Abstract
Psychological stress may be linked to cancer incidence; however, more direct evidence is required to support this viewpoint. In this study, we investigated the effects of stress on immunosurveillance against cancer cells using a previously established examination stress model. We showed that the cancer killing activity (CKA) of granulocytes (also known as polymorphic nuclear cells, PMNs) is sharply reduced during examination stress stimulation in some donors who are psychologically sensitive to examination stress, with the concentration of plasma stress hormones (cortisone, epinephrine, and norepinephrine) increasing accordingly. The effects of stress hormones on immune cell CKA were also investigated under two in vitro co-incubation conditions, with all three hormones found to exert inhibitory effects on the CKA of PMNs and mononuclear cells. We showed that stress triggered the release of stress hormones which had profound inhibitory effects on the innate anticancer functions of PMNs. These results provide a possible explanation for the relationship between psychological stress and cancer incidence.
1. Introduction
Among the general public there is a widespread belief that stress might contribute to the development of cancer (Willcox, Stewart, & Sitas, Citation2011). Many epidemiological and clinical studies have produced strong evidence to support this viewpoint, with a number of them reporting that stress not only increases the rate of tumor growth, but also the risk of tumor spread (Al-Wadei, Al-Wadei, Ullah, & Schuller, Citation2012; Al-Wadei, Plummer, & Schuller, Citation2009; Al-Wadei, Plummer, et al., Citation2012; Flint et al., Citation2013; Landen et al., Citation2007; Schuller, Al-Wadei, Ullah, & Plummer, Citation2012; Shahzad et al., Citation2010; Sklar & Anisman, Citation1980; Sood et al., Citation2006). Chida et al. reported that “stress-related psychosocial factors” were associated with higher cancer incidence among initially healthy individuals, lower survival rates in cancer patients, and higher mortality rates in communities with any type of cancer (Chida, Hamer, Wardle, & Steptoe, Citation2008).
Stress can be acute (short-lived) or chronic (repetitive or occurring over an extended period of time) (Chrousos, Citation2009). Acute stress is generally considered to have a positive effect on the organism (such as being confronted by predators), whereas chronic stress is usually detrimental and may lead to serious health complications (Dhabhar & McEwen, Citation1997; Krizanova, Babula, & Pacak, Citation2016). During chronic stress, the body remains in a constant state of “overdrive,” with dysregulation of the hypothalamic–pituitary–adrenal axis leading to an increase in the production of cortisol with simultaneous elevation in catecholamine levels (epinephrine and norepinephrine) (Krizanova et al., Citation2016). Clinical evidence indicates that such dysregulation or alterations in neuroendocrine hormones can be associated with a number of physical health problems (such as digestive problems, fertility problems, urinary problems, viral infections, and a weakened immune system) in healthy individuals and poorer outcomes in patients with cancer (Charmandari, Tsigos, & Chrousos, Citation2005; Heim, Ehlert, & Hellhammer, Citation2000; Kiecolt-Glaser, Bane, Glaser, & Malarkey, Citation2003; Seeman, Berkman, Blazer, & Rowe, Citation1994; Seeman, Singer, Rowe, Horwitz, & McEwen, Citation1997; Seeman & McEwen, Citation1996; Tyrka et al., Citation2008).
Numerous studies have shown that one of the major functions of stress hormones is to suppress the activities of the immune system (Dhabhar & McEwen, Citation1997; Elenkov, Citation2002; Glaser et al., Citation2001; Levy, Herberman, Lippman, & d'Angelo, Citation1987; Reiche, Nunes, & Morimoto, Citation2004; Sternberg, Chrousos, Wilder, & Gold, Citation1992). Recently, it has been demonstrated that chronic stress aggravates tumor progression by suppressing different facets of immune function, such as antigen presentation, humoral immunity, and cell-mediated immunity (Bovbjerg, Citation1991; Costanzo, Sood, & Lutgendorf, Citation2011; Dhabhar & McEwen, Citation1997; Elenkov, Citation2002; Glaser et al., Citation2001; Levy et al., Citation1987; Reiche et al., Citation2004; Saul et al., Citation2005).
Until recently, the specific role of granulocytes (also known as polymorphonuclear cells; PMNs) against cancer cells in vivo remained unclear. Our group revealed that PMNs exhibited the strongest anticancer activities in cancer-resistant mice and young healthy humans (Cui, Citation2010; Cui et al., Citation2003; Hicks et al., Citation2006). Animal studies have shown that PMNs with high cancer cell killing activity (CKA) are capable of reversing late-stage malignancies that would otherwise be lethal to the host (Cui et al., Citation2003; Hicks et al., Citation2006). Here, CKA was measured using an in vitro assay in which adherent cancer cells were co-cultured with non-adherent leukocytes (isolated PMNs and mononuclear cells; MNCs) for over 24 h. In most healthy humans, CKA varies from person to person and is predominantly present in the PMN fraction over the agranulocyte fraction (Cui, Citation2010).
Our study aimed to test the hypothesis that stress promotes cancer incidence by predominantly suppressing the CKA of PMNs via one or more stress hormones, namely cortisone, epinephrine, and norepinephrine. It is hypothesized that when CKA is suppressed, the major functionality of immunosurveillance against cancer cells is weakened, significantly increasing the opportunity for cancer cells to form and accumulate in the body. We used in vivo and in vitro models of stress responses to test this hypothesis. For the in vivo experiments, we used a previously reported human stress model in which a population of human subjects simultaneously experienced a stress event (Kiecolt-Glaser et al., Citation1986; McGregor, Murphy, Albano, & Ceballos, Citation2016). Blood samples were taken before, during, and after the stress event and the CKA values were subsequently measured. For the in vitro model, known amounts of different stress hormones were added to either whole blood or a CKA assay and the effects of the stress hormones on the CKA of PMNs were measured at different time points.
