Abstract
The central noradrenaline (NA) stress-response network co-mediates hypothalamic-pituitary-adrenal (HPA) axis activation and arginine-vasopressin (AVP) release. Dysregulation of these systems contributes to stress-related diseases such as human obesity, but their interrelation remains unclear. The study was aimed to test for the first time in vivo whether central noradrenergic activity quantitatively indexed by the availability of the presynaptic NA transporter (NAT) is associated with HPA axis responsiveness as measured with the combined dexamethasone suppression/corticotropin releasing hormone stimulation (dex/CRH) test and copeptin as a surrogate marker of the serum AVP tone in highly obese, otherwise, healthy individuals compared to age- and sex-matched non-obese, healthy controls. In order to assess central NAT availability, positron emission tomography (PET) was applied using the NAT-selective radiotracer S,S-[11C]O-methylreboxetine (MRB) and correlated with curve indicators derived from the dex/CRH test (maximum, MAX, and area under the curve, AUC, for cortisol and adrenocorticotropic hormone, ACTH) as well as with copeptin. In non-obese controls, positive correlations were found between the NAT distribution volume ratios (DVR) of the orbitofrontal cortex (OFC) and the amygdala with the HPA response (OFC: ACTHMAX r = 0.87, p = .001; cortisolMAX r = 0.86, p = .002; amygdala: ACTHMAX r = 0.86, p = .002; cortisolMAX r = 0.79, p = .006), while in obesity, the hypothalamic DVR correlated inversely with the HPA axis response (cortisolMAX, r = −0.66, p = .04) and with copeptin (r = −0.71, p = .02). This association of central NAT availability with HPA axis responsiveness and copeptin suggests a mechanistic interaction between noradrenergic transmission with HPA axis activity and the serum AVP system that differs between non-obese individuals with prefrontal-limbic involvement and obesity with a hypothalamic-centered relationship. Whether the latter finding contributes to obesogenic behavior needs to be further explored.
Trial registration: EU Clinical Trials Register identifier: 2012-000568-32.
1. Introduction
Obesity has reached an epidemic scale; however, its neurobiological underpinnings are not entirely understood and sustained treatment is limited or not available. One key assumption includes stress to promote overeating and to increase the vulnerability to obesity and diet-related metabolic risks (Incollingo et al., Citation2015; Pasquali, Vicennati, Cacciari, & Pagotto, Citation2006). Stressors activate forebrain, limbic, and brainstem structures (Ulrich-Lai & Herman, Citation2009), of which the latter directly extends noradrenergic projections to the paraventricular (PVN) nuclei of the hypothalamus as integrational homeostatic relay of the neuroendocrine stress response systems (Plotsky, Cunningham, & Widmaier, Citation1989; Radant et al., Citation1992). Noradrenergic neurotransmission exerts control over the endocrine axes (Plotsky et al., Citation1989; Zhou, Citation2004) by integrating stress signals at the level of the brainstem cell bodies and noradrenaline (NA) release in the forebrain-limbic and hypothalamic areas (Myers, Scheimann, Franco-Villanueva, & Herman, Citation2017). NA binding to hypothalamic NA receptors triggers corticotropin-releasing hormone (CRH) secretion into the pituitary-portal circulation (Feldman & Weidenfeld, Citation2004) and arginine-vasopressin (AVP) liberation from the neurohypophysis into the peripheral circulation (Liu et al., Citation1994; Radant et al., Citation1992; Simmler, Hysek, & Liechti, Citation2011; Spanakis, Wand, Ji, & Golden, Citation2016). While CRH induces HPA activation, serum AVP mediates water conservation and vascular regulation (Aguilera, Citation2011) with effects on glucose homeostasis and fat metabolism (Saleem et al., Citation2009). Increased activity of the vasopressin system relates to HPA activity (Rothermel et al., Citation2016; Schinke et al., Citation2017) and seems causally linked with obesity (Enhörning et al., Citation2011). Thus, dysregulation of stress-activated neuromodulatory systems that involve (hypothalamic) NA signaling together with hypothalamic-pituitary-adrenal (HPA) axis activity and AVP is thought to be implicated in overeating and the pathophysiology of obesity (Boundy & Cincotta, Citation2000; Incollingo et al., Citation2015; Li et al., Citation2014; Pasquali et al., Citation2006; Plotsky et al., Citation1989).
The NA transporter (NAT) is a critical modulator of noradrenergic transmission since it limits NA concentrations in the synaptic cleft by NA reuptake into the presynaptic neuron (Mandela & Ordway, Citation2006; Torres, Gainetdinov, & Caron, Citation2003) to terminate its action. NAT alterations are implicated in stress-related disorders (Li et al., Citation2014; Moriguchi et al., Citation2017), while NATs represent a major pharmacological target for the treatment of obesity as well (Astrup et al., Citation2008). The pharmacological decrease of NAT availability by NA reuptake inhibitors leads to higher concentrations of NA in the synaptic cleft, which in turn stimulates HPA activity (Schule, Citation2007) and vasopressin release (Simmler et al., Citation2011). Therefore, it is likely that NAT plays a pivotal role in the regulation of these neuroendocrine systems. Understanding perturbations of this interplay between central NA and the neuroendocrine stress response system is particularly important, given that HPA dysregulation and increased AVP tone are linked to obesity and unfavorable physical health conditions (Enhörning et al., Citation2011; Incollingo et al., Citation2015).
In accordance with this, we recently showed that increased HPA axis responsiveness is associated with enhanced concentrations of the AVP-surrogate copeptin in human obesity (Schinke et al., Citation2017). In another study, we furthermore assessed brain NAT availability in both highly obese, otherwise healthy individuals and non-obese, healthy controls by using NAT-selective (S,S)-[11C]O-methylreboxetine ([11C]MRB) positron emission tomography (PET) (Hesse et al., Citation2017). These data indicated a decrease in hypothalamic NAT availability with an increase in body mass index (BMI), which was previously shown to be related to emotional well-being (Melasch et al., Citation2016).
