Abstract
Cardiovascular disease (CVD) remains the leading cause of disease burden globally and chronic stress is associated with increased risk of CVD. Recognition of chronic occupational stressors as a potential contributor to CVD highlights the need to recognize and prevent stress during work. The ubiquity of wearable technology devices to monitor health provides a new opportunity to noninvasively examine the cardiovascular system throughout a work shift. In the current study, we examined changes in heart rate (HR) during a work shift in a retail store setting using 23 healthy female and male subjects that differed in their physical fitness status. Subjects had their HR tracked via an Apple Watch during three typical work shifts. The results demonstrated an increase in HR during a work shift to a level observed during a moderate stressor (resting HR = 83.2 BPM ± 7.8; highest HR mean = 109.1 BPM ± 11.7; p < .0001). Female subjects demonstrated a significantly elevated maximum HR, a larger change in HR, and a larger percent change in HR compared with males (all p < .05). Physical activity status did not influence the observed changes in HR for females or males. Neither the time of day the work shift occurred nor the length of the shift modulated the observed pattern of HR changes. Collectively, our findings demonstrate the potential for wearables in biomedical research and personalized health.
Introduction
Homeostatic control of the cardiovascular system is critical for organisms in order to maintain optimal health (Ramsay & Woods, Citation2014). During times of stress, the cardiovascular system must respond appropriately in order to properly meet the demands of the stressor on the organism. For example, during an acute bout of physical activity, a number of physiologic adjustments must occur to provide the necessary supply of oxygen and nutrients to meet the demands of muscular work required by the activity (Rowell, Citation1993). A failure of the cardiovascular system to respond appropriately to this stressor can have detrimental impacts on the organism (e.g. the inability to escape a predator).
While turning on the cardiovascular system during a “fight-or-flight” situation via the autonomic nervous system (ANS) and hypothalamic–pituitary–adrenal axis (HPA) is an adaptive response in an organism (Dhabhar Citation2018; Fleshner and Crane Citation2017; McEwen Citation1998), chronic activation of the cardiovascular system can have deleterious consequences (Burford, Webster, & Cruz-Topete, Citation2017; DeMorrow, Citation2018; Sapolsky, Citation1999). For example, numerous studies indicate that exposure to chronic, daily stressors, and/or severe psychological trauma can also increase the risk of developing and dying from cardiovascular disease (CVD) (Cohen, Edmondson, & Kronish, Citation2015; Kivimäki & Steptoe, Citation2018). A recent meta-analyses of prospective observational studies found that social isolation and loneliness were associated with a 50% increased risk of incident CVD events, while the increased risk associated with work-related stress was similar at 40% (Steptoe & Kivimäki, Citation2013). Chronic stressors have also been linked to worse prognosis in patients with existing CVD (Carroll, Naylor, Marsden, & Dornan, Citation2003; Sabzmakan et al., Citation2014). Given that coronary heart disease and stroke remain the two leading causes of disease burden globally (GBD 2016 Causes of Death Collaborators, Citation2017), and chronic stress is associated with increased risk of CVD (Kivimäki & Steptoe, Citation2018), there is a need to identify sources of chronic stress as they might serve as complementary targets for disease prevention/therapeutic management.
Researchers have recently recognized that chronic occupational stressors such as adverse psychosocial working conditions, job strain, effort-reward imbalance, and long working hours are associated with a higher risk of CVD (Kivimäki et al., Citation2012), musculoskeletal disorders (Ariëns et al., Citation2001; Hoogendoorn, van Poppel, Bongers, Koes, & Bouter, Citation2000), in addition to depression and anxiety (Bernburg, Vitzthum, Groneberg, & Mache, Citation2016; Tanner, Bamberg, & Kozak, Citation2015). In addition to potential ill health effects, chronic occupation stress is also a cause for absenteeism from work resulting in financial implications (Houtman et al., Citation1999; Mauss, Li, Schmidt, Angerer, & Jarczok, Citation2015). Thus, it would be beneficial to be able to objectively monitor (and subsequently reduce) one’s physiological stress while working.
