Abstract
The short (S) allele of a functional polymorphism (5-HTTLPR) within the promoter region of the serotonin transporter gene (SLC6A4) is found to predispose the risk for stress-related affective disorders relative to the long (L) allele. Evidence suggests that elevated stress reactivity of the hypothalamic–pituitary–adrenal (HPA) axis might underlie this association although there is little understanding about the origin of inconsistent findings. Since inadequate sleep is commonly known to promote HPA stress reactivity, it might well play an important modulating role. The present study tested this hypothesis by investigating whether sleep quality moderates the relationship between 5-HTTLPR and cortisol stress responsiveness. From a large 5-HTTLPR database (n = 771), a sample of healthy male and female participants homozygous for either the 5-HTTLPR S-allele (n = 25) or L-allele (n = 25) were assessed for sleep quality and salivary cortisol secretion during acute laboratory stress. Diminished sleep quality was found to exclusively potentiate cortisol stress reactivity in the homozygous L-allele genotype. Accounting for this 5-HTTLPR-dependent influence enhanced the predictive value of 5-HTTLPR on cortisol stress responsiveness, revealing greater HPA reactivity in S-allele relative to L-allele carriers. Current findings suggest that variations in sleep quality may serve as a confounding factor in the search for genetic differences in stress sensitivity and related affective disorders.
1. Introduction
It is well-recognized that stress predisposes the risk for affective disorders (Sapolsky, Citation2015; van Praag, Citation2004) although the psychopathological consequences vary considerably across individuals. There is increasing consensus that genetic variations relate to these interindividual differences (Kendler & Prescott, Citation2006). Particular emphasis has been placed on the serotonin (5-hydroxytryptamine; 5-HT) transporter gene-linked polymorphic region (5-HTTLPR). This functional polymorphism within the promotor region of the serotonin transporter gene (SLC6A4) regulates the expression of the serotonin transporter and comprises an allelic variation that includes a short (S) allele that is associated with lower transcriptional efficacy relative to the long (L) allele (Greenberg et al., Citation1999; Lesch et al., Citation1996).
Following the initial observations (Caspi et al., Citation2003; Hariri et al., Citation2002; Lesch et al., Citation1996), accumulating evidence suggests that the 5-HTTLPR S-allele is associated with an elevated susceptibility for stress-related affective disorders (Uher & McGuffin, Citation2008, Citation2010). However, recent meta-analyses yielded inconclusive results by either providing evidence (Karg, Burmeister, Shedden, & Sen, Citation2011) or reporting no support for this association (Munafò, Durrant, Lewis, & Flint, Citation2009; Risch et al., Citation2009). As a possible explanation for these equivocal findings, it has been suggested that 5-HTTLPR indirectly contributes to depression etiology by influencing the stress sensitivity of the hypothalamic–pituitary–adrenal (HPA) axis (Way & Taylor, Citation2010). This postulation is based on the role of 5-HT in HPA regulation (Fuller, Citation1990; Porter, Gallagher, Watson, & Young, Citation2004) together with the causal involvement of hypercortisolism in the development of depression (Sapolsky, Citation2015; van Praag, Citation2004).
Although multiple studies confirmed that the 5-HTTLPR S-allele potentiates cortisol stress responsiveness (Dougherty, Klein, Congdon, Canli, & Hayden, Citation2010; Gotlib, Joormann, Minor, & Hallmayer, Citation2008; Way & Taylor, Citation2010), this finding has not been consistently replicated (Alexander et al., Citation2009; Bouma, Riese, Nederhof, Ormel, & Oldehinkel, Citation2010; Verschoor & Markus, Citation2011; Wüst et al., Citation2009) and effects in the opposite direction have been reported (Mueller et al., Citation2011). A recent meta-analysis revealed that S-allele carriers on average display higher cortisol responses relative to L-allele carriers, even though the effect size was smaller than anticipated for intermediate biological phenotypes (Miller, Wankerl, Stalder, Kirschbaum, & Alexander, Citation2013). The authors argued that additional moderating variables potentially concealed stronger 5-HTTLPR-dependent effects (Miller et al., Citation2013). Recent insights reveal that sleep quality profoundly influences the stress reactivity of the HPA axis (for review, see, van Dalfsen & Markus, Citation2017). Together with consistent evidence demonstrating the relevance of sleep in the association between 5-HTTLPR and affective symptomatology (Carskadon, Sharkey, Knopik, & McGeary, Citation2012; Hartmann et al., Citation2014; van Roekel, Hartmann, Wichers, & Verhagen, Citation2016), these findings indicate that sleep quality might potentially moderate the relationship between 5-HTTLPR and cortisol stress responsiveness.
The aim of the present study was therefore to determine whether sleep quality moderates the association between 5-HTTLPR and cortisol stress reactivity. Accounting for the potential influence of sleep quality might reveal more consistent 5-HTTLPR-dependent effects, further clarifying the relevance of HPA stress reactivity in the relationship between 5-HTTLPR and affective disorders.
