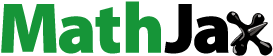
Abstract
In response to stress, apelin and corticotropin-releasing factor (CRF) are upregulated in the gastrointestinal (GI) tract. This study was designed to investigate the effect of stress on endogenous apelin in colon and its regulatory role on colonic motor functions. Colon transit (CT) was measured in rats exposed to acute restraint stress (ARS). APJ and CRF receptor antagonists F13A and astressin were administered intraperitoneally 30 min before ARS loading. Colonic muscle contractions were evaluated by in-vivo motility recording and in-vitro organ bath studies. Detection of apelin or CRF was performed using immunohistochemistry in proximal and distal colon of non-stressed (NS) and ARS-loaded rats. Immunoreactivity of CRF1 with apelin or APJ receptor was detected with double-labeled immunofluorescence in colonic myenteric neurons. Compared with NS rats, ARS accelerated the CT which was attenuated significantly by F13A or astressin. Following ARS, the expression of CRF was increased remarkably in distal colon, while the stress-induced change was not prominent in proximal colon. Apelin-positive cells were detected in myenteric ganglia of distal colon, while no apelin immunoreactivity observed in myenteric neurons of proximal colon. Both apelin and APJ receptor are colocalized with CRF1 in myenteric neurons of distal colon. In the in-vivo colonic motility experiments, apelin-13 exhibited a rapid stimulatory effect. CRF administration increased the motility which was abolished by F13A. Apelin-induced contractions in muscle strips were no longer observed with preadministration of F13A. These results suggest that enteric apelin contributes to the action of CRF on colonic motor functions under stressed conditions.
It has been suggested in rodents that acute stress increases the expression of apelin in gastrointestinal tissues. We have found that under stressed conditions, enteric apelin contributes to the CRF-induced alterations in colonic motor functions through APJ receptor.
LAY SUMMARY
1. Introduction
The alimentary tract is one of the primary targets of stressful stimuli. In rodents, it has been demonstrated that exposure to an acute psychological and/or physical stressor induces CRF upregulation in hypothalamic PVN which in turn accelerates CT; while inhibiting gastric emptying via autonomic neural pathways by mediation of CRF1 and CRF2, respectively (Masere et al., Citation2009; Ochi et al., Citation2008; Tache, Martinez, & Million, Citation2001; Tache & Bonaz, Citation2007). Besides the central nervous system, stress-induced upregulation of CRF has been shown in gut (Barreau et al., Citation2007; Estienne et al., Citation2010; Yuan, Wu, Wang, & Taché, Citation2010). In addition to its well-defined action in central stress circuitry, CRF is increased in gut and mediates the stress-induced changes in GI motor functions at the enteric level through its specific receptors, CRF1 and CRF2 (Barreau et al., Citation2007; Estienne et al., Citation2010; Tache, Larauche, & Yuan, Citation2018; Yuan et al., Citation2010). In rodents, it was demonstrated that acute restraint stress (ARS) induces GI motor dysfunction such as accelerated colonic transit (CT), increased fecal expulsion and delayed gastric emptying (Babygirija et al., Citation2010; Bulbul, Izgut-Uysal, & Sinen, Citation2016; Nakade et al., Citation2006; Zheng, Dobner, Babygirija, Ludwig, & Takahashi, Citation2009) which were antagonized by peripheral administration of CRF receptor antagonists (Rodino-Janeiro, Alonso-Cotoner, & Pigrau, Citation2015; Tache, Kiank, & Stengel, Citation2009; Tache et al., Citation2001).
