus on replicating results regarding differential links between stress system responsivity and concurrent behavior, based on gender. Examining these associations over the course of development could also lend insight into links between biological responsivity and behavior both during and after the pubertal stage. The stressor paradigm employed here is unique in combining challenges of social-evaluation and cognitive demand. Future research might seek to further probe the gender differences in cognitive performance by examining paradigms, or modifying extant paradigms, to manipulate the relative social-evaluation versus cognitive demand portions of the stressor. Future research should also seek to examine the extent to which these results replicate in samples with more racial diversity or in clinical/at-risk samples given that prior meta-analytic research in children suggests links between RSA withdrawal and fewer behavior problems are strongest in samples that are predominantly Caucasian, such as the present sample (Graziano & Derefinko, Citation2013). Finally, a statistical approach of particular interest would be to examine interactions between ANS and HPAA systems or interactions between baseline and reactivity levels across systems; however, we did not have the statistical power to conduct such analyses in the present study (Berntson, Cacioppo, & Quigley, Citation1993b). This approach would help understand the extent to which reactivity of specific stress systems is linked to behavior, versus synchrony or reciprocal activation across systems.
Although we cannot establish a causal link between biological reactivity and cognitive performance, the finding that engagement or disengagement of biological stress-response system components is predictive of concurrent performance suggests that flexible biological reactivity may be facilitative of effective goal-directed behavior under stress. Combined with a growing body of knowledge about anatomical links between biological systems, such knowledge can help us develop a more mechanistic approach to understanding behavior and child adjustment. The current work also highlights the need to examine the potential moderating role of gender on associations between biological responsivity and behavior. We suggest that employing stressor paradigms that permit the assessment of goal-directed behavior will be particularly important to understanding how effective biological responsivity may underlie regulated behavior under stressful conditions. Such research will contribute to a more detailed and increasingly mechanistic knowledge of how and for whom biological responsivity predicts adaptive, goal-directed behavior in contexts characterized by stress.
Disclosure statement
The authors of this manuscript declare no conflicts of interest.
Additional information
Funding
References
- Beauchaine, T. (2001). Vagal tone, development, and Gray’s motivational theory: Toward an integrated model of autonomic nervous system functioning in psychopathology. Development and Psychopathology, 13, 183–214. doi:10.1017/S0954579401002012
- Beauchaine, T.P., & Gatzke-Kopp, L. (2012). Instantiating the multiple levels of analysis perspective in a program of study on externalizing behavior. Development and Psychopathology, 24, 1003–1018. doi:10.1017/S0954579412000508
- Beauchaine, T.P., Hong, J., & Marsh, P. (2008). Sex differences in autonomic correlates of conduct problems and aggression. Journal of the American Academy of Child & Adolescent Psychiatry, 47, 788–796. doi:10.1097/CHI.Ob013e318172ef4b
- Berntson, G.G., Cacioppo, J.T., & Quigley, K.S. (1993a). Respiratory sinus arrhythmia: Autonomic origins, physiological mechanisms, and psychophysiological implications. Psychophysiology, 30, 183–196. doi:10.1111/j.1469-8986.1993.tb01731.x