To further investigate whether there is an association of central NAT availability with HPA and AVP activity in human obesity, we applied both PET imaging with [11C]MRB and the combined dexamethasone/CRH (dex/CRH) test for HPA responsiveness (Heuser, Yassouridis, & Holsboer, Citation1994; Schinke et al., Citation2017; Then Bergh, Kumpfel, Trenkwalder, Rupprecht, & Holsboer, Citation1999) in highly obese but otherwise healthy individuals compared to non-obesity, healthy controls which were carefully matched for age and sex. At the same time, we measured copeptin, the c-terminal precursor fragment of vasopressin, as a surrogate of the serum AVP tone (Enhörning et al., Citation2011). We hypothesized that NAT availability in brain regions relevant for stress control, i.e. the prefrontal cortex (PFC), the amygdala, and the hypothalamus (Arnsten, Citation2009), is related to HPA axis activity and copeptin and that these relations regionally differ between individuals with obesity compared to their non-obesity counterparts.
2. Material and methods
2.1. Participants and ethical approval
The study was conducted in accordance with the International Council for Harmonisation of Technical Requirements for Pharmaceuticals for Human Use Guideline for Good Clinical Practice and the declaration of Helsinki and approved by the local ethics committee (registration number 206-10-08032010) and the German Bundesamt für Strahlenschutz/Federal Office for Radiation Protection (Z5-22461-2-2011-002). The study was registered at the European clinical trial database EudraCT 2012-000568-32 and the German Clinical Trials Register (DRKS). Written informed consent was obtained from all participants.
Twenty individuals were prospectively included in the study, which included ten obese, otherwise healthy individuals with a BMI >35 kg/m2 and aged over 18 years. The participants with obesity were recruited from the outpatient clinic of the Integrated Research and Treatment Center AdiposityDiseases (IFB) Leipzig, which is a dedicated university clinic for obesity and associated disorders. Ten non-obese, healthy individuals carefully matched for age and sex and free of any medication or illicit drugs were recruited using flyers and advertisements on the webpage of the IFB (see for subject characteristics). Exclusion criteria for both cohorts were current psychiatric disease, i.e. psychosis, depression, and anxiety disorders. To ensure the psychiatric health, the Structured Clinical Interview for DSM-IV Axis I Disorders (SCID-I) was performed by an experienced psychiatrist during the first visit. In addition, self-rating questionnaires were applied to screen for subthreshold depression and anxiety (Beck Depression Inventory, BDI, German Version, Hautzinger, Citation1991) and the Symptom Checklist-90-Revised version (SCL-90-R, Derogatis, Lipman, & Covi, Citation1973). Binge-eating disorder was excluded based on the use of the Eating Disorder Examination (Hilbert & Tuschen-Caffier, Citation2006). Head trauma or vascular encephalopathy, malignant hypertension, insulin-dependent diabetes, or other general medical conditions that may alter brain function, the use of anorectic medication or other interventions for weight loss, centrally acting medication or nutrition supplements over the last 8 weeks, past or present history of alcohol misuse and/or illicit drug abuse, pregnancy and breastfeeding were also defined as exclusion criteria. The intake of oral contraceptives was not defined as an exclusion criterion and applied in only one female with obesity and normal cortisol suppression after dexamethasone intake. Compared to the group of highly obese participants, the BMI of controls was significantly lower ().
Table 1. Subject characteristics and dex/CRH test indicators.
All study participants underwent a general physical examination, including weight and length measurement for BMI calculation. They also underwent magnetic resonance imaging (MRI) (Magnetom Trio, 3 T, Siemens, Germany; T1-weighted 3 D magnetization prepared rapid gradient echo (MP-RAGE); time of repetition 2300 ms, time of echo 2.98 ms, 176 slices, field of view (FoV) 256 × 240 mm, voxel size 1 × 1 × 1 mm) for PET-MRI co-registration (Hesse et al., Citation2017) and for exclusion of brain pathologies such as diffuse or confluent white matter hyperintensities in T2-weighted images, tumors, or stroke. Dex/CRH test and [11C]MRB PET imaging were performed within a median of 17.5 days.
2.2. Dex/CRH test
The dex/CRH test was performed according to the standard protocols described previously (Heuser et al., Citation1994; Schinke et al., Citation2017; Then Bergh et al., Citation1999). Briefly, all participants received 1.5 mg dexamethasone orally at 2300 h on the day before CRH administration. Subjects were advised to come in a relaxed state and to avoid psychological or physical stress exceeding their daily routine and to have a light lunch before the test. On the test day, an intravenous cannula was inserted into the cubital vein at 1430 h and kept patent by isotonic saline infusion at a rate of 20 ml/h. The first blood sample was taken at 1500 h. At 1502 h, an i.v. bolus of 100 µg of synthetic human CRH (Ferring, Kiel, Germany) was applied. Subsequent blood samples were taken at 1530 h, 1545 h, 1600 h, and 1615 h. The filled tubes were stored at 4 °C, centrifuged immediately after the test, serum and plasma, respectively, were taken off and samples were stored at -80 °C until assayed. Copeptin was measured in the 1500 h sample after dexamethasone ingestion the night before, prior to CRH application. Sodium concentrations and plasma osmolality were assessed. Copeptin, cortisol, osmolality, and sodium concentrations were measured in serum; ACTH concentrations in EDTA plasma.