2. Materials and methods
2.1. Stress hormones
Hydrocortisone was purchased from TCI (Shanghai, China) and dissolved in ethanol (Sinopharm Chemical Reagent, Beijing, China). Epinephrine and norepinephrine were purchased from AMQUAR (Shanghai, China) and dissolved in HCl (0.5 M, Sinopharm Chemical Reagent). Each stress hormone mentioned above was diluted to the indicated concentrations in an appropriate medium on the day of use.
2.2. Donors
A total of 54 healthy undergraduate students (median age: 20 years; range: 18–21 years) from Tongji University (Shanghai, China) consented and were recruited as study participants from June 2016 to September 2017. During the study period, the participants did not consume alcohol, smoke, or use medicines. Their body mass indexes were under 30 kg/m2. No participants had any history of serious medical or psychiatric problems based on their medical records provided by physicians at Tongji University Hospital, nor did they have any history of immunosuppressive or radiation therapy (based on the same medical records). All recruited donors were interested in our research and donated their blood for free. This study was approved by the Tongji University Institutional Review Board.
2.3. Cells isolation
A total of 10 mL of blood was collected from each participant by heparinized venipuncture (Yu Li, Jiangsu, China). Granulocyte (or polymorphic nuclear cells, PMNs) and mononuclear cell (MNC) fractions were isolated from the whole blood via Percoll gradient separation (Blanks et al., Citation2011).
2.4. CKA assay
The A549 cell line (Shanghai Institute of Cell Biology, Shanghai, China) was used for the CKA assay. A549 cells (8 × 103 cells/well) were cultured for 24 h in 96-well plates with DMEM (Biological Industries, Kibbutz Beit Haemek, Israel) supplemented with 10% (v/v) FBS (Biological Industries) at 37 °C in the presence of 5% CO2. The CKA of PMNs and MNCs were tested separately, as described previously (Blanks et al., Citation2011; Cui et al., Citation2003). Briefly, isolated effector cells (PMNs or MNCs) were added to target cells at an E:T ratio of 20:1 and incubated at 37 °C for 24 h. After non-adherent cells had been carefully removed, the number of viable target cells not killed by the effector cells was determined using Cell Counting Kit-8 (CCK-8; ZOMANBIO, Beijing, China) according to the manufacturer’s instructions. Each data point was the average of triplicate experiments.
2.5. Assessment of cell morphology by light microscopy
For morphological studies, cytospin preparations of MNCs and PMNs were stained with Diff-Quik (Solarbio, Beijing, China) according to the manufacturer’s instructions (Ramirez et al., Citation2004) and photographed using a Zeiss Axioplan 2 fluorescence microscope (Nikon, Tokyo, Japan) with a 63× oil objective.
2.6. Plasma stress hormone measurement
To measure stress hormone levels, plasma samples were collected from the whole blood samples by centrifugation at 1000g and 4 °C for 20 min and stored at −80 °C. Plasma stress hormone concentrations were measured using ELISA kits, according to the manufacturer’s instructions (Crodis Biotech, Fujian, China). Briefly, standards or samples (50 µL) were added to the appropriate wells, then enzyme conjugate (100 µL) was added and incubated at 37 °C for 1 h. After washing the microtiter plate four times, substrates A and B (50 µL) were added to each well and incubated at 37 °C for 15 min. Finally, stop solution (50 µL) was added to each well and the optical density (OD) was measured using a microtiter plate reader at 450 nm. Each data point was the average of the triplicate experiments.
All three ELISA kits (human endogenous cortisol, epinephrine, and norepinephrine kits) were used in the same manner; however, the microtiter plate for each ELISA kit was pre-incubated with specific antibodies against endogenous cortisol, epinephrine, or norepinephrine by the manufacturer. In order to measure the concentration of stress hormones in the sample, the ELISA kits included a set of calibration standards which were assayed alongside the samples, allowing the operator to produce a standard curve of OD versus stress hormone concentration. The concentration of endogenous cortisol, epinephrine, or norepinephrine in the samples was determined by comparing the OD of the samples to the standard curve.
2.7. Cancer cell viability in the presence of stress hormones
To investigate the effect of stress hormones on A549 cell viability, hydrocortisone (0, 0.02, 0.2, 2, and 20 µg/mL), epinephrine (0, 0.01, 0.1, 1, and 10 ng/mL), and norepinephrine (0, 0.05, 0.5, 5, and 50 ng/mL) were separately incubated with A549 cells at 37 °C for 24 h. The number of viable cells was determined using a CCK-8 kit. The stress hormone detection concentrations ranged from 0.1 to 100× the physiological concentration (Abedelmalek, Chtourou, Souissi, & Tabka, Citation2015; Krakoff, Dziedzic, Mann, Felton, & Yeager, Citation1985). Four independent experiments were performed.
2.8. Statistical analysis
Statistical analyses were performed using Prism software, version 5.01 (GraphPad software, San Diego, United States). Two-tailed Student’s t-tests were used to analyze differences between two groups and one-way ANOVA with Dunnett’s post hoc tests were used to analyze differences between multiple groups. Statistical significance was set at p values of less than .05, .01, or .001, indicated by *, ** or ***, respectively.
3. Results
3.1. CKA of PMNs and MNCs
To confirm the purity of the isolated PMN and MNC (including monocytes, T cells, B cells, and NK cells) fractions, the different leukocyte fractions were isolated from the freshly collected peripheral blood of eight healthy donors, as described previously. An aliquot of each fraction was spun on to a glass slide, stained with Diff-Quik, and counted manually under a light microscope. All the PMN fractions from different individuals showed high degrees of purity, with >96% of cells displaying a morphology consistent with polynucleated cells. In all MNC fractions, >98% cells exhibited morphologies consistent with MNCs (). In all eight donors, the CKA of PMNs (74–94%) was significantly higher than that of MNCs (12–40%) at the same effect target ratio (E:T) of 20:1 (; n = 8; mean ± SEM; two-tailed Student’s t-test; ***, p < .001). These data confirmed the quality of the cell preparations required for the study and the predominance of CKA in the PMN fraction, as per our hypothesis.