2. Methods
2.1. Participants
Individuals that were included in a recently obtained 5-HTTLPR database of Maastricht University graduates and undergraduates (n = 771) were invited to complete an online screening. The sample that completed the screening questionnaires (n = 366) was carefully screened based on the following inclusion and exclusion criteria. Inclusion criteria comprised: homozygous S’/S’ (S/S, S/Lg, Lg/Lg) or L’/L’ (La/La) 5-HTTLPR genotype. This choice was based on previous findings demonstrating that genetic differences in stress reactivity are most consistently observed when comparing homozygous 5-HTTLPR genotypes (Miller et al., Citation2013). Exclusion criteria encompassed: moderate to severe depressive symptoms (BDI ≥14), current psychiatric diagnosis, contemporary medical condition, medication use, recreational drug use, and excessive alcohol intake (units a week >15). These inclusion and exclusion criteria were met by 196 participants who were invited to participate in the acute stress experiment. The subsequent inclusion was monitored to include an equal distribution of 5-HTTLPR genotypes as well as a similar amount of male and female participants within these groups resulting in the inclusion of the current sample (n = 53).
Initial data examination using the outlier labeling rule (Hoaglin, Iglewicz, & Tukey, Citation1986; Tukey, Citation1977) revealed one outlier concerning sleep quality (PSQI = 9) and was therefore excluded prior to final analyses. Few saliva samples contained too little amount of saliva for the determination of cortisol values. Cortisol parameters could not be computed for these participants (n = 2) and were therefore not incorporated in the statistical analyses. The final sample (n = 50), consisting of S’/S’ (n = 25) and L’/L’ (n = 25) 5-HTTLPR genotypes, included 10 (20%) male and 40 (80%) female participants, aged between 22 and 31 years of age (M = 24.0, SD = 2.0). The present study was conducted according to the guidelines laid down in the Declaration of Helsinki (1975; as revised in 1983). Followed procedures were approved by the Medical Ethics Committee of Maastricht University Medical Centre (Maastricht UMC). Informed consent was obtained from all subjects and participants received a financial compensation for their contribution to the experiment.
2.2. Genotyping
Sterile swabs (Omni Swabs, Whatman, ‘s-Hertogenbosch, the Netherlands) were used to acquire buccal cell samples for 5-HTTLPR determination. Isolation of genomic DNA was performed using QIamp DNA Mini Kits (Qiagen, Leusden, the Netherlands). PCR protocol was followed for the subsequent 5-HTTLPR genotyping (Glatz, Mossner, Heils, & Lesch, Citation2003). Tri-allelic variants were reclassified into a functionally relevant bi-allelic model including S’/S’ (S/S, S/Lg, Lg/Lg) and L’/L’ (La/La), respectively. On the original database (n = 771), Hardy–Weinberg equilibrium (HWE) was determined using χ2 test and revealed that genotype frequencies of S’/S’ (n = 158), S’/L’ (n = 396), and L’/L’ (n = 217) did not differ from the HWE (χ2 = 0.87, p = .35).
2.3. Design and procedure
In order to control for circadian variation in cortisol secretion (Balbo, Leproult, & Van Cauter, Citation2010), experimental sessions were exclusively scheduled in the middle and late afternoon. During the day of the study, participants were instructed to refrain from any caffeinated beverages. Upon arrival, subjects were seated in a waiting room in order to allow cortisol levels to reach normal basal levels. During this resting period, participants completed a general sleep questionnaire (Pittsburgh Sleep Quality Index, PSQI; see 2.4.1). Participants were subsequently guided to a behavioral lab where they were subjected to a psychosocial laboratory stress paradigm (Maastricht Acute Stress Task, MAST; see 2.5). In order to assess stress-related cortisol secretion, salivary cortisol samples were collected at predefined time points: after completing the sleep questionnaire (baseline), immediately before commencing the experimental stress induction (pre-stress), as well as 20 min (post-stress) and 40 min (recovery) after the onset of the laboratory stress procedure.
2.4. Instruments
2.4.1. Sleep quality
Sleep quality was evaluated using the Pittsburgh Sleep Quality Index (PSQI) (Buysse, Reynolds, Monk, Berman, & Kupfer, Citation1989). In order to ensure that the PSQI captured recent sleep quality, the phrasing of the questions was slightly adjusted to assess sleep quality during the past week (instead of the past month). Completing the PSQI requires participants to respond to a variety of statements on a scale ranging from 0 (not during the past week) to 3 (three or more times during the past week). Scoring of the 19 items resulted in 7 component scores: reflecting duration of sleep, sleep disturbance, sleep latency, day dysfunction due to sleepiness, sleep efficiency, overall sleep quality, and medications needed to sleep. The total score (sum of all sub-scores) comprises a general index of sleep quality, where higher scores are indicative of poorer sleep quality. The PSQI is a commonly used and well-validated scale with good psychometric properties (Buysse et al., Citation1989).