One increasingly popular tool to assess occupation stress is through monitoring changes in heart rate (HR) through wearable devices (Camm et al., Citation1996; Hernando, Roca, Sancho, Alesanco, & Bailón, Citation2018). In fact, altered HR variability has been reported in several diseases including cardiovascular diseases, such as ischemia, myocardial infarction and heart failure (Billman, Citation2011; Camm et al., Citation1996) and can indicate chronic stress (Hernando et al., Citation2016; Kim, Cheon, Bai, Lee, & Koo, Citation2018). Advances in the technology and accessibility of health related wearable devices has grown dramatically over the last decade (Canalys, Citation2018; Hernando et al., Citation2018) making it easier for nonpatient populations to monitor physiological measurements such as HR. Some of the most popular wearable devices are watch-like devices, which provide reliable HR estimates, derived from the pulse signal recorded at the wrist. The majority of these devices, such as the Apple Watch, are based on photophethysmography (PPG), which utilizes a light source that illuminates the skin and a photodetector that measures the intensity of the reflected light. HR estimates are based on the pulsatile changes in reflected light induced by fluctuations in blood flow every heartbeat (Hernando et al., Citation2018; Shcherbina et al., Citation2017).
Previous work has validated the use of HR assessment via the Apple Watch for stress monitoring (Bai, Hibbing, Mantis, & Welk, Citation2018; Hernando et al., Citation2018; Shcherbina et al., Citation2017) and demonstrated that wearable devices are effective in quantifying occupational stress in extreme environmental conditions (e.g. cold and heat stress situations) (Austad, Wiggen, Faerevik, & Seeberg, Citation2018). However, many of the studies involving examining changes in HR using wearable devices have been conducted in laboratory conditions with healthy subjects or in extreme conditions (Austad et al., Citation2018; Järvelin-Pasanen, Sinikallio, & Tarvanien, Citation2018). Less is known about the association between occupational stress and HR changes measured in real working life settings using wearable devices. In addition, a number of factors can influence HR responsiveness to stress, including age (Gerritsen et al., Citation2003; Koskinen et al., Citation2009), sex (Gerritsen et al., Citation2003, Koskinen et al., Citation2009), health and physical fitness status (Buchheit & Gindre, Citation2006; Dekker et al., Citation2000; Thayer, Åhs, Fredrikson, Sollers, & Wager, Citation2012), but few studies have examined these. Therefore, the aim of this study was to examine changes in HR during a work shift in an environmentally stable work environment (i.e. a retail store) using healthy female and male subjects that differed in their physical fitness status. The information obtained by this study may help in planning strategies for health assessment and stress reduction at work.
Materials and methods
Participants
Participants were employees of an Apple retail store (n = 23; 7 females and 16 males) aged 22-56 years who responded to in-store recruitment solicitations (). In order to assess physical activity status subjects were asked about their weekly physical activity levels. The American Heart Association suggests 150 minutes per week of moderate exercise or 75 minutes per week of vigorous exercise (or a combination of moderate and vigorous activity) for adults (AHA, Citation2016), thus subjects were categorized as either regularly meeting the physical activity guidelines (n = 9) or not regularly meeting the guidelines (n = 14) (). All subjects reported no chronic or acute illness, no regular medication regimen (with the exception of birth control), and good health prior to study onset. All procedures were approved by the University Institution Review Board (#1128772-3).
Table 1. Demographic characteristics of participants.
Procedures
All participants completed an informed consent and regularly wore an Apple Watch, Series 1. Participants were asked to abstain from caffeine and smoking 45 minutes prior to beginning work. Heart rate was recorded throughout a normal work shift (mean shift = 7.5 h) during three separate work days at an Apple Store. Subject job titles and responsibilities included sales, support and leadership. The QS Access app was used to extract HR data from each subject. This app converts the XML format type used by the Apple Health app into a CSV file that can be exported. For each work shift, the lowest HR, highest HR, change in HR, and percent change in HR was analyzed for each subject.
Data analysis
In order to examine differences in HR initial analysis utilized a t-test, analysis of variance (ANOVA) or two-way (Physical activity status by Sex) ANOVA to determine if main effects or interactions were present between groups of participants. Alpha was set at 0.05. When statistical significance was determined with ANOVA, Fisher’s PLSD post-hoc analysis was conducted to examine which groups differed. All figures are shown with group means and standard deviation bars.
Results
Participant characteristics
Of the 23 subjects who completed the study, the majority were males, not physically active, with a mean age of 30 years (). Two-way ANOVA revealed no differences in age based on sex or physical activity status (Female, Physically Active (PA)=24, Female, Not PA = 33.2, Male, PA = 27.5, Male, Not PA = 32.1; all p > .05).