2.3. Assay methodology
Commercial chemiluminescence immunoassays were used to determine ACTH (Liaison® ACTH, DiaSorin, Saluggia, Italy), and cortisol (Cobas Cortisol I®, Roche, Basel, Switzerland) concentrations. Respective intra- and inter-assay coefficients of variation (CV) for ACTH were below 7.7% for a target value of 9.53 pmol/L and below 7.3% for a target value of 62.3 pmol/L. Representative intra- and interassay CVs for cortisol were below 3.2% for a target value of 86.2 nmol/L and below 2.0% for a target value of 1120 nmol/L. The functional sensitivity of 20% CV was set to be 0.84 pmol/L for ACTH and 8.5 nmol/L for cortisol, according to the manufacturer’s instruction. Copeptin concentrations were measured with Brahms CopeptinUs® (ThermoScientific, Hennigsdorf, Germany) with a lower detection limit of 0.9 pmol/l, an intra-assay coefficient of variation (CV) of <15% and inter-assay CV <17% in the range of 3–4 pmol/l, according to the manufacturer’s instruction.
2.4. Radiotracer synthesis and PET imaging
[11C]MRB was synthesized with [11C]methyliodide ([11C]MeI) as previously described (Hesse et al., Citation2017). Dynamic PET was performed between 1000 h and 1200 h after intravenous bolus injection (90 sec) of 359 ± 11 MBq [11C]MRB (average injected mass: 0.027 ± 0.023 μg/kg) using the ECAT EXACT HR + scanner in three-dimensional acquisition mode (Siemens, Erlangen, Germany; intrinsic resolution at the center 4.3 mm (full-width at half maximum, FWHM), axial resolution: 5–6 mm, field of view: 15.5 cm). Emission scan duration was 120 min acquiring 26 frames (4 × 0.25, 4 × 1, 5 × 2, 5 × 5, 8 × 10 min). Immediately before the application of the radiotracer, a 10-min-transmission scan (from three 68Ge/Ga sources) was performed for attenuation correction and iterative data reconstruction was applied (Hesse et al., Citation2017).
2.5. Imaging data processing
For PET data processing, individual MRI data sets of the subjects were spatially reoriented onto a standard brain dataset similar to the Talairach space using the image processing software PMOD version 3.3 (PMOD Technologies, Zurich, Switzerland). Hereafter, volumes of interest (VOIs) were manually drawn atlas-based on consecutive transversal slices of the reoriented individual MRI data sets by the consensus of two experienced readers. The VOI set included the NAT-rich thalamus, the hypothalamus, and the LC, but also regions of moderate-to-low NAT density of the prefrontal-limbic brain, which are the orbitofrontal cortex (OFC), the insula, the hippocampus, and the amygdala. PET data were corrected for head motion artifacts with the help of SPM2 software (Statistical Parametric Mapping; Wellcome Trust Centre for Neuroimaging, London, UK) and co-registered to the individual MRI including VOIs (). Corresponding tissue time activity curves (TACs) were obtained from the dynamic PET data via PMOD and kinetic modeling of these regional brain TACs was performed using the multilinear reference tissue models MRTM2 (2 parameters) with the occipital cortex as a reference region for the calculation of the DVR (Hesse et al., Citation2017).
2.6. Statistical analysis
PASW/SPSS 25 was used for statistical analysis. Graphs were created with GraphPad Prism 5 (La Jolla, USA). All data are given as median with interquartile range or mean ± standard deviation (SD). After excluding asymmetries of corresponding brain regions, DVR was averaged side-by-side to reduce the number of variables and multiple comparisons. For statistical analysis of the dex/CRH test results, “post-CRH” concentrations for ACTH and cortisol (30 min after CRH application), maximum concentration (MAX) and area under the time course curve above zero according to the trapezoid rule (“ground” area-under-the-curve; AUC) were calculated from the plasma hormone concentrations measured at the five time points mentioned above and shown in . In addition, ACTH/cortisol ratios were computed for each indicator. The Shapiro–Wilk test was performed to test if data were normally distributed and yielded p < .05 for all neuroendocrine data. Hence, relationships between DVR and dex/CRH test parameters, i.e. ACTH and cortisol MAX and AUC, respectively, were analyzed using Spearman-rank correlation for categorical data. To correct the putative DVR-copeptin correlation for sodium and osmolality, copeptin data were logarithmically transformed so they reached normal distribution (Shapiro–Wilk test: p = .5). Then, partial correlation was applied, correcting for sodium and osmolality as covariates. Two-tailed significance was applied. The Mann–Whitney U-test (not-normally distributed data) or unpaired t-test (data with normal distribution) was conducted for group comparison. Results were considered significant at p < .05.
Figure 2. HPA responsiveness in the course of time. Obesity group vs. non-obesity controls. Time course of ACTH (A) and cortisol response (B) to the combined dex/CRH test in subjects with obesity (N = 10; solid line, squares) and non-obesity controls (N = 10; dashed line, circles). After 1.5 mg dexamethasone, taken orally at 2300 h, the night before the test, a bolus of 100 ug CRH was applied i.v. at 1502 h. Data are given as mean with standard error. Dex: dexamethasone; CRH: corticotropin-releasing hormone; ACTH: adrenocorticotropic hormone.
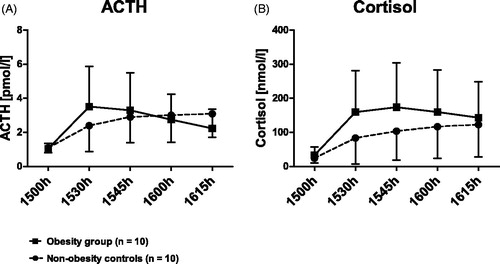
3. Results
3.1. Group statistics
Individuals with obesity and non-obese participants did not differ in demographical variables (with the exception of BMI and waist circumference), see .