2.5. Stress induction
The Maastricht Acute Stress Task (MAST) (Smeets et al., Citation2012) has been developed to capture the most important features of frequently used laboratory stress paradigms by combining both the physical aspects of the Cold Pressor Task (CPT) (Lovallo, Citation1975), and the psychosocial aspects of the Trier Social Stress Task (TSST) (Kirschbaum, Pirke, & Hellhammer, Citation1993). The procedure of the MAST is as follows. After a brief instruction phase (5 min), participants have to perform various cold pressor trials of unpredictable duration (60–90 s) within a constant water temperature (2 °C). In between these trials, participants are instructed to, equivalent to the TSST, perform mental arithmetic (i.e. counting down in steps of 17 starting at a random number) of unpredictable duration (45–90 s). During both tasks, performance is socially evaluated by the experimenter, who additionally provides negative feedback in case of incorrect performance or delayed responding. The alternating trials last for 10 min in total and subsequently participants are led to believe that the second part of the task will start in 5 min. However, after this resting period participants are informed that this was the end of the task. Hence, by encompassing both the lack of control (unpredictable duration) and a potential threat to self-esteem or status (social evaluation), the MAST is thought to capture the key psychological factors mediating the influence of psychological stress on cortisol reactivity (Dickerson & Kemeny, Citation2004). The MAST has been proven to have comparable stress-inducing properties as the TSST. For a more detailed description of the procedure and validation of the MAST, see Smeets et al. (Citation2012).
2.6. Salivary cortisol
2.6.1. Saliva sampling
Cotton swabs (Salivette, Sarstedt, Etten-Leur, the Netherlands) were used to obtain salivary cortisol samples. Immediately after collection, saliva samples were stored at −25 °C up until free cortisol levels were determined using a commercially available luminescence immunoassay (IBL, Hamburg, Germany). Mean intra- and interassay coefficients of variability were respectively lower than 4% and 8%.
2.6.2. Cortisol parameters
Area under the curve (AUC, arbitrary units) was used to determine the amount of cortisol secretion (nmol/L) during the experiment. AUC represents a reliable estimation of total cortisol secretion as it is able to incorporate both the magnitude of the response (intensity) as well as the difference between repeated measurements (sensitivity) within a single outcome measure (Fekedulegn et al., Citation2007). Standardized formulas (Pruessner, Kirschbaum, Meinlschmid, & Hellhammer, Citation2003) were used to calculate the total amount of cortisol secretion during the experiment (AUCg) as well as the stress-induced increase in cortisol levels (AUCi). Hence, since the main objective of the present study was to explore differences in cortisol responses to laboratory stress, AUCi was used as the main outcome measure for statistical analyses.
2.7. Statistical analyses
Statistical analyses were conducted in SPSS (SPSS Statistics 22.0 for Windows, IBM, Armonk, NY, USA). Mean differences between 5-HTTLPR genotype groups (S’/S’ vs. L’/L’) regarding demographic variables and cortisol parameters were evaluated using independent samples t-tests. Hierarchical multiple regression analysis was conducted to examine whether sleep quality moderates the association between 5-HTTLPR and cortisol stress responsiveness. In this regard, 5-HTTLPR genotype (i.e. a two-level categorical variable representing S’/S’ and L’/L’), sleep quality (i.e. a continuous variable representing total PSQI score), and a 5-HTTLPR genotype by sleep quality interaction term were sequentially added as a predictor for stress-induced change in cortisol levels (AUCi). In order to further interpret the results, univariate analysis of variance (ANOVA) was performed with 5-HTTLPR genotype (S’/S’ vs. L’/L’) and sleep quality (high vs. low; median split; Mdn = 3) as between-subject factors on stress-induced increase in cortisol levels (AUCi). This analysis included four subgroups including high sleep quality S’/S’ (n = 18) and L’/L’ (n = 13) as well as low sleep quality S’/S’ (n = 12) and L’/L’ (n = 7), respectively. Significant interaction effects were further interpreted with follow-up analyses. For all analyses, a two-tailed significance level of 5% was adopted. Data are reported in means (M) and SD.
3. Results
3.1. Sample characteristics
displays the sample characteristics in relation to allelic variation in 5-HTTLPR. As demonstrated, no significant differences between the S’/S’ and the L’/L’ group have been observed regarding relevant demographic variables. As indicated in , only baseline cortisol levels (upon arrival) were significantly higher in the L’/L’ (M = 8.48, SD = 3.41) relative to the S’/S’ (M = 6.73, SD = 2.22) group, t(48) = 2.147, p = .038, d = 0.61.
Table 1. Sample characteristics M (SD).
Table 2. Cortisol values (nmol/L).
3.2. Effectiveness of the stress procedure
Repeated measures ANOVA was conducted in order to determine whether the laboratory stress procedure induced a significant cortisol response. Repeated measures ANOVA with time (pre-stress cortisol level vs. post-stress cortisol level) as within-subject factor revealed a significant main effect of time, F(1, 49) = 84.674, p < .001, η2 = 0.633, indicating that post-stress cortisol values (M = 12.5, SD = 4.8) were significantly higher than pre-stress cortisol values (M = 7.0, SD = 2.1).