3.2. Variation in PMN CKA in the examination stress model
This study, began with an accidental discovery. In 2016, we tested the CKA of PMNs from 23 samples of peripheral blood from each participant at several time points between June and July for another purpose. We observed a clear reduction in PMN CKA during the week of final exams (student donors took one–two exams per day for seven consecutive days). The five blood samples collected in the two weeks before examination showed high levels of PMN CKA (killing 78–92% of the target cells) and served as reference points. The PMN CKA measured one week before examination was significantly lower (40–50%), whereas extremely low levels of CKA were detected in the PMN fractions during the week of the final exams (0–15%). One week after examination, the PMN CKA had partially recovered (46–65%), whereas two weeks after examination the PMN CKA levels were almost entirely recovered (73–80%; Figure S1; n = 23; mean ± SEM; one-way ANOVA with Dunnett’s post hoc test; ***, p < .001). These data demonstrated that PMN CKA levels were lower in the weeks just before and after the final exams and were lowest during the week of the final exams, suggesting that final examinations may suppress student’s PMN CKA.
Using the stress model of final exams, we investigated the relationship between exam stimulation, PMN CKA, and plasma stress hormones (endogenous cortisol, epinephrine, and norepinephrine) concentrations the following year (10 student donors took one–two exams a day for seven consecutive days during exam week). The data revealed that most PMN CKA significantly decreased during the examination period and recovered in the following days, consistent with the phenomenon observed in 2016 (; n = 10; mean ± SEM; one-way ANOVA with Dunnett’s post hoc test; *, p < .05; **, p < .01). Contrary to the changes in PMN CKA, the majority of plasma stress hormone concentrations significantly increased during the examination period and decreased in the following days (; n = 10; mean ± SEM; one-way ANOVA with Dunnett’s post hoc test; *, p < .05; **, p < .01; ***, p < .001). Further analysis revealed that the PMN CKA of four of the 10 donors significantly decreased during the examination period and recovered in the following days (subjects 3–6; n = 4; mean ± SEM; one-way ANOVA with Dunnett’s post hoc test; Days 3 and 7, *, p < .05; Day 0, **, p < .01). Contrary to the changes in PMN CKA, the plasma stress hormone concentrations of these four donors significantly increased during the examination period and then decreased in the following days (n = 4; mean ± SEM; one-way ANOVA with Dunnett’s post hoc test; endogenous cortisol: Day 0, *, p < .05; Day 7, **, p < .01; epinephrine: Day 0, **, p < .01; Day 7, ***, p < .001; norepinephrine: Day 0, **, p < .01; Day 7, ***, p < .001). In addition, the PMN CKA of two donors decreased during the examination week but only one stress hormone (endogenous cortisol; subject 2) or no stress hormones (subject 1) increased during the examination week. Moreover, the PMN CKA and plasma stress hormone concentrations of four donors showed no significant changes under exam stimulation (subjects 7–10; n = 4; mean ± SEM; one-way ANOVA with Dunnett’s post hoc test), suggesting that (1) not all donors (only subjects 1–6) were sensitive (PMN CKA decreased) to examination stress; (2) in some sensitive donors (subjects 3–6) plasma stress hormone concentrations significantly increased and PMN CKA correspondingly decreased during the examination period; (3) in some sensitive donors (subject 2), the levels of certain stress hormones (endogenous cortisol) increased during the examination period; (4) in some sensitive donors (subject 1), the decrease in PMN CKA might be associated with hormones besides the three stress hormones mentioned above; and (5) in the donors who were not sensitive to examination stress (subjects 7–10), the plasma stress hormone concentrations and PMN CKA did not show any significant changes under exam stimulation. In this study, the plasma stress hormone secretion of sensitive donors (subjects 2–6) may be associated with PMN CKA inhibition under examination stress conditions.
Figure 2. (A) Variation in the PMN CKA of 10 students (subjects 1–10) under final examination stress stimulation. (B–D) Changes in the levels of three stress hormones in undergraduate students around exam week. Blood was collected from the subjects at 8:00 AM around exam week and the concentration of endogenous cortisol, epinephrine, and norepinephrine in the plasma was measured by ELISA (examination week was from Day 3 to Day 5 and is marked in gray; n = 10; mean ± SEM; one-way ANOVA with Dunnett’s post hoc test; *, p < .05; **, p < .01; ***, p < .001).
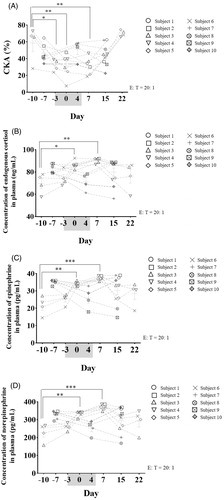
3.3. Cancer cell viability in the presence of stress hormones
We also investigated the cytotoxicity of stress hormones on PMN and A549 cells in order to exclude the effects of stress hormones on cell viability in the subsequent experiments. Three stress hormones (hydrocortisone, epinephrine, and norepinephrine) were incubated with human PMNs or A549 cells at 37 °C for 24 h and cell viability was tested using a CCK-8 assay. None of the stress hormones exhibited any significant effect on PMN (; n = 4; mean ± SD; one-way ANOVA with Dunnett’s post hoc test) or A549 viability at low concentrations; however, they strongly inhibited A549 cell viability at high concentrations (; n = 4; mean ± SD; one-way ANOVA with Dunnett’s post hoc test; *, p < .05; **, p < .01; ***, p < .001), consistent with previous studies (Croxtall & Flower, Citation1992). These results suggested that the effects of cytotoxicity on A549 cells should be excluded in the subsequent experiments.
Figure 3. Cytotoxicity of stress hormone co-culture with PMNs for 24 h. Cells were treated with hydrocortisone (A), epinephrine (B), norepinephrine (C), or a mixture of the three (D) for 24 h after which the relative viable cell number was measured using a CCK-8 assay (n = 4; mean ± SD; one-way ANOVA with Dunnett’s post hoc test).