Resting HR
Resting HR was determined to be the lowest recorded heart rate at the start of the subjects’ shift. Two-way ANOVA indicated no significant differences in resting HR between any of the groups ( and ; all p > .05).
Figure 1. Lowest average observed heart rate (HR) during a work shift in subjects. PA = physically active; Not PA = not physically active. Bars are means ± standard deviations of the means. N = 23.
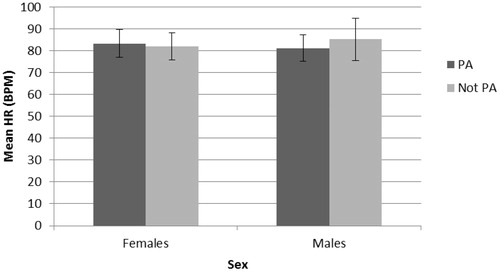
Table 2. Observed HR (BPM) throughout work shift.
HR changes during a work shift
During a work shift subjects demonstrated significant increases in HR ( and ). Unpaired t-test revealed that resting HR values (mean = 83.2 BPM ± 7.8) significantly increased (p < .0001) during the shift (highest HR mean = 109.1 BPM ± 11.7) in all subjects. Differences between the sexes were observed in both the highest HR observed () as well as the percent change in HR (). While both female (p < .0001) and male (p < .0001) subjects demonstrated a significant increase in HR (), two-way ANOVA indicated that female subjects demonstrated a significantly elevated highest HR compared with males (); F(1,64)=4.4, p = .03). Physical activity status, however, did not influence the highest HR observed (); p = .8). Overall, subjects demonstrated a mean increase in 25.9 BPM in HR at some point during their shift which resulted in a mean percent change of 39.5 (). Two-way ANOVA revealed that females had a larger change in HR during a work shift compared with males ( and , F(1,64)=3.8, p = .05); however, physical activity status did not modulate this change (p = .94). Similarly, ANOVA indicated that females had a larger percent change in HR during a work shift compared with males ( and , F(1,66)=4.6, p = .03) while physical activity had no effect (p = .96). In the current study, work shift duration and time of day varied between and within subjects and, therefore, we also examined if temporal factors influenced the previously mentioned changes in HR. No differences were observed in HR changes when a more typical work shift time (9:00–17:00, e.g.) was compared with an atypical shift time (12:00–21:00) in the same subject nor in different subjects (data not shown). The length of the work shift also did not appear to modulate the observed changes in HR.
Figure 2. Highest average observed heart rate (HR) during a work shift in subjects. Unpaired t-test revealed that resting HR values (mean = 83.2 BPM ± 7.8) significantly increased (p < .0001) throughout the shift (highest HR mean = 109.1 BPM ± 11.7) in all subjects. Both female (n = 7, p < .0001) and male (n = 16, p < .0001) subjects demonstrated a significant increase in HR. Two-way ANOVA indicated that female subjects demonstrated a significantly elevated highest HR compared with males (p = .03). Physical activity status did not influence the highest HR observed (p = .8). PA = physically active; Not PA = not physically active. Bars are means ± standard deviations of the means.
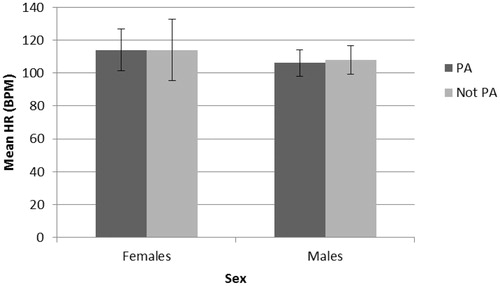
Figure 3. Average change (A) and percent change (B) in heart rate (HR) during a work shift in subjects. Two-way ANOVA revealed that females had a larger change in HR throughout a work shift compared with males (, p = .05), however, physical activity status did not modulate this change (p = .94). Females had a larger percent change in HR throughout a work shift compared with males (, p = .03) while physical activity had no effect (p = .96). PA = physically active; Not PA = not physically active. Bars are means ± standard deviations of the means.