3.3. Effect of sleep on the relationship between5-HTTLPR and cortisol responsiveness
Hierarchical multiple regression analysis was performed to determine whether sleep quality moderates the association between 5-HTTLPR and cortisol stress responsiveness (AUCi). In this regard, 5-HTTLPR (Model 1), sleep quality (Model 2), and a 5-HTTLPR by sleep quality interaction term (Model 3) were sequentially added as predictor for AUCi (). Analyses revealed that either including 5-HTTLPR as a single predictor (Model 1: F(1, 48) = 2.407, p = .127, R2 = 0.048) or 5-HTTLPR together with sleep quality (Model 2: F(2, 47) = 1636, p = .205, R2 = 0.065) did not significantly predict AUCi. However, adding the 5-HTTLPR by sleep quality interaction term in the final regression model (Model 3: F(3, 46) = 4.231, p = .010, R2 = 0.216) significantly improved predictive capacity (ΔR2 = 0.151, p = .005), producing a regression model that explained 21.6% of the variance in cortisol stress responsiveness (AUCi). As illustrated in , the influence of 5-HTTLPR on cortisol stress responsiveness (AUCi) changes significantly as a function of sleep quality [β = −0.896, t(46) = −2.978, p = .005]. The regression model indicates that while S’/S’ genotypes display significantly greater cortisol stress responsiveness relative to L’/L’ genotypes in the absence of reduced sleep quality [β = 0.964, t(46) = 3.420, p = .001], this pattern gradually changes as a function of sleep quality decrements. Further regression analyses for both genotype groups separately revealed a significant increase in AUCi as a function of lower sleep quality in the L’/L’ group [β = 0.506, t(23) = 2.811, p = .010], whereas the apparent decrease in the S’/S’ group was not statistically significant [β = −0.311, t(23) = −1.570, p = .130].
Figure 1. Stress-related change in salivary cortisol (AUCi) as a function of sleep quality in S’/S’ and L’/L’ 5-HTTLPR genotype. Interaction: p < .01.
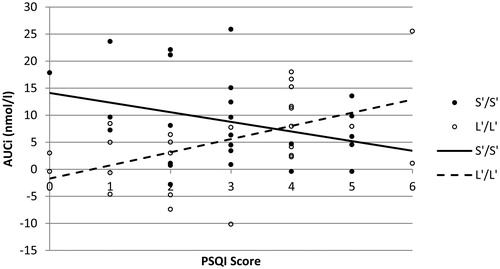
Table 3. Summary of hierarchical regression analysis for variables predicting AUCi.
To further elucidate how sleep quality may alter the association between 5-HTTLPR and cortisol stress responsiveness from a more ecologically valid perspective, an univariate ANOVA was conducted with 5-HTTLPR (S’/S’ vs. L’/L’) and sleep quality (high vs. low) as independent variables on stress-induced cortisol levels (AUCi). Analyses indeed revealed a significant interaction between 5-HTTLPR and sleep quality, F(1, 46) = 10.747, p = .002, η2 = 0.189, indicating that the influence of 5-HTTLPR on cortisol stress responsiveness varied as a function of sleep quality. Further analysis for both sleep quality groups separately revealed a significant main effect of 5-HTTLPR on AUCi in the high sleep quality group, F(1, 29) = 11.477, p = .002, η2 = 0.284, but not in the low sleep quality group, F(1, 17) = 2.393, p = .140, η2 = 0.123. As indicated in , exclusively in the high sleep quality group S’/S’ genotypes displayed higher cortisol stress responses (M = 10.4, SD = 8.8) relative to the L’/L’ group (M = 0.8, SD = 6.0).
3.4. Additional analyses
3.4.1. Control variables
In order to determine whether potential moderating factors influenced current results, the main analyses were repeated with gender, age, or baseline cortisol values as control variable. While gender [β = −0.163, t(45) = −1.252, p = .217] and age [β = 0.181, t(45) = 1.340, p = .187] were not significantly associated with AUCi in the final regression model (Model 3), a significant effect of baseline cortisol was observed [β = −0.359, t(45) = −2.798, p = .008]. However, this was not found to influence the main findings. Including baseline cortisol in the final regression model (Model 3) revealed a similar interaction between 5-HTTLPR and sleep quality [β = −0.946, t(45) = −3.363, p = .002] as well as a significant simple main effect of 5-HTTLPR [β = 0.899, t(45) = 3.406, p = .001]. Similarly, including baseline cortisol values as a covariate in the categorical analyses, F(1, 45) = 6.915, p = .012, η2 = 0.133, also revealed a comparable interaction between 5-HTTLPR and sleep quality, F(1, 45) = 12.920, p = .001, η2 = 0.223.
3.4.2. Outliers
As reported, initial data examination revealed one outlier concerning sleep quality (PSQI = 9) and was therefore excluded in the reported analyses. Including this outlier during statistical analyses did not influence the main findings. Hierarchical multiple regression analyses revealed a similar interaction between 5-HTTLPR and sleep quality [β = −0.987, t(47) = −3.288, p = .002] as well as a simple main effect of 5-HTTLPR [β = 0.933, t(47) = 3.618, p = .001] in the final regression model (Model 3). Correspondingly, univariate ANOVA also produced comparable results revealing a significant interaction between 5-HTTLPR and sleep quality, F(1, 47) = 12.311, p = .001, η2 = 0.208.
4. Discussion
Results of the present study reveal that the relationship between 5-HTTLPR and cortisol stress responsiveness may vary as a function of sleep quality. In conditions of relatively good sleep quality, 5-HTTLPR predicts an elevated cortisol stress reactivity in S’/S’ relative to L’/L’ genotype in accordance with general assumptions. However, as sleep quality declines, 5-HTTLPR-dependent differences in HPA reactivity gradually reduce since this exclusively potentiates cortisol stress responsiveness in L’/L’ carriers.