In rodents, the stress-induced changes in GI motor functions were found to be abolished by peripheral pretreatment of CRF receptor antagonists suggesting that the released CRF from gut mediates these alterations through local mechanisms (Hussain, Kim, Huh, Lee, & Park, Citation2017; Martinez, Wang, & Rivier, Citation2004; Million, Zhao, & Luckey, Citation2013; Nozu, Kumei, Takakusaki, & Okumura, Citation2014; Nozu, Tsuchiya, Kumei, Takakusaki, & Okumura, Citation2013; Tache & Million, Citation2015). Within GI tract, the expression of both CRF1 and CRF2 have been detected in wide variety of cells including enteric neurons, goblet cells and stem cells of the colonic crypts, enterochromaffin cells and mucosal cells (Chatzaki et al., Citation2004; Kimura et al., Citation2007; Yuan, Wu, & Chang, Citation2007; Yuan et al., Citation2010). In colonic myenteric neurons, the CRF1 has been found predominantly (Chatzaki et al., Citation2004). Immunoreactivity for CRF1 has been investigated in colonic enteric neurons of guinea pigs, whereas CRF2 immunoreactivity was only detected in myenteric neurons (Liu et al., Citation2010; Tache et al., Citation2018). On the other hand, peripheral administration of CRF and CRF-related peptides urocortins have been shown to stimulate colonic motor functions in rats and mice (Hussain et al., Citation2017; Martinez, Wang, & Million, 2004; Nozu et al., Citation2013; Rodino-Janeiro et al., Citation2015; Tache & Bonaz, Citation2007; Tache & Million, Citation2015). The CRF-induced stimulation of colonic motor function was reversed by peripheral administration of nonselective CRF receptor antagonists (Hussain et al., Citation2017; Nozu et al., Citation2013, Citation2014; Rodino-Janeiro et al., Citation2015; Tache et al., Citation2001, Citation2009; Tache & Million, Citation2015). In contrast, peripheral administration of CRF2 agonists did not alter distal colonic motility both in rats and mice suggesting that peripheral CRF regulates colon motility through acting on the CRF1 (Hussain et al., Citation2017; Larauche et al., 2009; Martinez, Wang et al., Citation2004; Nozu et al., Citation2013; Yuan, Million, & Wu, Citation2007). Interestingly, Miampamba and colleagues showed that intraperitoneal administration of CRF increased the c-Fos expression in choline acetyltransferase-positive neurons which was reversed by pretreatment of CRF1 antagonist. These results suggest the local stimulatory action of CRF on proximal colon myenteric neurons through CRF1 (Miampamba, Maillot, Million, & Taché, Citation2002).
Apelin, a recently isolated peptide from bovine stomach extracts was characterized as the endogenous ligand for the G-protein-coupled APJ receptor (Tatemoto et al., Citation1998). The apelin/APJ system exhibits a widespread expression pattern in the GI tract including enteric neurons reside in myenteric and submucosal plexi, smooth muscle cells and gastric mucosal cells (Fournel, Drougard, & Duparc, Citation2015; Susaki et al., Citation2005; Wang et al., Citation2009). We have shown previously in rats that the stimulatory effect of peripheral exogenous apelin-13 on colonic motor functions was abolished by intraperitoneal (i.p.) preadministration of APJ receptor antagonist suggesting that the released apelin from alimentary canal appears to regulate colon motility through acting on APJ receptor in gut (Bülbül, Sinen, Birsen, & Izgüt-Uysal, Citation2017). On the other hand, in recent studies, exposure to acute water-immersion restraint stress (WIRS) for 360 min has been shown to induce gastric apelin expression in rats (Birsen, Gemici, Acar, Üstünel, & İzgüt-Uysal, Citation2017; Izgut-Uysal, Gemici, & Birsen, Citation2014). Importantly, we have recently shown in rats that apelin expression was upregulated in stomach and duodenum of stress-loaded rats, additionally, stress-induced changes in solid gastric emptying and intestinal transit were attenuated by APJ receptor antagonist F13A (Bülbül et al., Citation2019).
The accumulating evidence demonstrates the interaction between apelin and CRF in central stress neurocircuitry (Bulbul et al., Citation2016; Newson et al., Citation2009). Despite both neuropeptides are upregulated in gut upon stress and their interaction has been investigated in brain (Bulbul et al., Citation2016) and upper GI organs (Bülbül et al., Citation2019), it has not been investigated whether an interaction exists between CRF and apelin in colon under stressed conditions. Therefore, to determine the hypothesis that enteric apelin may play in mediation of stress, we evaluated the ability of APJ receptor antagonist F13A to reverse the acute stress-induced acceleration in CT. Additionally, we aimed to investigate whether (i) ARS alters colonic apelin expression and (ii) apelin and/or APJ receptors are colocalized with CRF1 in colonic myenteric neurons.