3.2. Individuals with obesity exhibit a tendency to higher HPA responsiveness and copeptin concentrations
shows the time course of ACTH and cortisol response to the combined dex/CRH. On a group level, the obesity group tended to show higher cortisol secretion and post-dexamethasone copeptin concentrations, and lower ACTH/cortisol ratios, albeit not reaching statistical significance ().
3.3. Correlative analyses revealed associations between NAT DVR of selected brain areas with the stress response, which are different between obese and non-obese individuals
In non-obesity controls, NAT DVRs of the OFC and the amygdala, but not in the hypothalamus or the midbrain, showed significant positive correlations with neuroendocrine stress test indicators (; ). This includes both the ACTH and the cortisol response (MAX, AUC). No association between NAT and copeptin was found in non-obese controls. In the obesity group, there was no association between prefrontal and limbic NAT, respectively. Instead, hypothalamic NAT correlated negatively with cortisol and copeptin (, ). A moderate negative association between NAT and copeptin was also found for the hippocampus. No other significant correlations were detected. The associations between hypothalamic NAT and copeptin remained significant after correcting for sodium concentrations and osmolality (r = −0.79, p = .02).
Figure 3. Non-obesity controls. NAT DVR in relation to HPA axis responsiveness and serum copeptin. Noradrenaline transporter distribution volume ratios and neuroendocrine parameters in non-obesity controls. Spearman-rho and p-value given for significant correlations. Data are presented in ranks with regression line and 95% confidence interval. MAX: maximum; ACTH: adrenocorticotropic hormone. Significant positive correlations were found between noradrenaline transporter (NAT) distribution volume ratios (DVR) of the orbitofrontal cortex (OFC) and the amygdala with ACTH and cortisol maxima (D, E, G, H). No correlation of copeptin with NAT DVR was found (C, F, I).
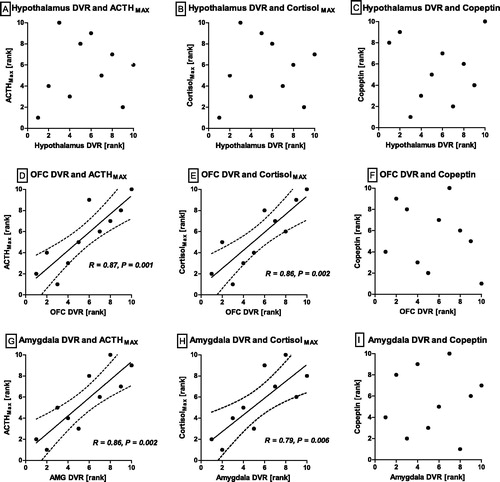
Figure 4. Obesity group. NAT DVR in relation to HPA responsiveness and serum copeptin. Noradrenaline transporter distribution volume ratios and neuroendocrine parameters in the obesity group. Spearman-rho and p-value given for significant correlations. Data are presented as ranks with regression line and 95% confidence interval. MAX: maximum; ACTH: adrenocorticotropic hormone. Significant negative correlations were found between noradrenaline transporter (NAT) distribution volume rations (DVR) of the hypothalamus with cortisol maximum (B) and copeptin, measured after dexamethasone ingestion, prior to CRH administration (C). No correlations between the neuroendocrine parameters with NAT DVR of the orbitofrontal cortex (OFC) or amygdala DVR were found (D–I).
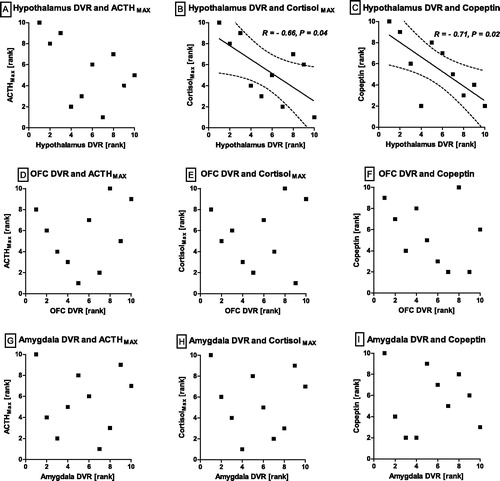
Table 2. Spearman correlation of dex/CRH test indicators and noradrenaline transporter availability.
4. Discussion
The current study demonstrates for the first time in vivo a putative association between regional noradrenergic activity as measured by means of NAT-selective [11C]MRB PET with neuroendocrine stress response indicators. These data add value to our previous findings of an increased HPA axis responsiveness in highly obese individuals, which was related to higher concentrations of the AVP-surrogate copeptin in human obesity (Schinke et al., Citation2017). These first data on an in vivo association between NAT availability with HPA axis parameters and the AVP system indicate that in non-obese controls, noradrenergic activity in the prefrontal-limbic brain (that is, in the OFC and the amygdala) is positively related to stress responsivity while in obese individuals, we observed that noradrenergic activity of the hypothalamus is negatively associated with HPA axis responsiveness and copeptin. This observed pattern of a distinct neuroendocrine stress adaptation suggests a switch from forebrain (prefrontal) and limbic NAT-HPA associations towards a bottom-up regulation primarily involving the hypothalamic neural-NA system together with HPA and neuro-hypophyseal axes regulation (Bains, Wamsteeker Cusulin, & Inoue, Citation2015; Arnsten, Citation2009).
4.1. Potential role of hypothalamic NA(T) in the regulation of the activity of the HPA axis and the vasopressin system
Hence, the present work supports both a physiological and pathophysiological role of the noradrenergic system in the regulation of the HPA axis and the vasopressin system in obesity and potentially in other stress-related diseases. Given that the activation of the neuroendocrine stress systems involves overlapping circuits of the limbic forebrain, the brainstem, and the hypothalamus (Ulrich-Lai & Herman, Citation2009), the positive association between prefrontal-limbic NAT availability with the HPA axis response in non-obese, healthy individuals is in line with a top-down control of HPA axis activity mediated by the forebrain and limbic system. The prefrontal brain is extensively connected with subcortical structures inhibiting HPA axis responses by limiting glucocorticoid secretion (Aihara et al., Citation2007; Arnsten, Citation2009; Ulrich-Lai & Herman, Citation2009) while the amygdala presumably mediates HPA axis responsiveness via intervening hypothalamus-projecting neurons (Ulrich-Lai & Herman, Citation2009).