In line with the established influence of sleep quality on the stress reactivity of the HPA axis (for review, see, van Dalfsen & Markus, Citation2017), the present study demonstrates that not controlling for inadequate sleep may substantially confound the association between 5-HTTLPR and cortisol stress responsiveness. This might be particularly relevant given the frequent inconsistencies among previous investigations (for meta-analysis, see, Miller et al., Citation2013), as variations in sleep quality may apparently conceal more consistent 5-HTTLPR-dependent effects. This is supported by the current observation that a robust effect of 5-HTTLPR on HPA reactivity could only be detected when accounting for the interfering influence of sleep quality. As demonstrated, analyses exclusively revealed a significantly greater cortisol stress responsiveness in S’/S’ relative to L’/L’ genotypes when either statistically controlling for the influence of sleep quality or when analyzing a subsample of participants that experienced relatively good sleep quality. Interestingly, the observed effect size of 5-HTTLPR was relatively large compared to previous investigations, supporting the assumption that accounting for additional moderating factors may enhance the predictive capacity of 5-HTTLPR (Miller et al., Citation2013).
Elevated stress reactivity of the HPA axis has been regarded as an important risk factor for affective disorders (Sapolsky, Citation2015; van Praag, Citation2004). In line with this assumption, greater cortisol stress responsiveness has been suggested as one of the primary mechanisms underlying the elevated depression susceptibility associated with the 5-HTTLPR S-allele (Way & Taylor, Citation2010). Current findings support this assumption by confirming an elevated cortisol responsiveness in S’/S’ relative to L’/L’ carriers when controlling for the influence of sleep quality reductions. However, from this prospective, the 5-HTTLPR L-allele might as well promote depression susceptibility when sleep quality declines. Although the S-allele is commonly regarded as the more susceptible genetic variant (for review, see, Uher & McGuffin, Citation2008, Citation2010), an association between the L-allele and depression has also been repeatedly reported (Chorbov et al., Citation2007; Laucht et al., Citation2009; Ritchie et al., Citation2009; Sjoberg et al., Citation2006; Zhang et al., Citation2009), suggesting that the influence of 5-HTTLPR can be heterogeneous. The present findings suggest that sleep-related alterations in the association between 5-HTTLPR and cortisol stress responsiveness might contribute to such heterogeneity.
Even though elevations in HPA reactivity associated with reduced sleep quality might indeed promote depression susceptibility in L’/L’ carriers, recent studies consistently revealed that the 5-HTTLPR S-allele promotes the negative affective consequences of inadequate sleep (Carskadon et al., Citation2012; Hartmann et al., Citation2014; van Roekel et al., Citation2016). Whereas different mechanisms may be involved, an intriguing possibility might be that the increased cortisol reactivity in L’/L’ carriers actually reflects an adaptive mechanism to cope with the additional demands of reduced sleep quality, whereas the generally elevated cortisol reactivity in S’/S’ carriers might limit such ability. In support for this postulation, increased basal HPA activity following sleep deprivation is generally regarded as an adaptive arousal mechanism (Balbo et al., Citation2010) and, hence, a similar mechanism might account for a stress-induced increase in cortisol levels. The HPA stress reactivity in the L-allele is further found to be more variable over time compared to S-allele carriers (Hankin, Badanes, Smolen, & Young, Citation2015), possibly relating to an increased adaptation to additional environmental influences.
The mechanisms thought to underlie the difference in cortisol stress responsiveness between 5-HTTLPR S-allele and L-allele carriers closely converge with the influence of sleep on these processes. Based on neuroanatomical connections between the amygdala and the HPA axis, it has been proposed that an elevated amygdala reactivity to adverse stimuli observed in S-allele carriers (for meta-analyses, see, Munafò, Brown, & Hariri, Citation2008; Murphy et al., Citation2013) has a central role in promoting cortisol stress responsiveness (Way & Taylor, Citation2010). Since impaired sleep is also found to promote amygdala reactivity (Motomura et al., Citation2013, Citation2014; Simon et al., Citation2015; Yoo, Gujar, Hu, Jolesz, & Walker, Citation2007), it could be argued that 5-HTTLPR and sleep may target shared regulatory mechanisms of the HPA axis. Hence, these findings may be seen as further support for the involvement of sleep in the association between 5-HTTLPR and HPA reactivity, emphasizing the relevance to study these factors in concert.
4.1. Limitations and future directions
The following limitations should be considered. Although the sample size is comparable to the homozygous 5-HTTLPR groups of previous studies exploring the influence of 5-HTTLPR on cortisol stress responsiveness (Alexander et al., Citation2009; Dougherty et al., Citation2010; Gotlib et al., Citation2008; Mueller, Brocke, Fries, Lesch, & Kirschbaum, Citation2010; Way & Taylor, Citation2010), also when performing additional group (Gotlib et al., Citation2008) and correlational (Alexander et al., Citation2009) analyses, larger sample sizes are desirable when replicating current findings. Nonetheless, already observing large and highly significant effects in a relatively small sample may strongly support the assumed associations. Although the sleep assessment included in the present study is a frequently used and well-validated measure, future studies should also incorporate more objective indices of sleep quality (i.e. polysomnography, actigraphy) or include sleep manipulation strategies (i.e. sleep restriction, sleep deprivation). Finally, it remains to be resolved how the current findings translate to real-life stressors and longitudinal studies are needed to elucidate how sleep-related alterations in stress reactivity may influence the development of affective disorders.