2. Material and methods
2.1. Animals
The adult male Wistar rats weighing 280–300 g were used in this study and approved by Animal Ethical Committee of Akdeniz University (B.30.2.AKD.0.05.07.00) and performed with standard guidelines for care and use of laboratory animals. All efforts were made to mitigate animal suffering and to reduce the number of animal in experiments. The rats were housed under temperature-controlled environment (22–24 °C) and illumination (12-h light cycle starting at 6:30 AM) with ad libitum access to food and water. Prior to the experiments, rats were acclimatized to handling for 7 days prior to the experiments.
2.2. Stress loading
Restraint stress has been used as a physical and psychogenic stressor in rodents (Dodiya, Forsyth, & Voigt, Citation2018; Farhin et al., Citation2019). ARS was applied for 90 min by placing the rats on a wooden plate with their trunks wrapped in a confining harness, while they were able to move their limbs and head but not their trunks, as previously reported (Bulbul, Babygirija, & Cerjak, Citation2012; Zheng et al., Citation2009).
2.3. Measurement of CT
CT was measured according to a spectrophotometric method described elsewhere (Bulbul et al., Citation2016). Under isoflurane (Baxter, Deerfield, IL, USA) anesthesia (5% for induction; 2.5% for maintenance in pure O2 at a flow rate of 200–400 ml/min), a 20 cm long silicon catheter with 2.3 mm outer diameter (Cole-Parmer, Vernon Hills, IL, USA) was placed into the proximal colon through an incision made 1 cm proximal to the cecocolic junction and fixed with sutures. Then, the catheter was tunneled through the anterior abdominal wall subcutaneously and placed outside the neck skin of rats. For postoperative analgesia, rats received tramadol hydrochloride (40 mg/kg, i.m.) and they were allowed to recover for 5 days. On the experiment day, 1.5 ml of saline containing 0.75 mg non-absorbable phenol red was injected via the colonic catheter, and the catheter was flushed with 0.5 ml of saline. Ninety minutes later, rats were euthanized under deep isoflurane anesthesia and the entire colon was immediately removed and divided into six segments of equal length. The released fecal content was collected and referred to as segment-7. Each colon segment was placed in 100 ml of 0.1 N NaOH and homogenized. The homogenates were allowed to stand for 1 h. Then, 5 ml of the supernatant was added to another tube containing 0.5 ml of 20% trichloroacetic acid. After centrifugation at 10,000 g for 30 min, 4 ml of 0.5 N NaOH was added to the supernatant. Phenol red was determined by measuring the absorption at 560 nm using a spectrophotometer (Shimadzu Corporation, Kyoto, Japan). CT was calculated as the geometric center of distribution of phenol red described as follows:
2.4. In-vivo colonic motility
Under isoflurane anesthesia, the proximal and distal parts of colon were exposed through a midline laparotomy. After two miniature 120 Ω strain gages (Kyowa Electronic Instruments, Tokyo, Japan) were implanted onto the serosal surface of the proximal and distal colon, abdominal muscles and skin were sutured and closed. The rats were intubated with an 18 G tracheal catheter and tail vein was catheterized with a 24 G catheter and PE50 tubing for the delivery of the drugs. Throughout the experiment, isoflurane anesthesia was maintained through a vaporizer (1.5% in 200 ml/min O2) connected to a mechanic rodent ventilator (Harvard Apparatus, Model 683, Holliston, MA, USA). The rats were then transferred to a heating pad and their rectal temperature was monitored and kept constant at 37 °C using a homeothermic controller (Harvard Apparatus, Model 50-079). The wires from the transducers were connected to a bridge amplifier. Following a 1 h of stabilization period, the proximal and distal colonic contractions were recorded using a computer-based data acquisition system and software (Axoscope, Molecular Devices, Sunnyvale, CA, USA). The area under the curve (AUC) was calculated and expressed as a motility index (MI) which was evaluated before and after the drug administrations.