The hypothalamus is a central homeostatic control region for both the regulation of weight and the neuroendocrine axes (Farr, Li, & Mantzoros, Citation2016; Radant et al., Citation1992) that has dense noradrenergic innervation. As shown by preclinical experiments, hypothalamic noradrenergic activity is closely related to energy expenditure, feeding behavior, and the pathogenesis of obesity (Boundy & Cincotta, Citation2000; Nelson, Gehlert, & Gehlert, Citation2006; Paeger et al., Citation2017; Robertson et al., Citation2010). These hypothalamic centers stimulate ACTH release to trigger the adrenal production of cortisol (Aguilera, Citation2011). Furthermore, hypothalamic neurons contain AVP, which is transported axonally into the posterior pituitary and released into the systemic circulation in response to stress (Aguilera, Citation2011; Katan et al., Citation2009), but also stimulates HPA axis activity (Keck et al., Citation2002; Sivukhina & Jirikowski, Citation2016). Alterations of these endocrine stress systems have been frequently associated with obesity and health impairment (Enhörning et al., Citation2011; Incollingo et al., Citation2015; Pasquali et al., Citation2006). However, there is still an inconsistency in the literature on the relation of cortisol to metabolic parameters (Abraham, Rubino, Sinaii, Ramsey, & Nieman, Citation2013). This is partly based on the variety of methodological approaches and the high inter-individual variability of cortisol secretion (Incollingo et al., Citation2015). Further, peripheral determinants of cortisol concentration such as the activity of the 11-beta-hydroxysteroid dehydrogenases 1 and 2, enzymes converting active cortisol into inactive cortisone and vice versa, contribute to the complex regulation of circulating cortisol concentrations (Bailey, Citation2017; Cooper & Stewart, Citation2009). While basal hypercortisolism is not supported by the majority of the literature (Abraham et al., Citation2013; Bailey, Citation2017; Incollingo et al., Citation2015), it seems that rather alterations of HPA reactivity in response to different kinds of stressors are associated especially to abdominal obesity (Bjorntorp & Rosmond, Citation2000; Incollingo et al., Citation2015; Schinke et al., Citation2017; van der Valk, Savas, & van Rossum, Citation2018).
4.2. Relation of NAT to noradrenergic function and an approach to explain the inverse relation to the HPA axis and copeptin
Considering the positive relation between NAT availability and HPA axis responsiveness in non-obese controls in contrast to absent or, respectively, negative relations in obese individuals, the following assumptions can be made: (1) Normally, NAT density depends on synaptic NA concentration in a homeostatic attempt to normalize noradrenergic transmission (Lee, Javitch, & Snyder, Citation1983), indicating that a higher NAT density is associated with higher synaptic NA concentrations and therefore with a higher HPA response, as reflected by the close association between prefrontal and limbic NAT availability with HPA axis responsiveness observed in healthy, non-obesity controls. (2) The absence of a significant association between prefrontal-limbic NAT availability and HPA axis activity in individuals with obesity hint towards a loss (or gradual reduction) of the prefrontal top-down control to a distress-induced regulation mediated by the subcortical noradrenergic system. In keeping with this hypothesis, one may speculate that lowered NAT in obesity is accompanied by higher intra- or extra-synaptic NA concentrations as an indicator of a higher noradrenergic tone that increases HPA axis activity and changes AVP tone (as indexed by copeptin). This is in accordance with pharmacological studies showing that NAT inhibition by reboxetine leads to higher ACTH and cortisol responses in the dex/CRH test (Schule et al., Citation2006), or to higher copeptin serum concentrations after the consumption of amphetamines (Simmler et al., Citation2011). It has to be noted that only the short-term application of NAT inhibitors produce enhanced HPA activity, whereas long-term administration leads to a gradual normalization of HPA activation by the restoration of feedback control, partly explaining the effectiveness of NAT inhibitors in the treatment of stress-related disorders (Schule, Citation2007).
4.3. Limitation
This is an observational trial. From the pilot character of the study, we cannot conclude whether the individual stress response helps to predict phenotype; this has to be elucidated in larger, longitudinal samples that reduce the relatively high variance in HPA axis activity, which was driven by a few subjects with pronounced stress reactivity. Another limitation, which was yet beyond the score of this research project, is that the number of study participants appeared inappropriate to differentiate effects of age and sex on the outcome measures of the stress test results or NAT DVR (Bangasser et al., Citation2013; Künzel et al., Citation2003; Rothermel et al., Citation2016).
The time interval between PET scan and the dex/CRH test was within a median of 17.5 days. A possible limitation, however, is that two participants out of the non-obesity controls underwent NAT PET and neuroendocrine testing within >2 months due to logistic difficulties, maybe partly explaining the high variance. Moreover, a challenging aspect of any PET study on central NAT availability is that NAT levels are low and changes in modulatory systems to which the noradrenergic fibers belong are rather low and difficult to balance for accuracy and noise. Hence, the interpretability of data, in particular, of areas with low NAT expression, which includes the prefrontal cortex, is limited and the results need replication in studies with large sample sizes.