5. Conclusions
The present study provides preliminary evidence that sleep quality could play an important modulating role in the relationship between 5-HTTLPR and cortisol stress responsiveness. Together with earlier findings revealing an important role for sleep in the association between 5-HTTLPR and affective symptomatology, current results emphasize the relevance of incorporating sleep quality in future studies. This may enhance the predictive value as well as the interpretation of 5-HTTLPR-dependent effects.
Disclosure statement
The authors report no conflicts of interest.
Funding
This research did not receive any specific grant from funding agencies in the public, commercial, or not-for-profit sectors.
References
- Alexander, N., Kuepper, Y., Schmitz, A., Osinsky, R., Kozyra, E., & Hennig, J. (2009). Gene-environment interactions predict cortisol responses after acute stress: Implications for the etiology of depression. Psychoneuroendocrinology, 34, 1294–1303. doi:10.1016/j.psyneuen.2009.03.017
- Balbo, M., Leproult, R., & Van Cauter, E. (2010). Impact of sleep and its disturbances on hypothalamo-pituitary-adrenal axis activity. International Journal of Endocrinology, 2010, 759234.doi:10.1155/2010/759234
- Bouma, E., Riese, H., Nederhof, E., Ormel, J., & Oldehinkel, A. (2010). No replication of genotype effect of 5-HTTLPR on cortisol response to social stress in larger adolescent sample. Biological Psychiatry, 68, e33–e34. doi:10.1016/j.biopsych.2010.04.041
- Buysse, D.J., Reynolds, C.F. III, Monk, T.H., Berman, S.R., & Kupfer, D.J. (1989). The Pittsburgh Sleep Quality Index: A new instrument for psychiatric practice and research. Psychiatry Research, 28, 193–213. doi:10.1016/0165-1781(89)90047-4
- Carskadon, M.A., Sharkey, K.M., Knopik, V.S., & McGeary, J.E. (2012). Short sleep as an environmental exposure: A preliminary study associating 5-HTTLPR genotype to self-reported sleep duration and depressed mood in first-year university students. Sleep, 35, 791–796. doi:10.5665/sleep.1876
- Caspi, A., Sugden, K., Moffitt, T.E., Taylor, A., Craig, I.W., Harrington, H., … Poulton, R. (2003). Influence of life stress on depression: Moderation by a polymorphism in the 5-HTT gene. Science, 301, 386–389. doi:10.1126/science.1083968
- Chorbov, V.M., Lobos, E.A., Todorov, A.A., Heath, A.C., Botteron, K.N., & Todd, R.D. (2007). Relationship of 5-HTTLPR genotypes and depression risk in the presence of trauma in a female twin sample. American Journal of Medical Genetics Part B: Neuropsychiatric Genetics, 144b, 830–833. doi:10.1002/ajmg.b.30534
- Dickerson, S.S., & Kemeny, M.E. (2004). Acute stressors and cortisol responses: A theoretical integration and synthesis of laboratory research. Psychological Bulletin, 130, 355–391. doi:10.1037/0033-2909.130.3.355
- Dougherty, L.R., Klein, D.N., Congdon, E., Canli, T., & Hayden, E.P. (2010). Interaction between 5-HTTLPR and BDNF Val66Met polymorphisms on HPA axis reactivity in preschoolers. Biological Psychology, 83, 93–100. doi:10.1016/j.biopsycho.2009.10.009
- Fekedulegn, D.B., Andrew, M.E., Burchfiel, C.M., Violanti, J.M., Hartley, T.A., Charles, L.E., & Miller, D.B. (2007). Area under the curve and other summary indicators of repeated waking cortisol measurements. Psychosomatic Medicine, 69, 651–659. doi:10.1097/PSY.0b013e31814c405c
- Fuller, R.W. (1990). Serotonin receptors and neuroendocrine responses. Neuropsychopharmacology, 3, 495–502.