2.5. In-vitro colonic motility
To investigate whether APJ receptors expressed in colon mediate colonic contractions in response to apelin-13, an in-vitro organ bath study was performed. The rats were fasted overnight and anesthetized by urethane (1.5 g·kg−1, i.p). Following adequate depth of anesthesia was obtained; circular muscle strips were isolated from the proximal and distal colon and mounted in the longitudinal axis in 20 ml organ baths filled with Krebs buffer solution. Mechanical activity was monitored through isometric force transducers (Commat, Model FDT05, Ankara, Turkey) connected to a digital data acquisition system (Biopac, Model MP150, Goleta, CA, USA). Throughout the protocol, the solution was gassed with the premixed 95%O2 and 5%CO2, while the temperature was maintained at 37.5 °C. Krebs solution was changed every 20 min. Following an equilibration period for 60 min, the effects of different doses of apelin-13 (10−9–10−6 M) on colonic motility were investigated with or without preadministration of APJ receptor antagonist F13A (10−6 M). At the end of the protocol, colonic motor responses to bethanechol (10−6 M) were monitored to test whether postganglionic cholinergic transmission is intact.
2.6. Drugs
CRF (Sigma Chemical, St Louis, MO, USA), nonselective CRF receptor antagonist astressin (Sigma), APJ receptor antagonist F13A (Phoenix Pharmaceuticals, Burlingame, CA, USA), apelin-13 (Tocris, Minneapolis, MN, USA) and bethanechol chloride (Sigma) were freshly prepared in sterile saline on the experimental days. In CT measurements, astressin (100 µg·kg−1) or F13A (300 µg·kg−1) was administered intraperitoneally 30 min prior to ARS induction. The doses of astressin and F13A were obtained from our previous report (Bülbül et al., Citation2019).
2.7. Colonic whole-mount longitudinal muscle-myenteric plexus (LMMP) preparation
In a separate group, following ARS loading rats were sacrificed by cervical dislocation under anesthesia. Segments of the distal colon (approximately 2–3 cm proximal from anus) and proximal colon (2–3 cm distal from ileocecal junction) were removed and placed in a dissecting dish containing phosphate buffered saline (PBS). After the colonic segments were flushed of their contents and trimmed of mesentery, they were cut longitudinally along the mesenteric border in cold PBS solution. The tissues were then pinned and stretched on a petri dish containing Sylgard with the mucosal side facing up. The tissues were incubated and fixed in 4% paraformaldehyde (PFA) for 18–24 h at 4 °C. Following incubation, the PFA was removed by rinsing the tissue with PBS. Then, under a dissecting stereo microscope, mucosa, submucosa and circular muscle layers were removed using fine forceps. The obtained LMMP was kept in PBS containing 0.1% sodium azide at 4 °C until the immunofluorescence staining.
2.8. Histology
After a transcardiac perfusion with 100 ml of a heparinized 0.9% NaCl followed by 100 ml of 4% PFA, the proximal and distal colon segments were removed and postfixed in PFA for 24 h at room temperature. Coronal colonic sections were then embedded to paraffin and 5 µm thick sections were cut using a rotary microtome (Leica RM 2125RT). Slides were dried overnight at 56 °C. The samples were deparaffinized in xylene at room temperature for 10 min, dehydrated with a graded ethanol series and then washed in distilled water. For immunoperoxidase staining, antigen retrieval was performed by boiling the slides in a citrate buffer (pH 6.0, 0.01 M) in a microwave oven at 100 °C for 7 min. After cooling for 20 min at room temperature, the sections were washed with PBS. Then, the slides were incubated with Ultra V blocking serum (TA-125-4B, Lab Vision) for 7 min to block nonspecific immunoglobulin binding. The slides were incubated with rabbit anti-CRF antibody (AB8901, Abcam) at 1:100 dilution overnight in a humidified chamber at 4 °C. Negative controls were performed by replacing the primary antibody with antibody diluent. After 3 × 5 min washing in PBS, the sections were incubated with peroxidase-conjugated anti-rabbit secondary antibody (Vector Lab. Inc., Burlingame, CA, USA) at 1:400 dilution for 45 minutes at room temperature. Then the slides were washed with PBS and peroxidase activity was visualized with 3,3′-Diaminobenzidine (DAB) (Sigma-Aldrich Co. LLC, Steinheim, Germany) for 1–2 min. The slides were counterstained with hematoxylin, dehydrated, mounted in entellan (Merck, NJ, USA) and examined by light microscopy.