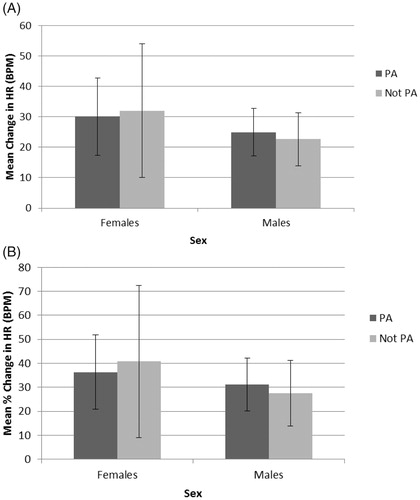
Discussion
Between 1990 and 2016, age-standardized life-years lived with ischemic heart disease declined by 47% and those of cerebrovascular disease by 17% (GBD 2016 Causes of Death Collaborators, 2016), in large part due to reductions in known cardiovascular disease factors such as smoking, blood pressure and serum cholesterol (Ezzati et al., Citation2015; Tzoulaki, Elliott, Kontis, & Ezzati, Citation2016). Despite these improvements, coronary artery disease and stroke remain the two leading causes of disease globally (GBD 2016 Causes of Death Collaborators, Citation2017) illustrating the need to identify new prevention targets. An emerging risk and prognostic factor for cardiovascular disease is chronic stress as mechanistic studies have corroborated previous observations demonstrating stress-related pathophysiological changes that underlie cardiovascular disease (Kivimäki & Steptoe, Citation2018; Steptoe & Kivimäki, 2012).
Researchers are increasingly recognizing occupational stress as one of the most commonly experienced stressors in adults (Kivimäki et al., Citation2012; Kivimäki & Steptoe, Citation2018). Fortunately, the popularity of wearable devices to monitor health has created a new noninvasive tool to assess occupational stress through monitoring changes in HR (Camm et al., Citation1996; Hernando et al., Citation2018). In the current study, we examined changes in HR measured via an Apple Watch during a work shift in an environmentally stable work environment using healthy female and male subjects that differed in their physical fitness status. Although previous work has validated the use of the HR assessment via the Apple Watch for stress monitoring (Bai et al., Citation2018; Hernando et al., Citation2018; Shcherbina et al., Citation2017) and for walking (Khushhal, Nicholas, Evans, Citation2017), this is the first study that we are aware of that examined occupational stress and HR changes measured in real working life settings using wearable devices.
Overall our results demonstrated that subjects experienced significant increases in HR during their work shift (, ). Heart rate values at rest (mean = 83.2 BPM ± 7.8) significantly increased (p < .0001) during the shift (highest HR mean = 109.1 BPM ± 11.7) at a level typically observed for this demographic during acute stress (Campisi, Bravo, Cole, & Gobeil, Citation2012; Hawley, Hargreaves, Joyner, & Zierath, Citation2014). Interestingly, although subjects’ lowest heart rates were not different among groups (, ), female subjects experienced the highest HR and the largest increase in HR (demonstrating a close to 40% change in HR) during t the work shift (, ). Numerous physiological differences exist between men and women including differences in height, weight, body composition (including fat and lean muscle mass), hormones (e.g. estrogen, progesterone, testosterone, etc.), and hemoglobin levels (Charkoudian, Citation2001; Charkoudian & Joyner, Citation2004). In addition, sex differences in response to physiological and psychological stressors have been reported in rodents and humans suggesting that females respond more robustly to an acute stressor than males (Heck & Handa, Citation2018; Oyola & Handa, Citation2017). The results from the current study are consistent with these findings but are the first that we are aware of that demonstrate a differential response to occupational stress (as indicated by changes in HR) in females during a normal work shift. It will be interesting to examine other indicators of stress (e.g. blood pressure, cortisol levels) in similar situations to further corroborate our findings.
Considerable data indicate that the physical fitness status of an organism modulates that organism’s response to a stressor (Campisi & Fleshner, Citation2003; Campisi et al., Citation2003; Fleshner et al., Citation2002; Fleshner, Greenwood, & Yirmiya, Citation2014; Lloyd et al., Citation2017). Therefore, we were surprised to observe in the current study that physical activity status did not influence the highest HR observed () nor the change in HR observed throughout the work shift (). One explanation for this finding was that we relied on subjects’ responses to a survey which might not have been reliable. Furthermore, it is possible that the use of AHA guidelines to classify subjects based on physical activity did not result in significant physiological differences in physical fitness between the subjects. A more direct, quantifiable measure of fitness status (e.g. VO2 max levels) or body composition (e.g. BMI) might have resulted in different results. In addition, we did not collect HR data during a resting (stabilization) time which might have provided for interesting comparisons. Finally, it is possible that a larger sample size might uncover a significant finding.