- Glatz, K., Mossner, R., Heils, A., & Lesch, K.P. (2003). Glucocorticoid-regulated human serotonin transporter (5-HTT) expression is modulated by the 5-HTT gene-promotor-linked polymorphic region. Journal of Neurochemistry, 86, 1072–1078. doi:10.1046/j.1471-4159.2003.01944.x
- Gotlib, I.H., Joormann, J., Minor, K.L., & Hallmayer, J. (2008). HPA axis reactivity: A mechanism underlying the associations among 5-HTTLPR, stress, and depression. Biological Psychiatry, 63, 847–851. doi:10.1016/j.biopsych.2007.10.008
- Greenberg, B.D., Tolliver, T.J., Huang, S.J., Li, Q., Bengel, D., & Murphy, D.L. (1999). Genetic variation in the serotonin transporter promoter region affects serotonin uptake in human blood platelets. American Journal of Medical Genetics, 88, 83–87. doi:10.1002/(SICI)1096-8628(19990205)88:1<83::AID-AJMG15>3.0.CO;2-0
- Hankin, B.L., Badanes, L.S., Smolen, A., & Young, J.F. (2015). Cortisol reactivity to stress among youth: Stability over time and genetic variants for stress sensitivity. Journal of Abnormal Psychology, 124, 54–67. doi:10.1037/abn0000030
- Hariri, A.R., Mattay, V.S., Tessitore, A., Kolachana, B., Fera, F., Goldman, D., … Weinberger, D.R. (2002). Serotonin transporter genetic variation and the response of the human amygdala. Science, 297, 400–403. doi:10.1126/science.1071829
- Hartmann, J.A., Wichers, M., van Bemmel, A.L., Derom, C., Thiery, E., Jacobs, N., … Simons, C.J.P. (2014). The serotonin transporter 5-HTTLPR polymorphism in the association between sleep quality and affect. European Neuropsychopharmacology, 24, 1086–1090. doi:10.1016/j.euroneuro.2014.01.015
- Hoaglin, D.C., Iglewicz, B., & Tukey, J.W. (1986). Performance of some resistant rules for outlier labeling. Journal of the American Statistical Association, 81, 991–999. doi:10.1080/01621459.1986.10478363
- Karg, K., Burmeister, M., Shedden, K., & Sen, S. (2011). The serotonin transporter promoter variant (5-HTTLPR), stress, and depression meta-analysis revisited: Evidence. Archives of General Psychiatry, 68, 444–454. doi:10.1001/archgenpsychiatry.2010.189
- Kendler, K.S., & Prescott, C.A. (2006). Genes, environment, and psychopathology: Understanding the causes of psychiatric and substance use disorders. New York, NY: Guilford Publications.
- Kirschbaum, C., Pirke, K.M., & Hellhammer, D.H. (1993). The ‘Trier Social Stress Test’ – A tool for investigating psychobiological stress responses in a laboratory setting. Neuropsychobiology, 28, 76–81. doi:10.1159/000119004
- Laucht, M., Treutlein, J., Blomeyer, D., Buchmann, A.F., Schmid, B., Becker, K., … Banaschewski, T. (2009). Interaction between the 5-HTTLPR serotonin transporter polymorphism and environmental adversity for mood and anxiety psychopathology: Evidence from a high-risk community sample of young adults. The International Journal of Neuropsychopharmacology, 12, 737–747. doi:10.1017/S1461145708009875
- Lesch, K.P., Bengel, D., Heils, A., Sabol, S.Z., Greenberg, B.D., Petri, S., … Murphy, D.L. (1996). Association of anxiety-related traits with a polymorphism in the serotonin transporter gene regulatory region. Science, 274, 1527–1531. doi:10.1126/science.274.5292.1527
- Lovallo, W. (1975). The cold pressor test and autonomic function: A review and integration. Psychophysiology, 12, 268–282. doi:10.1111/j.1469-8986.1975.tb01289.x
- Miller, R., Wankerl, M., Stalder, T., Kirschbaum, C., & Alexander, N. (2013). The serotonin transporter gene-linked polymorphic region (5-HTTLPR) and cortisol stress reactivity: A meta-analysis. Molecular Psychiatry, 18, 1018–1024. doi:10.1038/mp.2012.124
- Motomura, Y., Kitamura, S., Oba, K., Terasawa, Y., Enomoto, M., Katayose, Y., … Mishima, K. (2013). Sleep debt elicits negative emotional reaction through diminished amygdala-anterior cingulate functional connectivity. PLoS One, 8, e56578. doi:10.1371/journal.pone.0056578
- Motomura, Y., Kitamura, S., Oba, K., Terasawa, Y., Enomoto, M., Katayose, Y., … Mishima, K. (2014). Sleepiness induced by sleep-debt enhanced amygdala activity for subliminal signals of fear. BMC Neuroscience, 15, 97. doi:10.1186/1471-2202-15-97
- Mueller, A., Armbruster, D., Moser, D.A., Canli, T., Lesch, K.P., Brocke, B., & Kirschbaum, C. (2011). Interaction of serotonin transporter gene-linked polymorphic region and stressful life events predicts cortisol stress response. Neuropsychopharmacology, 36, 1332–1339. doi:10.1038/npp.2011.11
- Mueller, A., Brocke, B., Fries, E., Lesch, K.P., & Kirschbaum, C. (2010). The role of the serotonin transporter polymorphism for the endocrine stress response in newborns. Psychoneuroendocrinology, 35, 289–296. doi:10.1016/j.psyneuen.2009.07.002
- Munafò, M.R., Brown, S.M., & Hariri, A.R. (2008). Serotonin transporter (5-HTTLPR) genotype and amygdala activation: A meta-analysis. Biological Psychiatry, 63, 852–857. doi:10.1016/j.biopsych.2007.08.016
- Munafò, M.R., Durrant, C., Lewis, G., & Flint, J. (2009). Gene × Environment interactions at the serotonin transporter locus. Biological Psychiatry, 65, 211–219. doi:10.1016/j.biopsych.2008.06.009