5. Conclusion
Stress has long been associated with obesity (Spencer & Tilbrook, Citation2011; van der Valk et al., Citation2018). Previous literature suggested individual alterations of the HPA and AVP stress response to predispose to an obesogenic phenotype or behavior in some individuals (Enhörning et al., Citation2011; Epel, Lapidus, McEwen, & Brownell, Citation2001; Rosmond & Bjorntorp, Citation2000; Schinke et al., Citation2017). Our work aimed to add new insights into how the HPA and AVP axes are related to the NA neurotransmitter system in the living human brain in obesity and potentially in other stress-associated diseases. Altogether, this study combined for the first time in vivo PET measures of brain noradrenergic transmission with an assessment of HPA axis activity and the AVP system. The findings suggest a distinct NA modulated stress response in human obesity, which is centered in the hypothalamus and which is different from NA-mediated stress adaptation in healthy, non-obese individuals. If this points to a noradrenergic dysregulation or an alteration of homeostatic control together with changes in eating behavior that lead to obesity remains to be elucidated in a larger population or in diseases with pronounced stress axis dysregulation. If confirmed, the findings of our study may support pharmacological or behavioral treatment strategies to normalize NA transmission in individuals susceptible to compensate with overeating.
Acknowledgments
The authors thank Mathias Fasshauer for referring additional patients and for helpful discussion throughout the course of the study, and Dr. A. Willenberg, Mr. R. Schmidt and Mrs. K. Tautz for their assistance with laboratory analyses.
Disclosure statement
No potential conflict of interest was reported by the authors.
Additional information
Funding
References
- Abraham, S.B. , Rubino, D. , Sinaii, N. , Ramsey, S. , & Nieman, L.K . (2013). Cortisol, obesity, and the metabolic syndrome: A cross-sectional study of obese subjects and review of the literature. Obesity (Silver Spring, Md.), 21, E105–E117. doi:10.1002/oby.20083
- Aguilera, G . (2011). Regulation of the hypothalamic-pituitary-adrenal axis by neuropeptides. Hormone Molecular Biology and Clinical Investigation, 7, 327–336. doi:10.1515/HMBCI.2011.123
- Aihara, M. , Ida, I. , Yuuki, N. , Oshima, A. , Kumano, H. , Takahashi, K. , … Mikuni, M . (2007). HPA axis dysfunction in unmedicated major depressive disorder and its normalization by pharmacotherapy correlates with alteration of neural activity in prefrontal cortex and limbic/paralimbic regions. Psychiatry Research, 155, 245–256. doi:10.1016/j.pscychresns.2006.11.002
- Arnsten, A.F.T . (2009). Stress signalling pathways that impair prefrontal cortex structure and function. Nature Reviews Neuroscience, 10, 410–422. doi:10.1038/nrn2648
- Astrup, A. , Madsbad, S. , Breum, L. , Jensen, T.J. , Kroustrup, J.P. , & Larsen, T.M . (2008). Effect of tesofensine on bodyweight loss, body composition, and quality of life in obese patients: A randomised, double-blind, placebo-controlled trial. The Lancet, 372, 1906–1913. doi:10.1016/S0140-6736(08)61525-1
- Bailey, M.A . (2017). 11β-Hydroxysteroid dehydrogenases and hypertension in the metabolic syndrome. Current Hypertension Reports, 19, 100. doi:10.1007/s11906-017-0797-z
- Bains, J.S. , Wamsteeker Cusulin, J.I. , & Inoue, W . (2015). Stress-related synaptic plasticity in the hypothalamus. Nature Reviews Neuroscience, 16, 377–388. doi:10.1038/nrn3881
- Bangasser, D.A. , Reyes, B.A.S. , Piel, D. , Garachh, V. , Zhang, X.-Y. , Plona, Z.M. , … Valentino, R.J . (2013). Increased vulnerability of the brain norepinephrine system of females to corticotropin-releasing factor overexpression. Molecular Psychiatry, 18, 166–173. doi:10.1038/mp.2012.24
- Bjorntorp, P. , & Rosmond, R . (2000). Obesity and cortisol. Nutrition (Burbank, Los Angeles County, Calif.), 16, 924–936. doi:10.1016/S0899-9007(00)00422-6
- Boundy, V.A. , & Cincotta, A.H . (2000). Hypothalamic adrenergic receptor changes in the metabolic syndrome of genetically obese (ob/ob) mice. American Journal of Physiology. Regulatory, Integrative and Comparative Physiology, 279, R505–R514. doi:10.1152/ajpregu.2000.279.2.R505
- Cooper, M.S. , & Stewart, P.M . (2009). 11Beta-hydroxysteroid dehydrogenase type 1 and its role in the hypothalamus-pituitary-adrenal axis, metabolic syndrome, and inflammation. The Journal of Clinical Endocrinology and Metabolism, 94, 4645–4654. doi:10.1210/jc.2009-1412
- Derogatis, L.R. , Lipman, R.S. , & Covi, L . (1973). SCL-90: An outpatient psychiatric rating scale–preliminary report. Psychopharmacology Bulletin, 9, 13–28.
- Enhörning, S. , Struck, J. , Wirfält, E. , Hedblad, B. , Morgenthaler, N.G. , & Melander, O . (2011). Plasma copeptin, a unifying factor behind the metabolic syndrome. The Journal of Clinical Endocrinology and Metabolism, 96, E1065. doi:10.1210/jc.2010-2981
- Epel, E. , Lapidus, R. , McEwen, B. , & Brownell, K . (2001). Stress may add bite to appetite in women: a laboratory study of stress-induced cortisol and eating behavior. Psychoneuroendocrinology, 26, 37–49. doi:10.1016/S0306-4530(00)00035-4
- Farr, O.M. , Li, C-sR. , & Mantzoros, C.S . (2016). Central nervous system regulation of eating: Insights from human brain imaging. Metabolism: Clinical and Experimental, 65, 699–713. doi:10.1016/j.metabol.2016.02.002
- Feldman, S. , & Weidenfeld, J . (2004). Involvement of endogeneous glutamate in the stimulatory effect of norepinephrine and serotonin on the hypothalamo-pituitary-adrenocortical axis. Neuroendocrinology, 79, 43–53. doi:10.1159/000076044
- Hautzinger, M . (1991). The Beck Depression Inventory in clinical practice. Nervenarzt, 62, 689–696.