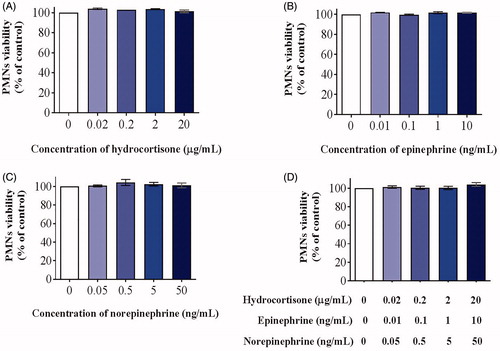
Figure 4. Cytotoxicity of stress hormone co-culture with A549 cells for 24 h. Cells were treated with hydrocortisone (A), epinephrine (B), norepinephrine (C), or a mixture of the three (D) for 24 h after which relative viable cell number was measured using a CCK-8 assay (n = 4; mean ± SD; one-way ANOVA with Dunnett’s post-hoc test; *, p < .05; **, p < .01; ***, p < .001).
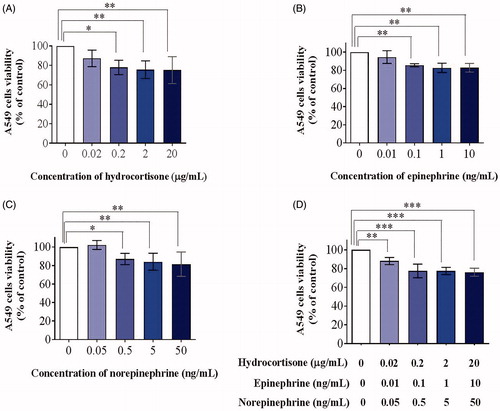
3.4. Stress hormones inhibit CKA under in vitro co-culture conditions
To determine whether the three major stress hormones could individually, or in combination, have a specific effect on PMN or MNC CKA, we used two different in vitro co-culture conditions. In the first, stress hormones were added to whole blood; upon co-culturing for 24 h, PMNs and MNCs were isolated from other blood components via Percoll gradient separation and the specific CKA was measured against A549 cells at an E:T ratio of 10:1 (). The data showed several findings: (1) hydrocortisone, epinephrine, and norepinephrine exhibited different inhibition effects on human PMN CKA when administrated individually, but with no statistical significance; (2) hydrocortisone, epinephrine, and norepinephrine significantly inhibited human PMN CKA when combined, indicating an additive effect on inhibition; (3) such an inhibitory effect occurred on the PMN CKA of all donors, but the degree of inhibition varied significantly between the donors; (4) among the three stress hormones tested, norepinephrine exhibited the strongest inhibition on human PMN CKA; (5) epinephrine exhibited the weakest inhibition on PMN CKA; and (6) the response of MNC CKA to the three stress hormones was similar with that of PMN (; n = 4; mean ± SEM; one-way ANOVA with Dunnett’s post hoc test; *, p < .05).
Figure 5. Effects of stress hormones on PMN and MNC CKA were investigated under stress hormones and whole blood co-culture conditions. Blood donated from healthy volunteers was co-incubated with 100× physiological concentrations of hydrocortisone (20 µg/mL), epinephrine (10 ng/mL), norepinephrine (50 ng/mL), or a mixture of the three at 37 °C for 24 h. PMNs (A) and MNCs (B) were isolated and incubated with A549 cells at 37 °C for a further 24 h, after which CKA was measured using a CCK-8 assay (n = 4; mean ± SEM; one-way ANOVA with Dunnett’s post hoc test; *, p < .05).
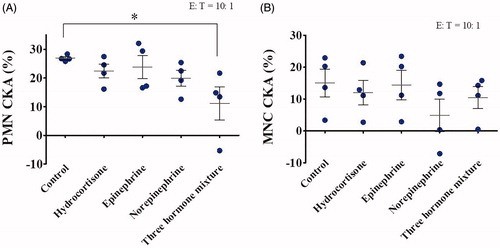
In the second in vitro co-culture conditions, PMNs and MNCs were isolated from the whole blood via Percoll gradient separation and stress hormones were added to the CKA reaction system at an E:T ratio of 10:1 and incubated for 24 h (). The findings include: (1) the inhibition effects of hydrocortisone, epinephrine, and norepinephrine on human PMN CKA varied considerably when administrated individually, but with no statistical significance; (2) the inhibition effects of hydrocortisone, epinephrine, and norepinephrine on human PMN CKA were found to be significant when administered in a combined manner, indicating an additive effect on inhibition; (3) an inhibitory effect occurred on the PMN CKA of all donors, but varied significantly between the donors; (4) among the three stress hormones tested, hydrocortisone exhibited the strongest inhibition effect on human PMN CKA; (5) epinephrine exhibited the weakest inhibition effect on human PMN CKS; and (6) the response of MNC CKA to the three stress hormones was similar to that of PMN (; n = 4; mean ± SEM; one-way ANOVA with Dunnett’s post hoc test; *, p < .05; **, p < .01). These results indicate that CKA inhibition could be directly induced by stress hormones.
Figure 6. Effects of stress hormones on PMN and MNC CKA were investigated under the in vitro CKA reaction system co-culture conditions. PMNs (A) and MNCs (B) were isolated from whole blood donated from healthy volunteers and co-incubated with 100× the physiological concentration of hydrocortisone (20 µg/mL), epinephrine (10 ng/mL), norepinephrine (50 ng/mL), or a mixture of the three in the CKA reaction system consisting of PMN and A549 cells at 37 °C for 24 h, after which CKA was measured using a CCK-8 assay (n = 4; mean ± SEM; one-way ANOVA with Dunnett’s post hoc test; *, p < .05; **, p < .01).