For immunofluorescent staining, coronal colonic sections and LMMP tissues were washed in PBS, blocked with 10% normal horse serum with 0.5% Triton X-100 for 2 h at room temperature, and subsequently incubated in primary antibodies at room temperature for 72 hours. Primary antibodies were i) goat anti-CRF1 antibody (1:200; STJ71309, St. John’s Labs), ii) rabbit anti-apelin antibody (1:100; bs-2425R, Bioss), iii) rabbit anti-APJ antibody (1:200; AB66218; Abcam) and iv) mouse anti-PGP 9.5 antibody (1:200, AB8189, Abcam). Then, sections were incubated 18–24 h at room temperature with secondary antibodies. The secondary antibodies were donkey anti-mouse Alexafluor 568 or 350, donkey anti-rabbit Alexafluor 488 or 568 and donkey anti-goat Alexafluor 568 (ThermoScientific, Waltham MA; 1:500). After 3 × 15 min washing in PBS, the sections were mounted with Fluoromount-G (Southern Biotechnology, Birmingham, AL, USA) or mounting medium containing DAPI (Vectashield, H-1200, Burlingame, CA). The images were captured using a fluorescent microscope equipped with appropriate filters for secondary antibodies.
The colonic whole-mount LMMP preparations were harvested from distal colons of NS rats (n = 4). The intensity for apelin immunoreactivity was scored semi-quantitatively using the intensity categories: weak (+), moderate (++) and strong (+++). The total number of the neuronal cells in ganglia was counted and the immune-positive neurons were included in quantification. Apelin-positive cells were counted in 5 ganglia from each preparation and the number of the reactive cells was expressed as % of total neuronal cells.
2.9. Statistics
Statistical analyses were performed using SPSS v13.0 software. Data were presented as the mean ± SEM. The Kruskal–Wallis test followed by Mann–Whitney U test was used to determine any significance among the experimental groups. For in-vitro organ bath experiments, data were evaluated by the Friedman test followed by the Wilcoxon test. Results were expressed as means ± SE. Differences were considered to be statistically significant at p < .05.
3. Results
3.1. Effect of F13A and astressin on ARS-induced acceleration in CT
In vehicle-injected non-stressed (NS) animals, CT was measured 4.17 ± 0.19. ARS significantly accelerated CT (6.14 ± 0.27, F = 6.29, p < .01, n = 6), whereas, the ARS-induced acceleration was restored to the basal level by peripheral pretreatment of APJ receptor antagonist F13A (4.93 ± 0.60, F = 6.21, p < .05 vs ARS; p = .08 vs vehicle, n = 6) or nonselective CRF receptor antagonist astressin (4.30 ± 0.79, F = 8.29, p < .01 vs ARS; p = .11 vs vehicle, n = 6), ().
Figure 1. Effect of F13A and astressin on ARS-induced accelerated CT. Data are means ± SD. **p < .01 vs NS; #p < .05; ##p < .01 vs vehicle-ARS. The vehicle-treated NS group (white bar) rats were administered with saline and were euthanized 90 min after the phenol red application. Vehicle (500 µl of saline, i.p.), APJ receptor antagonist F13A (300 µg·kg−1, i.p.) or CRF receptor antagonist astressin (100 µg·kg−1, i.p.) was administered 30 min prior to the ARS loading. NS: non-stressed, ARS: acute restraint stress for 90 min. Statistical analyses were performed according to Mann Whitney-U test, n = 6 in all groups. NS: non-stressed, ARS: acute restraint stress for 90 min.