- Hesse, S. , Becker, G.-A. , Rullmann, M. , Bresch, A. , Luthardt, J. , Hankir, M.K. , … Sabri, O . (2017). Central noradrenaline transporter availability in highly obese, non-depressed individuals. European Journal of Nuclear Medicine and Molecular Imaging, 44, 1056–1064. doi:10.1007/s00259-016-3590-3
- Heuser, I. , Yassouridis, A. , & Holsboer, F . (1994). The combined dexamethasone/CRH test: A refined laboratory test for psychiatric disorders. Journal of Psychiatric Research, 28, 341–356. doi:10.1016/0022-3956(94)90017-5
- Hilbert, A. , & Tuschen-Caffier, B . (2006). Eating disorder examination: Deutschsprachige Übersetzung. Münster: Verlag für Psychotherapie Retrieved from http://www.vfp-muenster.de/publikationen/online.html
- Incollingo, R.A.C. , Epel, E.S. , White, M.L. , Standen, E.C. , Seckl, J.R. , & Tomiyama, A.J . (2015). Hypothalamic-pituitary-adrenal axis dysregulation and cortisol activity in obesity: A systematic review. Psychoneuroendocrinology, 62, 301–318. doi:10.1016/j.psyneuen.2015.08.014
- Katan, M. , Fluri, F. , Morgenthaler, N.G. , Schuetz, P. , Zweifel, C. , Bingisser, R. , … Christ-Crain, M . (2009). Copeptin: A novel, independent prognostic marker in patients with ischemic stroke. Annals of Neurology, 66, 799–808. doi:10.1002/ana.21783
- Keck, M.E. , Wigger, A. , Welt, T. , Muller, M.B. , Gesing, A. , Reul, J.M.H.M. , … Neumann, I.D . (2002). Vasopressin mediates the response of the combined dexamethasone/CRH test in hyper-anxious rats: Implications for pathogenesis of affective disorders. Neuropsychopharmacology, 26, 94–105. doi:10.1016/S0893-133X(01)00351-7
- Künzel, H.E. , Binder, E.B. , Nickel, T. , Ising, M. , Fuchs, B. , Majer, M. , … Modell, S . (2003). Pharmacological and nonpharmacological factors influencing hypothalamic-pituitary-adrenocortical axis reactivity in acutely depressed psychiatric in-patients, measured by the Dex-CRH test. Neuropsychopharmacology, 28, 2169–2178. doi:10.1038/sj.npp.1300280
- Lee, C.M. , Javitch, J.A. , & Snyder, S.H . (1983). Recognition sites for norepinephrine uptake: Regulation by neurotransmitter. Science (New York, N.Y.), 220, 626–629. doi:10.1126/science.6301013
- Li, C-sR. , Potenza, M.N. , Lee, D.E. , Planeta, B. , Gallezot, J.-D. , Labaree, D. , … Neumeister, A . (2014). Decreased norepinephrine transporter availability in obesity: Positron emission tomography imaging with (S,S)-(11)CO-methylreboxetine. NeuroImage, 86, 306–310. doi:10.1016/j.neuroimage.2013.10.004
- Liu, J.P. , Clarke, I.J. , Funder, J.W. , & Engler, D . (1994). Studies of the secretion of corticotropin-releasing factor and arginine vasopressin into the hypophysial-portal circulation of the conscious sheep. II. The central noradrenergic and neuropeptide Y pathways cause immediate and prolonged hypothalamic-pituitary-adrenal activation. Potential involvement in the pseudo-Cushing's syndrome of endogenous depression and anorexia nervosa. Journal of Clinical Investigation , 93, 1439–1450. doi:10.1172/JCI117121
- Mandela, P. , & Ordway, G.A . (2006). The norepinephrine transporter and its regulation. Journal of Neurochemistry, 97, 310–333. Retrieved from http://onlinelibrary.wiley.com/doi/10.1111/j.1471-4159.2006.03717.x/full
- Melasch, J. , Rullmann, M. , Hilbert, A. , Luthardt, J. , Becker, G.A. , Patt, M. , … Pleger, B . (2016). The central nervous norepinephrine network links a diminished sense of emotional well-being to an increased body weight. International Journal of Obesity ( Obesity), 40, 779–787. doi:10.1038/ijo.2015.216
- Moriguchi, S. , Yamada, M. , Takano, H. , Nagashima, T. , Takahata, K. , Yokokawa, K. , … Suhara, T . (2017). Norepinephrine transporter in major depressive disorder: A PET study. American Journal of Psychiatry, 174, 36–41. doi:10.1176/appi.ajp.2016.15101334
- Myers, B. , Scheimann, J.R. , Franco-Villanueva, A. , & Herman, J.P . (2017). Ascending mechanisms of stress integration: Implications for brainstem regulation of neuroendocrine and behavioral stress responses. Neuroscience and Biobehavioral Reviews, 74, 366–375. doi:10.1016/j.neubiorev.2016.05.011
- Nelson, D.L. , & Gehlert, D.R. Gehlert (2006). Central nervous system biogenic amine targets for control of appetite and energy expenditure. Endocrine, 29, 49–60. doi:10.1385/ENDO:29:1:49
- Paeger, L. , Karakasilioti, I. , Altmüller, J. , Frommolt, P. , Brüning, J. , & Kloppenburg, P . (2017). Antagonistic modulation of NPY/AgRP and POMC neurons in the arcuate nucleus by noradrenalin. eLife, 6, pii: e25770. doi:10.7554/eLife.25770