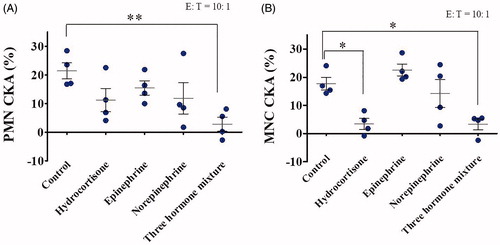
4. Discussion
Although stress is believed to contribute to cancer incidence, more direct evidence is required to support this viewpoint (Willcox et al., Citation2011). Some studies have suggested that stress is insignificant in cancer risk and survival (Nakaya, Citation2014); possible explanations for such divergent results are as follows: (1) the types of stress differ among different studies (Antonova, Aronson, & Mueller, Citation2011); (2) the research methodology remains controversial (Chida et al., Citation2008; Jenkins, Van Houten, & Bovbjerg, Citation2014); and (3) some outcomes might be restricted to certain cancer types or development stages (Chida et al., Citation2008).
If a connection between stress and cancer incidence exists, there might be two major mechanisms: (1) stress hormones cause normal cells to transform into cancer cells; and (2) stress hormones suppress a previously active system for eliminating cancer cells after they are formed. Numerous studies have reported the inhibitory effects of stress on the immune system, such as reducing NK cell number, inhibiting NK cell activity, decreasing T and B lymphocyte proliferation, and impairing antibody responses (Webster Marketon & Glaser, Citation2008). However, they do not provide sufficient explanation for the relationship between stress and cancer incidence for the following reasons: (1) the innate immune system can clear cancer cells following first-time recognition, thus playing a major role in preventing cancer; (2) T and B lymphocytes belong to the adaptive immune system and are important for preventing cancer development and migration, but not for decreasing incidence; and (3) although NK cells belong to the innate immune system, they are not the main immune cells due to their relatively small numbers.
This study was inspired by our previous finding that the PMNs of healthy humans and other mammals possess the profound innate activity of killing a large variety of cancer cells in vivo and in vitro. In this study, we found that PMN CKA levels vary significantly among different individuals and different time points in the same individual. Using a unique in vitro assay for innate CKA (Cui, Citation2010; Cui et al., Citation2003; Hicks et al., Citation2006), we set out to determine: (1) whether CKA was suppressed in a stress model in which human subjects simultaneously experienced a stress event; and (2) whether CKA could be directly inhibited by three major stress hormones individually or jointly in the in vitro assay.
The final exams of an undergraduate program served as a useful model for studying how human populations respond to the same stress event with precisely defined points (Kiecolt-Glaser et al., Citation1986; McGregor et al., Citation2016). Although stress responses are understandably different, most students experienced psychological pressures including anxiety, anticipation, and nervousness induced by the final exams, particularly in the week of the final exams. In this model, all participants carried on routine activities and the final exams were considered the only major stress factor during the study period. The advantages of this model include: (1) the start and end times of the stress event were clearly defined; (2) the intensity of the stress event was dominant among all other social activities and was well registered by the participants; and (3) the synchronicity of the subjects in the open social environment. In this study, the major innate CKA of the PMNs decreased in the stress-sensitive donors during the week of final exams compared to the levels before the exam week. CKA inhibition was observed in the randomly sampled subjects and also in the six subjects who underwent multiple blood collections before, during, and after the exam week. After the examination week, we observed rapid recovery of the suppressed CKA in those subjects, validating that the lower levels of CKA were genuine. CKA levels returned to pre-exam levels a short time after the stress event was over. It is important to note that we only tested ten subjects in the multiple blood collection experiments; therefore, the data obtained in this experiment only represent a relatively small proportion of the population and are not adequate to analyze the different subjects in detail. More subjects should be included in further studies to counteract the limitations of this study.
CKA protects the host by eliminating cancer cells and is sufficient to protect the host against lethal injections of cancer cells (Cui et al., Citation2003; Hicks et al., Citation2006). The long-term suppression or absence of CKA has been associated with a higher risk of developing cancer. Although the examination stress model for college students is a short-term model and no subject developed cancer or is expected to develop cancer, it is reasonable to assume that the cancer risk can significantly increase if CKA is absent or suppressed for a prolonged period (e.g. years). CKA is an innate activity of PMNs. One of the possible mechanisms by which PMNs are able to recognize a wide array of cancer cells is by detecting the unique negative surface charge displayed by most, if not all, cancer cells (Chen et al., Citation2016), since all normal cells possess a neutral surface charge (Chen et al., Citation2016).
We performed additional studies to determine whether CKA could be inhibited by exogenous stress hormones when co-cultured individually, or jointly, for 24 h with whole blood or a CKA reaction assay. Our results showed that all three hormones exerted an inhibitory effect on the CKA of PMNs and MNCs; however, subjects differed in their sensitivities to the effects of the stress hormones. These in vitro results were consistent with CKA variation in response to the stress induced by final exams.
It has been reported that the concentration of human plasma stress hormones could increase by 5- to 2600-fold due to stress (Boyanova, Citation2017; Dhabhar, Malarkey, Neri, & McEwen, Citation2012; Jentsch, Marz, & Kruger, Citation2013; Muthuraman et al., Citation2016). In order to simulate a more realistic in vivo environment under stress, we co-cultured whole blood or the CKA reaction system with stress hormones at 100-fold their normal physiological blood plasma levels.
It should be noted that two in vitro co-culture conditions were used in this study: one in which stress hormones and whole blood were co-cultured and one in which stress hormones and the CKA reaction system were co-cultured. By comparing the two conditions, we concluded that the former condition was much closer to the in vivo situation; therefore, the results of the former condition were deemed more accurate and realistic. Among all the three stress hormones investigated, norepinephrine may be the strongest PMN CKA inhibition agent in vivo.