Because work shift duration and time of day varied in the current study we also examined if temporal factors influenced the previously mentioned changes in HR. No differences were observed in HR changes when a more typical work shift time (9:00–17:00 pm) was compared with an atypical shift time (12:00–21:00) (data not shown). The length of the work shift also did not appear to modulate the observed changes in HR. Given that circadian changes in hormone levels differ in females in males and that these hormones can influence HR (Charkoudian, Citation2001; Charkoudian & Joyner, Citation2004) and stress responses (Heck & Handa, Citation2018; Oyola & Handa, Citation2017) these were surprising results. We did not monitor food, beverage, or stimulant intake throughout the work shift or measure the type of interactions subjects had with customers or employees. It is possible that these external factors might have influenced hormonally modified changes in HR.
The results from the current study add to a growing literature demonstrating occupational stress as a potential modulator of the cardiovascular system and indicate that females might respond to occupational stress differently compared with males. Our analysis indicates that wearable metrics can potentially help in planning strategies for health assessment and stress reduction at work. As public adoption of wearable activity trackers continues to increase (Chuah et al., Citation2016), our findings demonstrate the potential for wearables in biomedical research and personalized health.
Acknowledgements
The authors wish to thank our subjects for participating in the study as well as the Apple Store in Boulder, CO for allowing us to conduct our study.
Disclosure statement
No potential conflict of interest was reported by the authors.
Additional information
Notes on contributors
Bethany Lucas
Bethany Lucas is an assistant professor.
Stella Grayson
Stella Grayson, Halimah Hamidu, Andrew Han, Sandra No, and Ajay Varghese are recent graduates of the Master of Science in Biomedical Sciences program at Regis University.
Halimah Hamidu
Stella Grayson, Halimah Hamidu, Andrew Han, Sandra No, and Ajay Varghese are recent graduates of the Master of Science in Biomedical Sciences program at Regis University.
Andrew Han
Stella Grayson, Halimah Hamidu, Andrew Han, Sandra No, and Ajay Varghese are recent graduates of the Master of Science in Biomedical Sciences program at Regis University.
Sandra No
Stella Grayson, Halimah Hamidu, Andrew Han, Sandra No, and Ajay Varghese are recent graduates of the Master of Science in Biomedical Sciences program at Regis University.
Ajay Varghese
Stella Grayson, Halimah Hamidu, Andrew Han, Sandra No, and Ajay Varghese are recent graduates of the Master of Science in Biomedical Sciences program at Regis University.
Jay Campisi
Jay Campisi is an associate professor at Regis University.
References
- American Heart Association Recommendations for Physical Activity in Adults. (2016, July 27). American Heart Association. Retrieved from http://www.heart.org/HEARTORG/HealthyLiving/PhysicalActivity/FitnessBasics/American-Heart-Association-Recommendations-for-Physical-Activity-in-Adults_UCM_307976_Article.jsp#.Wa-QVciGPIU
- Ariëns, G.A., Bongers, P.M., Hoogendoorn, W.E., Houtman, I.L., van der Wal, G., & van Mechelen, W. (2001). High quantitative job demands and low coworker support as risk factors for neck pain: results of a prospective cohort study. Spine, 26, 1896–1901.