- Berntson, G.G., Cacioppo, J.T., & Quigley, K.S. (1993b). Cardiac psychophysiology and autonomic space in humans: Empirical perspectives and conceptual implications. Psychological Bulletin, 114, 296–322. doi:10.1037/0033-2909.114.2.296
- Berntson, G.G., Lozano, D.L., Chen, Y.J., & Cacioppo, J.T. (2004). Where to Q in PEP. Psychophysiology, 41, 333–337. doi:10.1111/j.1469-8986.2004.00156.x
- Blair, C., Granger, D.A., Willoughby, M., Mills‐Koonce, R., Cox, M., Greenberg, M.T., … Fortunato, C.K. (2011). Salivary cortisol mediates effects of poverty and parenting on executive functions in early childhood. Child Development, 82, 1970–1984. doi:10.1111/j.1467-8624.2011.01643.x
- Blair, C., Granger, D., & Razza, R.P. (2005). Cortisol reactivity is positively related to executive function in preschool children attending head start. Child Development, 76, 554–567. doi:10.1111/j.1467-8624.2005.00863.x
- Boyce, W.T., Quas, J., Alkon, A., Smider, N.A., Essex, M.J., & Kupfer, D.J. (2001). Autonomic reactivity and psychopathology in middle childhood. British Journal of Psychiatry, 179, 144–150. doi:10.1192/bjp.179.2.144
- Chaplin, T.M., & Aldao, A. (2013). Gender differences in emotion expression in children: A meta-analytic review. Psychological Bulletin, 139, 735–765. doi:10.1037/a0030737
- Chaplin, T.M., Cole, P.M., & Zahn-Waxler, C. (2005). Parental socialization of emotion expression: Gender differences and relations to child adjustment. Emotion, 5, 80–88. doi:10.1037/1528-3542.5.1.80
- Del Giudice, M., Ellis, B.J., & Shirtcliff, E.A. (2011). The adaptive calibration model of stress responsivity. Neuroscience & Biobehavioral Reviews, 35, 1562–1592. doi:10.1016/j.neubiorev.2010.11.007
- Duschek, S., Muckenthaler, M., Werner, N., & del Paso, G.A.R. (2009). Relationships between features of autonomic cardiovascular control and cognitive performance. Biological Psychology, 81, 110–117. doi:10.1016/j.biopsycho.2009.03.003
- Efron, B., & Tibshirani, R.J. (1993). An Introduction to the bootstrap. New York: Chapman and Hall.
- El-Sheikh, M., Kouros, C.D., Erath, S., Cummings, E.M., Keller, P., & Staton, L. (2009). Marital conflict and children’s externalizing behavior: Pathways involving interactions between parasympathetic and sympathetic nervous system activity. Monographs of the Society for Research in Child Development, 74, 1–101. doi:10.1111/j.1540-5834.2009.00501.x
- Flinn, M.V. (2006). Evolution and ontogeny of stress response to social challenges in the human child. Developmental Review, 26, 138–174. doi:10.1016/j.dr.2006.02.003
- Gallucci, W.T., Baum, A., Laue, L., Rabin, D.S., Chrousos, G.P., Gold, P.W., & Kling, M.A. (1993). Sex differences in sensitivity of the hypothalamic-pituitary-adrenal axis. Health Psychology, 12, 420–425. doi:10.1037/0278-6133.12.5.420
- Granger, D.A., Weisz, J.R., & Kauneckis, D. (1994). Neuroendocrine reactivity, internalizing behavior problems, and control-related cognitions in clinic-referred children and adolescents. Journal of Abnormal Psychology, 103, 267–276. doi:10.1037/0021-843X.103.2.267
- Graziano, P., & Derefinko, K. (2013). Cardiac vagal control and children's adaptive functioning: A meta-analysis. Biological Psychology, 94, 22–37. doi:10.1016/j.biopsycho.2013.04.011
- Gunnar, M.R., Talge, N.M., & Herrera, A. (2009). Stressor paradigms in developmental studies: What does and does not work to produce mean increases in salivary cortisol. Psychoneuroendocrinology, 34, 953–967. doi:10.1016/j.psyneuen.2009.02.010
- Gunnar, M., & Quevedo, K. (2007). The neurobiology of stress and development. Annual Review of Psychology, 58, 145–173. doi:10.1146/annurev.psych.58.110405.085605
- Hastings, P.D., & De, I. (2008). Parasympathetic regulation and parental socialization of emotion: Biopsychosocial processes of adjustment in preschoolers. Social Development, 17, 211–238. doi:10.1111/j.1467-9507.2007.00422.x