Based on these results, we propose that the association between stress and cancer incidence may be mediated by PMN CKA inhibition as the result of the levels of one or more stress hormones being elevated for a long time. It has been reported that chemoattraction contributes to PMN CKA (Riedlinger et al., Citation2010). Other reports have shown that stress hormones inhibit PMN chemotaxis (Davis et al., Citation1991), which may be an important mechanism responsible for PMN CKA reduction during the examination week. Given the decreased lymphocyte count as a result of immune cell distribution in a similar stress model previously reported, it is possible that cancer risk can be significantly increased by lowering both blood lymphocyte count and the specific CKA (Dhabhar et al., Citation2012; Guidi et al., Citation1999).
Furthermore, the stress hormones showed no significant influence on the viability of A549 cells at low concentrations but significantly inhibited their viability at high concentrations. Some studies have reported that stress hormones contribute to the development and progression of non-small cell lung cancer (NSCLC), which seems contrary to our results (Al-Wadei, Plummer, et al., Citation2012). One possibility is that some NSCLC cells (such as HCC827, HCC4006, and H3255) are EGFR mutant cells, whereas others (such as A549, H441, and Clau-6) are EGFR wild-type cells, since it has been reported that stress hormones can only induce a robust increase in IL-6 levels in EGFR mutant NSCLC cells, but not in EGFR wild-type NSCLC cells (Nilsson et al., Citation2017; Yao et al., Citation2010). IL-6 can activate the IL-6/gp130/JAK/STAT signal pathway and promote EGFR mutant NSCLC cell proliferation, thereby inhibiting cellular apoptosis (Cao et al., Citation2015).
In the clinic, stress and fear after initial diagnosis may also exert a profound impact on overall survival by accelerating disease progression. It cannot be ignored that the general effect of stress on the overall functionality of the immune system could lead to a wide array of clinical complications.
In summary, our study has demonstrated that stress adversely effects a major protective system supported by the functionality of PMNs against cancer cells. These findings may offer an additional explanation for a possible link between cancer incidence and stress.
20190712-supplementary_data-R41.docx
Download MS Word (43.4 KB)Disclosure statement
No potential conflict of interest was reported by the authors.
Additional information
Funding
References
- Abedelmalek, S., Chtourou, H., Souissi, N., & Tabka, Z. (2015). Caloric restriction effect on proinflammatory cytokines, growth hormone, and steroid hormone concentrations during exercise in judokas. Oxidative Medicine and Cellular Longevity, 2015, 1. doi:10.1155/2015/809492
- Al-Wadei, H.A., Al-Wadei, M.H., Ullah, M.F., & Schuller, H.M. (2012). Celecoxib and GABA cooperatively prevent the progression of pancreatic cancer in vitro and in xenograft models of stress-free and stress-exposed mice. PLoS One, 7, e43376. doi:10.1371/journal.pone.0043376
- Al-Wadei, H.A., Plummer, H.K., 3rd, Ullah, M.F., Unger, B., Brody, J.R., & Schuller, H.M. (2012). Social stress promotes and gamma-aminobutyric acid inhibits tumor growth in mouse models of non-small cell lung cancer. Cancer Prevention Research, 5, 189–196. doi:10.1158/1940-6207.CAPR-11-0177
- Al-Wadei, H.A., Plummer, H.K., 3rd., & Schuller, H.M. (2009). Nicotine stimulates pancreatic cancer xenografts by systemic increase in stress neurotransmitters and suppression of the inhibitory neurotransmitter gamma-aminobutyric acid. Carcinogenesis, 30, 506–511. doi:10.1093/carcin/bgp010
- Antonova, L., Aronson, K., & Mueller, C.R. (2011). Stress and breast cancer: From epidemiology to molecular biology. Breast Cancer Research, 13, 208. doi:10.1186/bcr2836
- Blanks, M.J., Stehle, J.R., Du, W., Adams, J.M., Willingham, M.C., Allen, G.O., … Cui, Z. (2011). Novel innate cancer killing activity in humans. Cancer Cell International, 11, 26. doi:10.1186/1475-2867-11-26
- Bovbjerg, D.H. (1991). Psychoneuroimmunology. Implications for oncology? Cancer, 67, 828–832. doi:10.1002/1097-0142(19910201)67:3+<828::AID-CNCR2820671413>3.0.CO;2-A
- Boyanova, L. (2017). Stress hormone epinephrine (adrenaline) and norepinephrine (noradrenaline) effects on the anaerobic bacteria. Anaerobe, 44, 13–19. doi:10.1016/j.anaerobe.2017.01.003
- Cao, W., Liu, Y., Zhang, R., Zhang, B., Wang, T., Zhu, X., … Huang, L. (2015). Homoharringtonine induces apoptosis and inhibits STAT3 via IL-6/JAK1/STAT3 signal pathway in Gefitinib-resistant. Scientific Reports, 5, 8477–8477. doi:10.1038/srep08477
- Charmandari, E., Tsigos, C., & Chrousos, G. (2005). Endocrinology of the stress response. Annual Review of Physiology, 67, 259–284. doi:10.1146/annurev.physiol.67.040403.120816
- Chen, B., Le, W., Wang, Y., Li, Z., Wang, D., Lin, L., … Cui, Z. (2016). Targeting negative surface charges of cancer cells by multifunctional nanoprobes. Theranostics, 6, 1887–1898. doi:10.7150/thno.16358
- Chida, Y., Hamer, M., Wardle, J., & Steptoe, A. (2008). Do stress-related psychosocial factors contribute to cancer incidence and survival? Nature Clinical Practice Oncology, 5, 466–475. doi:10.1038/ncponc1134
- Chrousos, G.P. (2009). Stress and disorders of the stress system. Nature Reviews Endocrinology, 5, 374–381. doi:10.1038/nrendo.2009.106
- Costanzo, E.S., Sood, A.K., & Lutgendorf, S.K. (2011). Biobehavioral influences on cancer progression. Immunology and Allergy Clinics of North America, 31, 109–132. doi:10.1016/j.iac.2010.09.001
- Croxtall, J.D., & Flower, R.J. (1992). Lipocortin 1 mediates dexamethasone-induced growth arrest of the A549 lung adenocarcinoma cell line. Proceedings of the National Academy of Sciences of the United States of America, 89, 3571–3575. doi:10.1073/pnas.89.8.3571
- Cui, Z. (2010). Near term prospects for broad spectrum amelioration of cancer. In G.M. Fahy, M.D. West, L.S. Coles, & S. B. Harris (Eds.), The future of aging: Pathways to human life extension (pp. 307–329). Dordrecht: Springer Netherlands.