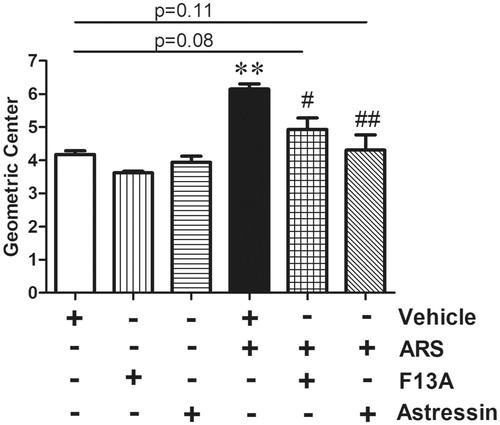
3.2. Cellular localization of CRF immunoreactivity in proximal and distal colon
In the whole thickness tissue sections of the proximal and distal colon from ARS compared to NS rats, CRF immunoreactivity was detected in cells scattered within lamina propria, submucosal and myenteric plexi, while a weak staining was observed in tunica muscularis of proximal colon of NS rats. The circular and longitudinal muscle layers of ARS group rats were immuno-labeled for CRF intensely (). The CRF immunoreactivities in myenteric plexi were quantified in . Compared with NS group, the CRF staining in the distal colonic myenteric plexi of ARS animals was found significantly more intense (38.3 ± 5, F = 8.21, p < .01, n = 3).
Figure 2. Effect of ARS on CRF immunoreactivities in proximal (A-B) and distal (D-E) colonic sections and quantification of CRF staining in NS and ARS-loaded rats (I). The ganglia in myenteric plexus of a NS (G) and ARS-loaded rat (H) are represented as the higher magnification of the boxed regions. Anti-CRF antibody was replaced by antibody diluent in negative controls (C, F). Scale bars represent 100 µm, n = 3 in all groups, p < .01 vs NS). NS: non-stressed, ARS: acute restraint stress for 90 min.
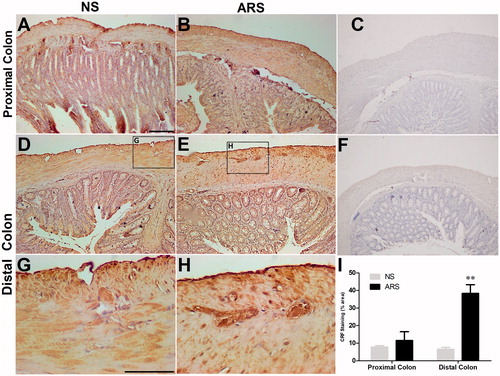
In contrast, no significant effect of the ARS was observed in proximal colonic sections (NS: 7.8 ± 0.8; ARS: 11.5 ± 5, . In negative control sections, the CRF immunostaining was abolished by replacing the primary antibody with PBS ().
3.3. Cellular localization of apelin immunoreactivity in proximal and distal colon
Immuno-florescence for enteric neuronal marker PGP 9.5 was detected uniformly in the myenteric ganglia of both proximal and distal colon coronal sections. Apelin immuno-florescence was detected in the mucosa of both proximal and distal colon as well as tunica muscularis layer of distal colon. The cells exhibited double immunoreactivities for PGP 9.5 and apelin were only observed in distal colonic myenteric ganglia ( insert) which were found more pronounced in preparations obtained from ARS-loaded rats ( insert).
Figure 3. Effect of ARS on apelin (green) immunoreactivity in proximal (A-H) and distal (I-P) coronal colonic sections. PGP9.5 (red) was used to assess the total numbers of neurons in the preparations. TM: tunica muscularis, SM: submucosa, M: mucosa. The scale bars represent 100 µm, n = 3 in all groups. NS: non-stressed, ARS: acute restraint stress for 90 min.