- Austad, H., Wiggen, Ø., Færevik, H., & Seeberg, T.M. (2018). Towards a wearable sensor system for continuous occupational cold stress assessment. Industrial Health, 56, 228–240. doi:10.2486/indhealth.2017-0162
- Bai, Y., Hibbing, P., Mantis, C., & Welk, G. (2018). Comparative evaluation of heart rate-based monitors: Apple Watch vs Fitbit Charge HR. Journal of Sports Sciences, 36, 1734–1741. doi:10.1080/02640414.2017.1412235
- Bernburg, M., Vitzthum, K., Groneberg, D.A., & Mache, S. (2016). Physicians’ occupational stress, depressive symptoms and work ability in relation to their working environment: a cross-sectional study of differences among medical residents with various specialties working in German hospitals. BMJ Open, 6, e011369. doi:10.1136/bmjopen-2016-011369
- Billman, G. (2011). Heart rate variability – a historical perspective. Frontiers in Physiology, 2, 86. doi:10.3389/fphys.2011.00086
- Buchheit, M., & Gindre, C. (2006). Cardiac parasympathetic regulation: respective associationswith cardiorespiratory fitness and training load. American Journal of Physiology Heart Circulatory Physiology, 291, H451–H458. doi:10.1152/ajpheart.00008.2006
- Burford, N.G., Webster, N.A., & Cruz-Topete, D. (2017). Hypothalamic-pituitary-adrenal axis modulation of glucocorticoids in the cardiovascular system. International Journal of Molecular Sciences, 18, 2150. doi:10.3390/ijms18102150
- Camm, A.J., Malik, M., Bigger, J.T., Brethardt, G., Cerutti, S., Cohen, R.J., … Kleiger, R.E., et al. (1996). Heart rate variability: Standards of measurement, physiological interpretation, and clinical use. Circulation, 93, 1043–1065.
- Campisi, J., & Fleshner, M. (2003). Role of extracellular HSP72 in acute stress-induced potentiation of innate immunity in active rats. Journal of Applied Physiology (Bethesda, Md.: 1985), 94, 43–52. doi:10.1152/japplphysiol.00681.2002
- Campisi, J., Bravo, Y., Cole, J., & Gobeil, K. (2012). Acute psychosocial stress differentially influences salivary endocrine and immune measures in undergraduate students. Physiology and Behavior, 107, 317–321. doi:10.1016/j.physbeh.2012.09.003
- Campisi, J., Leem, T.H., & Fleshner, M. (2003). Stress-induced extracellular Hsp72 is a functionally significant danger signal to the immune system. Cell Stress Chaperones, 8, 272–286.
- Canalys. 18 Million Apple Watches Ship in 2017, up 54% on 2016. 2018. Available online: https://www.canalys.com/newsroom/18-million-apple-watches-ship-2017-54-2016
- Carroll, C., Naylor, E., Marsden, P., & Dornan, T. (2003). How do people with type 2 diabetes perceive and respond to cardiovascular risk? Diabetic Medicine: A Journal of the British Diabetic Association, 20, 355–360.
- Charkoudian, N. (2001). Influences of female reproductive hormones on sympathetic control of the circulation in humans. Clinical Autonomic Research, 11, 295–301. doi:10.1007/BF02332974
- Charkoudian, N., & Joyner, M.J. (2004). Physiologic considerations for exercise performance in women. Clinics in Chest Medicine, 25, 247–255. doi:10.1016/j.ccm.2004.01.001
- Chuah, S.H.W., Rauschnabel, P.A., Krey, N., Nguyen, B., Ramayah, T., & Lade, S. (2016). Wearable technologies: The role of usefulness and visibility in smartwatch adoption. Computers in Human Behavior, 65, 276–284. doi:10.1016/j.chb.2016.07.047
- Cohen, B.E., Edmondson, D., & Kronish, I.M. (2015). State of the art review: depression, stress, anxiety, and cardiovascular disease. American Journal of Hypertension, 28, 1295–1302. doi:10.1093/ajh/hpv047
- Dekker, J.M., Crow, R.S., Folsom, A.R., Hannan, P.J., Liao, D., Swenne, C.A., & Schouten, E.G. (2000). Low heart rate variability in a 2-minute rhythm strip predicts risk of coronary heart disease and mortality from several causes: the ARIC study. Atherosclerosis risk in communities. Circulation, 102, 1239–1244.
- DeMorrow, S. (2018). Role of the hypothalamic-pituitary-adrenal axis in health and disease. International Journal of Molecular Sciences, 19, 986. doi:10.3390/ijms19040986
- Dhabhar, F.S. (2018). The short-term stress response – Mother nature’s mechanism for enhancing protection and performance under conditions of threat, challenge, and opportunity. Frontiers in Neuroendocrinology, 49, 175–192. doi:10.1016/j.yfrne.2018.03.004
- Ezzati, M., Obermeyer, Z., Tzoulaki, I., Mayosi, B.M., Elliott, P., & Leon, D.A. (2015). Contributions of risk factors and medical care to cardiovascular mortality trends. Nature Reviews Cardiology, 12, 508–530. doi:10.1038/nrcardio.2015.82
- Fleshner, M., & Crane, C.R. (2017). Exosomes, DAMPs and miRNA: features of stress physiology and immune homeostasis. Trends in Immunology, 38, 768–776. doi:10.1016/j.it.2017.08.002
- Fleshner, M., Campisi, J., Deak, T., Greenwood, B.N., Kintzel, J.A., Leem, T.H., … Sorensen, B. (2002). Acute stressor exposure facilitates innate immunity more in physically active than in sedentary rats. American Journal of Physiology Regulatory, Integrative. and Comparative. Physiology, 282, R1680–1686.