- Cui, Z., Willingham, M.C., Hicks, A.M., Alexander-Miller, M.A., Howard, T.D., Hawkins, G.A., … DeLong, C.J. (2003). Spontaneous regression of advanced cancer: Identification of a unique genetically determined, age-dependent trait in mice. Proceedings of the National Academy of Sciences of the United States of America, 100, 6682–6687. doi:10.1073/pnas.1031601100
- Davis, J.M., Albert, J.D., Tracy, K.J., Calvano, S.E., Lowry, S.F., Shires, G.T., & Yurt, R.W. (1991). Increased neutrophil mobilization and decreased chemotaxis during cortisol and epinephrine infusions. Journal of Trauma, 31, 725–731. discussion 731–722. doi:10.1097/00005373-199106000-00001
- Dhabhar, F.S., Malarkey, W.B., Neri, E., & McEwen, B.S. (2012). Stress-induced redistribution of immune cells – From barracks to boulevards to battlefields: a tale of three hormones – Curt Richter Award winner. Psychoneuroendocrinology, 37, 1345–1368. doi:10.1016/j.psyneuen.2012.05.008
- Dhabhar, F.S., & McEwen, B.S. (1997). Acute stress enhances while chronic stress suppresses cell-mediated immunity in vivo: A potential role for leukocyte trafficking. Brain, Behavior, and Immunity, 11, 286–306. doi:10.1006/brbi.1997.0508
- Seeman, T.E., Berkman, L.F., Blazer, D., & Rowe, J.D. (1994). Social ties and support and neuroendocrine function: The MacArthur studies of successful aging. Annals of Behavioral Medicine, 16, 95–106.
- Elenkov, I.J. (2002). Systemic stress-induced Th2 shift and its clinical implications. International Review of Neurobiology, 52, 163–186. doi:10.1016/S0074-7742(02)52009-2
- Flint, M.S., Baum, A., Episcopo, B., Knickelbein, K.Z., Liegey Dougall, A.J., Chambers, W.H., & Jenkins, F.J. (2013). Chronic exposure to stress hormones promotes transformation and tumorigenicity of 3T3 mouse fibroblasts. Stress, 16, 114–121. doi:10.3109/10253890.2012.686075
- Glaser, R., MacCallum, R.C., Laskowski, B.F., Malarkey, W.B., Sheridan, J.F., & Kiecolt-Glaser, J.K. (2001). Evidence for a shift in the Th-1 to Th-2 cytokine response associated with chronic stress and aging. The Journals of Gerontology Series A: Biological Sciences and Medical Sciences, 56, M477–M482. doi:10.1093/gerona/56.8.M477
- Guidi, L., Tricerri, A., Vangeli, M., Frasca, D., Errani, A.R., Di Giovanni, A., … Bartoloni, C. (1999). Neuropeptide Y plasma levels and immunological changes during academic stress. Neuropsychobiology, 40, 188–195. doi:10.1159/000026618
- Heim, C., Ehlert, U., & Hellhammer, D.H. (2000). The potential role of hypocortisolism in the pathophysiology of stress-related bodily disorders. Psychoneuroendocrinology, 25, 1–35. doi:10.1016/S0306-4530(99)00035-9
- Hicks, A.M., Riedlinger, G., Willingham, M.C., Alexander-Miller, M.A., Von Kap-Herr, C., Pettenati, M.J., … Cui, Z. (2006). Transferable anticancer innate immunity in spontaneous regression/complete resistance mice. Proceedings of the National Academy of Sciences of the United States of America, 103, 7753–7758. doi:10.1073/pnas.0602382103
- Jenkins, F.J., Van Houten, B., & Bovbjerg, D.H. (2014). Effects on DNA damage and/or repair processes as biological mechanisms linking psychological stress to cancer risk. Journal of Applied Biobehavioral Research, 19, 3–23. doi:10.1111/jabr.12019
- Jentsch, H.F., Marz, D., & Kruger, M. (2013). The effects of stress hormones on growth of selected periodontitis related bacteria. Anaerobe, 24, 49–54. doi:10.1016/j.anaerobe.2013.09.001
- Kiecolt-Glaser, J.K., Bane, C., Glaser, R., & Malarkey, W.B. (2003). Love, marriage, and divorce: newlyweds' stress hormones foreshadow relationship changes. Journal of Consulting and Clinical Psychology, 71, 176–188. doi:10.1037//0022-006X.71.1.176
- Kiecolt-Glaser, J.K., Glaser, R., Strain, E.C., Stout, J.C., Tarr, K.L., Holliday, J.E., & Speicher, C.E. (1986). Modulation of cellular immunity in medical students. Journal of Behavioral Medicine, 9, 5–21. doi:10.1007/BF00844640
- Krakoff, L.R., Dziedzic, S., Mann, S.J., Felton, K., & Yeager, K. (1985). Plasma epinephrine concentration in healthy men: Correlation with systolic pressure and rate-pressure product. Journal of the American College of Cardiology, 5, 352–356. doi:10.1016/S0735-1097(85)80058-9
- Krizanova, O., Babula, P., & Pacak, K. (2016). Stress, catecholaminergic system and cancer. Stress, 19, 419–428. doi:10.1080/10253890.2016.1203415
- Landen, C.N., Lin, Y.G., Armaiz Pena, G.N., Das, P.D., Arevalo, J.M., Kamat, A.A., … Sood, A.K. (2007). Neuroendocrine modulation of signal transducer and activator of transcription-3 in ovarian cancer. Cancer Research, 67, 10389–10396. doi:10.1158/0008-5472.CAN-07-0858