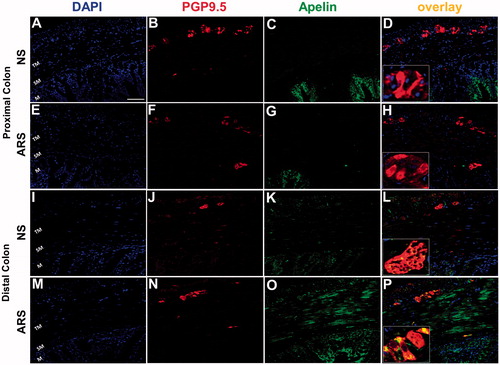
3.4. Effect of ARS on apelin immunoreactivity in distal colonic LMMP preparations
To further demonstrate whether ARS increases the enteric apelin expression, double immunofluorescence labeling of apelin (green) and neuronal marker PGP 9.5 (red) was performed in whole-mount LMMP preparations. LMMP preparations of distal colon were double labeled for PGP 9.5 and apelin supporting the immune-florescence labeling pattern of the coronal sections. The double-positive neurons are enhanced in the distal part of the colon (. The percentage of the double-labeled neurons increased in ARS (8 ± 0.5 vs 40 ± 1.0%cells/ganglion, F = 7.13, p < .01, n = 3) (.
Figure 4. Whole‐mount preparations of distal colonic myenteric neurons obtained from NS (A) and ARS-loaded (B) rats. The quantitative analysis of PGP 9.5 (red)- and apelin (green)-immunoreactive cells (C) in myenteric neurons harvested from distal regions of colon in NS and ARS-loaded rats. Data are means ± SE. **p < .01 vs NS. NS: non-stressed, ARS: acute restraint stress for 90 min. The scale bars represent 200 µm, n = 4 in all groups.
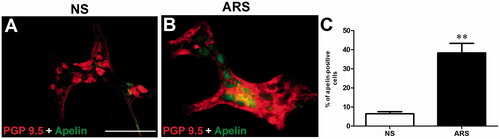
3.5. Cellular localization of APJ immunoreactivity in proximal and distal colon
The APJ receptor was investigated in proximal () and distal () colonic coronal sections. Submucosa and tunica muscularis were immunolabelled uniformly both in proximal and distal colonic sections. However, the APJ immunoreactivity was only observed in the mucosal layer of distal colon. The APJ immunoreactivities in proximal and distal colonic sections were quantified in . The intensities of APJ staining were similar in submucosa (2.3 ± 1.1% in proximal; 3.8 ± 0.6% in distal colon) and tunica muscularis (7 ± 1.8% in proximal; 9.4 ± 0.3% in distal colon) In contrast, compared with proximal colon (1.5 ± 0.2, n = 5), more prominent staining was detected within the mucosal layer of distal colon (8.5 ± 2.4%, F = 7.42, p < .01, n = 5) (.
3.6. Colocalization of apelin and APJ receptor with CRF1 in distal colonic myenteric neurons
To further investigate whether apelin or APJ receptor is colocalized with CRF1 in myenteric neurons, double immunofluorescence labeling was performed in the whole-mount LMMP preparations harvested from the distal colon of the NS rats. LMMP preparations showed uniform CRF1 labeling (red) (), while the apelin positive (green in ) neurons and APJ positive neurons (green in ) in distal colon were observed as a subset of the total cells in ganglia when merged with CRF1 immunolabeling ( and ). Apelin/CRF1 and APJ/CRF1 immunoreactivities in distal colonic myenteric ganglion cells were quantified in .
Figure 6. Proportion of neuronal cells coexpressing of CRF1 with apelin or APJ receptor in distal colonic whole-mount LMMP preparations. PGP 9.5 (blue) was used to assess the total number of neurons in the preparations. Digital merger of B-C and F-G revealed the coexpression of apelin (green)/CRF1 (red) or APJ (green)/CRF1 (red) in myenteric ganglia, respectively. The scale bar represents 100 µm, n = 3 in all groups.