- Fleshner, M., Greenwood, B.N., & Yirmiya, R. (2014). Neuronal-glial mechanisms of exercise-evoked stress robustness. Current Topics in Behavioral Neurosciences, 18, 1–12. doi:10.1007/7854_2014_277
- GBD 2016 Causes of Death Collaborators. (2017). Global, regional, and national age-sex specific mortality for 264 causes of death, 1980–2016: a systematic analysis for the Global Burden of Disease Study 2016. Lancet, 390, 1151–1210.
- Gerritsen, J., TenVoorde, B.J., Dekker, J.M., Kingma, R., Kostense, P.J., Bouter, L.M., & Heethaar, R.M. (2003). Measures of cardiovascular autonomic nervous function: agreement, reproducibility, and reference values in middle age and elderly subjects. Diabetologia, 46, 330–338. doi:10.1007/s00125-003-1032-9
- Hawley, J.A., Hargreaves, M., Joyner, M.J., & Zierath, J.R. (2014). Integrative biology of exercise. Cell, 159, 738–749. doi:10.1016/j.cell.2014.10.029
- Heck, A.L., & Handa, R.J. (2018). Sex differences in the hypothalamic-pituitary-adrenal axis' response to stress: an important role for gonadal hormones. Neuropsychopharmacology, 44, 45–58. doi: 10.1038/s41386-018-0167-9
- Hernando, A., Lázaro, J., Gil, E., Arza, A., Garzón, J., López-Antón, R., … Bailón, R. (2016). Inclusion of respiratory frequency information in heart rate variability analysis for stress assessment. IEEE Journal of Biomedical and Health Informatics, 20, 1016–1025. doi:10.1109/JBHI.2016.2553578
- Hernando, D., Roca, S., Sancho, J., Alesanco, Á., & Bailón, R. (2018). Validation of the apple watch for heart rate variability measurements during relax and mental stress in healthy subjects. Sensors, 18, 2619. doi:10.3390/s18082619
- Hoogendoorn, W.E., van Poppel, M.N., Bongers, P.M., Koes, B.W., & Bouter, L.M. (2000). Systematic review of psychosocial factors at work and private life as risk factors for back pain. Spine, 25, 2114–2125.
- Houtman, I., Kornitzer, M., De Smet, P., Koyuncu, R., De Backer, G., Pelfrene, E., … Östergren, P. (1999). Job stress, absenteeism and coronary heart disease European cooperative study (the JACE study): Design of a multicentre prospective study. European Journal of Public Health, 9, 52–57. doi:10.1093/eurpub/9.1.52
- Järvelin-Pasanen, S., Sinikallio, S., & Tarvainen, M.P. (2018). Heart rate variability and occupational stress-systematic review. Industrial Health, 56, 500–511. doi:10.2486/indhealth.2017-0190
- Khushhal, A., Nichols, S., Evans, W., et al. (2017). Validity and reliability of the Apple Watch for measuring heart rate during exercise. Sports Medicine International Open, 1, E206–E211. doi:10.1055/s-0043-120195
- Kim, H., Cheon, E., Bai, D., Lee, Y., & Koo, B. (2018). Stress and heart rate variability: A meta-analysis and review of the literature. Psychiatry Investigation, 15, 235–245. doi:10.30773/pi.2017.08.17
- Kivimäki, M., & Steptoe, A. (2018). Effects of stress on the development and progression of cardiovascular disease. Nature Reviews. Cardiology, 15, 215–229. doi:10.1038/nrcardio.2017.189
- Kivimäki, M., Leino-Arjas, P., Luukkonen, R., Riihimäki, H., Vahtera, J., & Kirjonen, J. (2002). Work stress and risk of cardiovascular mortality: prospective cohort study of industrial employees. Bmj (Clinical Research ed.), 325, 857. doi:10.1136/bmj.325.7369.857
- Kivimäki, M., Nyberg, S.T., Batty, G.D., Fransson, E.I., Heikkilä, K., Alfredsson, L., … Theorell, T, IPD-Work Consortium (2012). Job strain as a risk factor for coronary heart disease: A collaborative meta-analysis of individual participant data. Lancet (London, England), 380, 1491–1497. doi:10.1016/S0140-6736(12)60994-5