- Levy, S., Herberman, R., Lippman, M., & d'Angelo, T. (1987). Correlation of stress factors with sustained depression of natural killer cell activity and predicted prognosis in patients with breast cancer. Journal of Clinical Oncology, 5, 348–353. doi:10.1200/JCO.1987.5.3.348
- McGregor, B.A., Murphy, K.M., Albano, D.L., & Ceballos, R.M. (2016). Stress, cortisol, and B lymphocytes: A novel approach to understanding academic stress and immune function. Stress, 19, 185–191. doi:10.3109/10253890.2015.1127913
- Muthuraman, P., Nagajyothi, P.C., Chandrasekaran, M., Enkhtaivan, G., Venkitasamy, B., Kim, D.H., … Shim, J. (2016). Differential sensitivity of Madin-Darby Canine Kidney (MDCK) cells to epinephrine. The Journal of Nutrition, Health and Aging, 20, 486–493. doi:10.1007/s12603-015-0604-y
- Nakaya, N. (2014). Effect of psychosocial factors on cancer risk and survival. Journal of Epidemiology, 24, 1–6. doi:10.2188/jea.je20130124
- Nilsson, M.B., Sun, H., Diao, L., Tong, P., Liu, D., Li, L., … Heymach, J.V. (2017). Stress hormones promote EGFR inhibitor resistance in NSCLC: Implications for combinations with β-blockers. Science Translational Medicine, 9, eaao4307. doi:10.1126/scitranslmed.aao4307
- Ramirez, M.J., Titos, E., Claria, J., Navasa, M., Fernandez, J., & Rodes, J. (2004). Increased apoptosis dependent on caspase-3 activity in polymorphonuclear leukocytes from patients with cirrhosis and ascites. Journal of Hepatology, 41, 44–48. doi:10.1016/j.jhep.2004.03.011
- Reiche, E.M., Nunes, S.O., & Morimoto, H.K. (2004). Stress, depression, the immune system, and cancer. The Lancet Oncology, 5, 617–625. doi:10.1016/S1470-2045(04)01597-9
- Riedlinger, G., Adams, J., Stehle, J.R., Blanks, M.J., Sanders, A.M., Hicks, A.M., … Cui, Z. (2010). The spectrum of resistance in SR/CR mice: The critical role of chemoattraction in the cancer/leukocyte interaction. BMC Cancer, 10, 179. doi:10.1186/1471-2407-10-179
- Saul, A.N., Oberyszyn, T.M., Daugherty, C., Kusewitt, D., Jones, S., Jewell, S., … Dhabhar, F.S. (2005). Chronic stress and susceptibility to skin cancer. Journal of the National Cancer Institute, 97, 1760–1767. doi:10.1093/jnci/dji401
- Schuller, H.M., Al-Wadei, H.A., Ullah, M.F., & Plummer, H.K., 3rd. (2012). Regulation of pancreatic cancer by neuropsychological stress responses: A novel target for intervention. Carcinogenesis, 33, 191–196. doi:10.1093/carcin/bgr251
- Seeman, T.E., & McEwen, B.S. (1996). Impact of social environment characteristics on neuroendocrine regulation. Psychosomatic Medicine, 58, 459–471. doi:10.1097/00006842-199609000-00008
- Seeman, T.E., Singer, B.H., Rowe, J.W., Horwitz, R.I., & McEwen, B.S. (1997). Price of adaptation–allostatic load and its health consequences. Archives of Internal Medicine, 157, 2259–2268. doi:10.1001/archinte.1997.00440400111013
- Shahzad, M.M.K., Arevalo, J.M., Armaiz-Pena, G.N., Lu, C., Stone, R.L., Moreno-Smith, M., … Sood, A.K. (2010). Stress effects on FosB- and interleukin-8 (IL8)-driven ovarian cancer growth and metastasis. Journal of Biological Chemistry, 285, 35462–35470. doi:10.1074/jbc.M110.109579
- Sklar, L.S., & Anisman, H. (1980). Social stress influences tumor growth. Psychosomatic Medicine, 42, 347–365. doi:10.1097/00006842-198005000-00005
- Sood, A.K., Bhatty, R., Kamat, A.A., Landen, C.N., Han, L., Thaker, P.H., … Cole, S.W. (2006). Stress hormone-mediated invasion of ovarian cancer cells. Clinical Cancer Research, 12, 369–375. doi:10.1158/1078-0432.CCR-05-1698
- Sternberg, E.M., Chrousos, G.P., Wilder, R.L., & Gold, P.W. (1992). The stress response and the regulation of inflammatory disease. Annals of Internal Medicine, 117, 854–866. doi:10.7326/0003-4819-117-10-854
- Tyrka, A.R., Wier, L., Price, L.H., Ross, N., Anderson, G.M., Wilkinson, C.W., & Carpenter, L.L. (2008). Childhood parental loss and adult hypothalamic–pituitary–adrenal function. Biological Psychiatry, 63, 1147–1154. doi:10.1016/j.biopsych.2008.01.011
- Webster Marketon, J.I., & Glaser, R. (2008). Stress hormones and immune function. Cellular Immunology, 252, 16–26. doi:10.1016/j.cellimm.2007.09.006
- Willcox, S.J., Stewart, B.W., & Sitas, F. (2011). What factors do cancer patients believe contribute to the development of their cancer? (New South Wales, Australia). Cancer Causes & Control, 22, 1503–1511. doi:10.1007/s10552-011-9824-6
- Yao, Z., Fenoglio, S., Gao, D.C., Camiolo, M., Stiles, B., Lindsted, T., … Sordella, R. (2010). TGF-beta IL-6 axis mediates selective and adaptive mechanisms of resistance to molecular targeted therapy in lung cancer. Proceedings of the National Academy of Sciences of the United States of America, 107, 15535–15540. doi:10.1073/pnas.1009472107