- Kirschbaum, C., Pirke, K.M., & Hellhammer, D.H. (1993). The ‘Trier Social Stress Test’–a tool for investigating psychobiological stress responses in a laboratory setting. Neuropsychobiology, 28, 76–81. doi:10.1159/000119004
- Kryski, K.R., Smith, H.J., Sheikh, H.I., Singh, S.M., & Hayden, E.P. (2011). Assessing stress reactivity indexed via salivary cortisol in preschool-aged children. Psychoneuroendocrinology, 36, 1127–1136. doi:10.1016/j.psyneuen.2011.02.003
- Kumsta, R., Entringer, S., Koper, J.W., van Rossum, E.F., Hellhammer, D.H., & Wüst, S. (2007). Sex specific associations between common glucocorticoid receptor gene variants and hypothalamus-pituitary-adrenal axis responses to psychosocial stress. Biological Psychiatry, 62, 863–869. doi:10.1016/j.biopsych.2007.04.013
- McEwen, B.S. (1998). Stress, adaptation, and disease. Allostasis and allostatic load. Annals of the New York Academy of Sciences, 840, 33–44. doi:10.1111/j.1749-6632.1998.tb09546.x
- Obradović, J. (2016). Physiological responsivity and executive functioning: Implications for adaptation and resilience in early childhood. Child Development Perspectives, 10, 65–70. doi:10.1111/cdep.12164
- Ordaz, S., & Luna, B. (2012). Sex differences in physiological reactivity to acute psychosocial stress in adolescence. Psychoneuroendocrinology, 37, 1135–1157. doi:10.1016/j.psyneuen.2012.01.002
- Piccolo, L.D.R., Salles, J.F.D., Falceto, O.G., Fernandes, C.L., & Grassi-Oliveira, R. (2016). Can reactivity to stress and family environment explain memory and executive function performance in early and middle childhood? Trends in Psychiatry and Psychotherapy, 38, 80–89. doi:10.1590/2237-6089-2015-0085
- Piferi, R.L., Kline, K.A., Younger, J., & Lawler, K.A. (2000). An alternative approach for achieving cardiovascular baseline: Viewing an aquatic video. International Journal of Psychophysiology, 37, 207–217. doi:10.1016/S0167-8760(00)00102-1
- Porges, S.W. (2007). The polyvagal perspective. Biological Psychology, 74, 116–143. doi:10.1016/j.biopsycho.2006.06.009
- Quas, J.A., Yim, I.S., Rush, E., & Sumaroka, M. (2012). Hypothalamic pituitary adrenal axis and sympathetic activation: Joint predictors of memory in children, adolescents, and adults. Biological Psychology, 89, 335–341. doi:10.1016/j.biopsycho.2011.11.006
- Roos, L.E., Giuliano, R.J., Beauchamp, K.G., Gunnar, M., Amidon, B., & Fisher, P.A. (2017). Validation of autonomic and endocrine reactivity to a laboratory stressor in young children. Psychoneuroendocrinology, 77, 51–55. doi:10.1016/j.psyneuen.2016.11.023
- Sapolsky, R.M., Romero, L.M., & Munck, A.U. (2000). How do glucocorticoids influence stress responses? Integrating permissive, suppressive, stimulatory, and preparative actions 1. Endocrine Reviews, 21, 55–89. doi:10.1210/edrv.21.1.0389
- Skowron, E.A., Cipriano‐Essel, E., Gatzke‐Kopp, L.M., Teti, D.M., & Ammerman, R.T. (2014). Early adversity, RSA, and inhibitory control: Evidence of children's neurobiological sensitivity to social context. Developmental Psychobiology, 56, 964–978. doi:10.1002/dev.21175
- Taylor, S.E. (2006). Tend and befriend biobehavioral bases of affiliation under stress. Current Directions in Psychological Science, 15, 273–277. doi:10.1111/j.1467-8721.2006.00451.x
- Taylor, S.E., Klein, L.C., Lewis, B.P., Gruenewald, T.L., Gurung, R.A., & Updegraff, J.A. (2000). Biobehavioral responses to stress in females: Tend-and-befriend, not fight-or-flight. Psychological Review, 107, 411–429. doi:10.1037/0033-295X.107.3.411
- Young, E.A., & Altemus, M. (2004). Puberty, ovarian steroids, and stress. Annals of the New York Academy of Sciences, 1021, 124–133. doi:10.1196/annals.1308.013
- Zelazo, P.D., & Carlson, S.M. (2012). Hot and cool executive function in childhood and adolescence: Development and plasticity. Child Development Perspectives, 6, 354–360. doi:10.1111/j.1750-8606.2012.00246.x