- Pasquali, R. , Vicennati, V. , Cacciari, M. , & Pagotto, U . (2006). The hypothalamic-pituitary-adrenal axis activity in obesity and the metabolic syndrome. Annals of the New York Academy of Sciences, 1083, 111–128. doi:10.1196/annals.1367.009
- Plotsky, P.M. , Cunningham, E.T., JR. , & Widmaier, E.P . (1989). Catecholaminergic modulation of corticotropin-releasing factor and adrenocorticotropin secretion. Endocrine Reviews, 10, 437–458. doi:10.1210/edrv-10-4-437
- Radant, A. , Peskind, E.R. , Wilkinson, C.W. , Veith, R.C. , Dorsa, D.M. , Leake, R.D. , … Raskind, M.A . (1992). Neurohypophyseal and pituitary-adrenocortical responses to the alpha 1 agonist methoxamine in humans. Neuroendocrinology, 55, 361–366. doi:10.1159/000126145
- Robertson, S.D. , Matthies, H.J.G. , Owens, W.A. , Sathananthan, V. , Christianson, N.S.B. , Kennedy, J.P. , … Galli, A . (2010). Insulin reveals Akt signaling as a novel regulator of norepinephrine transporter trafficking and norepinephrine homeostasis. The Journal of Neuroscience: The Official Journal of the Society for Neuroscience, 30, 11305–11316. doi:10.1523/JNEUROSCI.0126-10.2010
- Rosmond, R. , & Bjorntorp, P . (2000). The hypothalamic-pituitary-adrenal axis activity as a predictor of cardiovascular disease, type 2 diabetes and stroke. Journal of Internal Medicine, 247, 188–197. doi:10.1046/j.1365-2796.2000.00603.x
- Rothermel, J. , Kulle, A. , Holterhus, P.-M. , Toschke, C. , Lass, N. , & Reinehr, T . (2016). Copeptin in obese children and adolescents: Relationships to body mass index, cortisol and gender. Clinical Endocrinology, 85, 868–873. doi:10.1111/cen.13235
- Saleem, U. , Khaleghi, M. , Morgenthaler, N.G. , Bergmann, A. , Struck, J. , Mosley, T.H. , & Kullo, I.J . (2009). Plasma carboxy-terminal provasopressin (copeptin): A novel marker of insulin resistance and metabolic syndrome. The Journal of Clinical Endocrinology and Metabolism, 94, 2558–2564. doi:10.1210/jc.2008-2278
- Schinke, C. , Hesse, S. , Stoppe, M. , Meyer, K. , Schmidt, E. , Orthgiess, J. , … Then Bergh, F . (2017). Post-dexamethasone serum copeptin corresponds to HPA axis responsiveness in human obesity. Psychoneuroendocrinology, 78, 39–47. doi:10.1016/j.psyneuen.2017.01.004
- Schule, C . (2007). Neuroendocrinological mechanisms of actions of antidepressant drugs. Journal of Neuroendocrinology, 19, 213–226. doi:10.1111/j.1365-2826.2006.01516.x
- Schule, C. , Baghai, T.C. , Eser, D. , Zwanzger, P. , Jordan, M. , Buechs, R. , & Rupprecht, R . (2006). Time course of hypothalamic-pituitary-adrenocortical axis activity during treatment with reboxetine and mirtazapine in depressed patients. Psychopharmacology, 186, 601–611. doi:10.1007/s00213-006-0382-7
- Simmler, L.D. , Hysek, C.M. , & Liechti, M.E . (2011). Sex differences in the effects of MDMA (ecstasy) on plasma copeptin in healthy subjects. The Journal of Clinical Endocrinology and Metabolism, 96, 2844–2850. doi:10.1210/jc.2011-1143
- Sivukhina, E.V. , & Jirikowski, G.F . (2016). Magnocellular hypothalamic system and its interaction with the hypothalamo-pituitary-adrenal axis. Steroids, 111, 21–28. doi:10.1016/j.steroids.2016.01.008
- Spanakis, E.K. , Wand, G.S. , Ji, N. , & Golden, S.H . (2016). Association of HPA axis hormones with copeptin after psychological stress differs by sex. Psychoneuroendocrinology, 63, 254–261. doi:10.1016/j.psyneuen.2015.10.009
- Spencer, S.J. , & Tilbrook, A . (2011). The glucocorticoid contribution to obesity. Stress (Amsterdam, Netherlands), 14, 233–246. doi:10.3109/10253890.2010.534831
- Then Bergh, F. , Kumpfel, T. , Trenkwalder, C. , Rupprecht, R. , & Holsboer, F . (1999). Dysregulation of the hypothalamo-pituitary-adrenal axis is related to the clinical course of MS. Neurology, 53, 772–777. doi:10.1212/WNL.53.4.772
- Torres, G.E. , Gainetdinov, R.R. , & Caron, M.G . (2003). Plasma membrane monoamine transporters: structure, regulation and function. Nature Reviews Neuroscience, 4, 13–25. doi:10.1038/nrn1008
- Ulrich-Lai, Y.M. , & Herman, J.P . (2009). Neural regulation of endocrine and autonomic stress responses. Nature Reviews Neuroscience, 10, 397–409. doi:10.1038/nrn2647
- van der Valk, E.S. , Savas, M. , & van Rossum, E.F.C . (2018). Stress and obesity: Are there more susceptible individuals? Current Obesity Reports, 7, 193–203. doi:10.1007/s13679-018-0306-y
- Zhou, J . (2004). Norepinephrine transporter inhibitors and their therapeutic potential. Drugs of the Future, 29, 1235–1244. doi:10.1358/dof.2004.029.12.855246