- Murphy, S.E., Norbury, R., Godlewska, B.R., Cowen, P.J., Mannie, Z.M., Harmer, C.J., & Munafo, M.R. (2013). The effect of the serotonin transporter polymorphism (5-HTTLPR) on amygdala function: A meta-analysis. Molecular Psychiatry, 18, 512–520. doi:10.1038/mp.2012.19
- Porter, R.J., Gallagher, P., Watson, S., & Young, A.H. (2004). Corticosteroid-serotonin interactions in depression: A review of the human evidence. Psychopharmacology, 173, 1–17. doi:10.1007/s00213-004-1774-1
- Pruessner, J.C., Kirschbaum, C., Meinlschmid, G., & Hellhammer, D.H. (2003). Two formulas for computation of the area under the curve represent measures of total hormone concentration versus time-dependent change. Psychoneuroendocrinology, 28, 916–931. doi:10.1016/S0306-4530(02)00108-7
- Risch, N., Herrell, R., Lehner, T., Liang, K.Y., Eaves, L., Hoh, J., … Merikangas, K.R. (2009). Interaction between the serotonin transporter gene (5-HTTLPR), stressful life events, and risk of depression: A meta-analysis. JAMA, 301, 2462–2471. doi:10.1001/jama.2009.878
- Ritchie, K., Jaussent, I., Stewart, R., Dupuy, A.M., Courtet, P., Ancelin, M.L., & Malafosse, A. (2009). Association of adverse childhood environment and 5-HTTLPR genotype with late-life depression. The Journal of Clinical Psychiatry, 70, 1281–1288. doi:10.4088/JCP.08m04510
- Sapolsky, R.M. (2015). Stress and the brain: Individual variability and the inverted-U. Nature Neuroscience, 18, 1344–1346. doi:10.1038/nn.4109
- Simon, E.B., Oren, N., Sharon, H., Kirschner, A., Goldway, N., Okon-Singer, H., … Hendler, T. (2015). Losing neutrality: The neural basis of impaired emotional control without sleep. Journal of Neuroscience, 35, 13194–13205. doi:10.1523/JNEUROSCI.1314-15.2015
- Sjoberg, R.L., Nilsson, K.W., Nordquist, N., Ohrvik, J., Leppert, J., Lindstrom, L., & Oreland, L. (2006). Development of depression: Sex and the interaction between environment and a promoter polymorphism of the serotonin transporter gene. The International Journal of Neuropsychopharmacology, 9, 443–449. doi:10.1017/S1461145705005936
- Smeets, T., Cornelisse, S., Quaedflieg, C.W.E.M., Meyer, T., Jelicic, M., & Merckelbach, H. (2012). Introducing the Maastricht Acute Stress Test (MAST): A quick and non-invasive approach to elicit robust autonomic and glucocorticoid stress responses. Psychoneuroendocrinology, 37, 1998–2008. doi:10.1016/j.psyneuen.2012.04.012
- Tukey, J.W. (1977). Exploratory data analysis.Reading, Massachusetts: Addison-Wesley Publishing Company.
- Uher, R., & McGuffin, P. (2008). The moderation by the serotonin transporter gene of environmental adversity in the aetiology of mental illness: Review and methodological analysis. Molecular Psychiatry, 13, 131–146. doi:10.1038/sj.mp.4002067
- Uher, R., & McGuffin, P. (2010). The moderation by the serotonin transporter gene of environmental adversity in the etiology of depression: 2009 update. Molecular Psychiatry, 15, 18–22. doi:10.1038/mp.2009.123
- van Dalfsen, J.H., & Markus, C.R. (2017). The influence of sleep on human hypothalamic-pituitary-adrenal (HPA) axis reactivity: A systematic review. Sleep Medicine Reviews, 39, 187–194. doi:10.1016/j.smrv.2017.10.002
- van Praag, H.M. (2004). Can stress cause depression? Progress in Neuro-Psychopharmacology and Biological Psychiatry, 28, 891–907. doi:10.1016/j.pnpbp.2004.05.031
- van Roekel, E., Hartmann, J.A., Wichers, M., & Verhagen, M. (2016). The 5-HTTLPR genotype moderates the association between sleep quality and positive affect: A replication study. European Neuropsychopharmacology, 26, 1350–1351. doi:10.1016/j.euroneuro.2016.06.001
- Verschoor, E., & Markus, C.R. (2011). Effects of acute psychosocial stress exposure on endocrine and affective reactivity in college students differing in the 5-HTTLPR genotype and trait neuroticism. Stress, 14, 407–419. doi:10.3109/10253890.2010.548886
- Way, B.M., & Taylor, S.E. (2010). The serotonin transporter promoter polymorphism is associated with cortisol response to psychosocial stress. Biological Psychiatry, 67, 487–492. doi:10.1016/j.biopsych.2009.10.021
- Wüst, S., Kumsta, R., Treutlein, J., Frank, J., Entringer, S., Schulze, T.G., & Rietschel, M. (2009). Sex-specific association between the 5-HTT gene-linked polymorphic region and basal cortisol secretion. Psychoneuroendocrinology, 34, 972–982. doi:10.1016/j.psyneuen.2009.01.011
- Yoo, S.S., Gujar, N., Hu, P., Jolesz, F.A., & Walker, M.P. (2007). The human emotional brain without sleep – A prefrontal amygdala disconnect. Current Biology, 17, R877–R878. doi:10.1016/j.cub.2007.08.007
- Zhang, K., Xu, Q., Xu, Y., Yang, H., Luo, J., Sun, Y., … Shen, Y. (2009). The combined effects of the 5-HTTLPR and 5-HTR1A genes modulates the relationship between negative life events and major depressive disorder in a Chinese population. Journal of Affective Disorders, 114, 224–231. doi:10.1016/j.jad.2008.07.012