- Koskinen, T., Kähönen, M., Jula, A., Laitinen, T., Keltikangas-Järvinen, L., Viikari, J., … Raitakari, O.T. (2009). Short-term heart rate variability in healthy young adults: The cardiovascular risk in young Finns study. Autonomic Neuroscience: Basic and Clinical, 145, 81–88. doi:10.1016/j.autneu.2008.10.011
- Lloyd, B.A., Hake, H.S., Ishiwata, T., Farmer, C.E., Loetz, E.C., Fleshner, M., … Greenwood, B.N. (2017). Exercise increases mTOR signaling in brain regions involved in cognition and emotional behavior. Behavioural Brain Research, 323, 56–67. doi:10.1016/j.bbr.2017.01.033
- Mauss, D., Li, J., Schmidt, B., Angerer, P., & Jarczok, M.N. (2015). Measuring allostatic load in the workforce: A systematic review. Industrial Health, 53, 5.
- McEwen, B.S. (1998). Protective and damaging effects of stress mediators. New England Journal of Medicine, 338, 171–179. doi:10.1056/NEJM199801153380307
- McEwen, B.S. (1998). Stress, adaptation, and disease: Allostasis and allostatic loading. Annals of the New York Academy of Sciences, 840, 33–44. doi:10.1111/j.1749-6632.1998.tb09546.x
- Oyola, M.G., & Handa, R.J. (2017). Hypothalamic-pituitary-adrenal and hypothalamic-pituitary-gonadal axes: Sex differences in regulation of stress responsivity. Stress (Amsterdam, Netherlands), 20, 476–494. doi:10.1080/10253890.2017.1369523
- Ramsay, D.S., & Woods, S.C. (2014). Clarifying the roles of homeostasis and allostasis in physiological regulation. Psychological Review, 121, 225–247. doi:10.1037/a0035942
- Rowell, L.B. (1993). Human cardiovascular control. New York: Oxford University Press.
- Sabzmakan, L., Morowatisharifabad, M.A., Mohammadi, E., Mazloomy-Mahmoodabad, S.S., Rabiei, K., Naseri, M.H., … Mirzaei, M. (2014). Behavioral determinants of cardiovascular diseases risk factors: a qualitative directed content analysis. ARYA Atheroscler, 10, 71–81.
- Sapolsky, R.M. (1999). Glucocorticoids, stress, and their adverse neurological effects: Relevance to aging. Experimental Gerontology, 34, 721–732.
- Shcherbina, A., Mattsson, C., Waggott, D., Salisbury, H., Christle, J., Hastie, T., … Ashley, E. (2017). Accuracy in wrist-worn, sensor-based measurements of heart rate and energy expenditure in a diverse cohort. Journal of Personalized Medicine, 7, 3. doi:10.3390/jpm7020003
- Steptoe, A., & Kivimäki, M. (2013). Stress and cardiovascular disease: An update on current knowledge. Annual Review of Public Health, 34, 337–354. doi:10.1146/annurev-publhealth-031912-114452
- Tanner, G., Bamberg, E., Kozak, A., et al. (2015). Hospital physicians’ work stressors in different medical specialities: A statistical group comparison. Journal of Occupational Medicine and Toxicology, 10, 7. doi: 10.1186/s12995-015-0052-y
- Thayer, J.F., Åhs, F., Fredrikson, M., Sollers, J.J., 3rd., & Wager, T.D. (2012). A meta-analysis of heart rate variability and neuroimaging studies: Implications for heart rate variability as a marker of stress and health. Neuroscience and Biobehavioral Reviews, 36, 747–756. doi:10.1016/j.neubiorev.2011.11.009
- Tzoulaki, I., Elliott, P., Kontis, V., & Ezzati, M. (2016). Worldwide exposures to cardiovascular risk factors and associated health effects: Current knowledge and data gaps. Circulation, 133, 2314–2333. doi:10.1161/CIRCULATIONAHA.115.008718