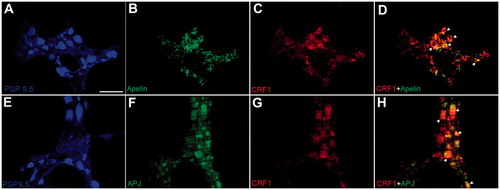
Table 1. Neuronal cells immunoreactive to both CRF1 and apelin or APJ receptor in distal colonic whole-mount LMMP preparations were harvested from NS (n = 4) and ARS-loaded (n = 4) rats.
3.7. In-vivo effect of apelin-13 on colonic motility
In-vivo colonic motility was assessed in anesthetized rats. Following a baseline recording, apelin-13 was administered through an i.v. catheter (100 μg·kg−1, n = 5). Both in proximal and distal colon, immediately after the injection, a rapid stimulatory phase was recorded which was then followed by a long-lasting inhibitory phase characterized with decreased frequency of spontaneous contractions ().
Figure 7. The effect of peripherally administered apelin-13 on in-vivo colonic motility in anesthetized rats. Representative traces showing the response of the proximal (A) and distal (B) colon to apelin-13 (100 μg·kg−1, i.v., n = 5). The black arrow represents the injection performed after recording a basal motor. The gray area and the dashed line represent the rapid stimulatory and long-lasting inhibitory phases, respectively.
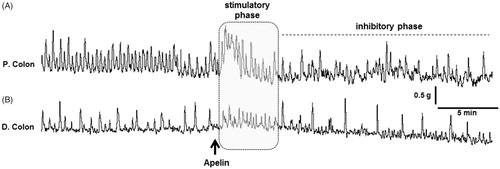
3.8. In-vivo effect of F13A on CRF-induced changes in colonic motility
CRF administration (100 μg·kg−1, i.v.) significantly increased the amplitudes of the spontaneous contractions both in proximal and distal colon. Compared with basal (pre-injection) period, CRF caused a remarkable increase in MI, while the alterations were more intense in the distal colon (275.1 ± 20.4% and 132.6 ± 37.3% distal and proximal colon, respectively). In contrast, the stimulatory action of CRF was significantly (p < .05, n = 5) abolished by preadministration of APJ receptor antagonist F13A both in distal (42.8 ± 15.2%, p < .05, n = 5) and proximal (9.5 ± 19.1, p < .05, n = 5) colon ().
Figure 8. The effect of peripheral CRF administration on in-vivo colonic motility in anesthetized rats. Representative traces showing the response of the proximal and distal colon to CRF (100 μg·kg−1, i.v., n = 5) (A) and the alterations in response to APJ receptor antagonist F13A (300 μg·kg−1, i.v., n = 5) (B). The AUC was calculated as MI within equal pre- and post-injection time periods and expressed as % change (C). The black arrows represent the injections. Data are means ± SE. *p < .05; **p < .01 vs CRF.
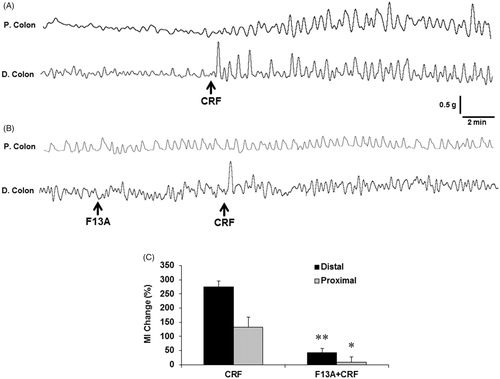
3.9. In-vitro effect of apelin on mechanical activity in colonic strips
In the in-vitro colonic muscle strip study, no muscle effect was observed in response to apelin-13 (10−9–10−7 M), however, when added into the organ bath at 10−6 M concentration, apelin-13 increased the amplitudes of the spontaneous contractions (). Apelin-13 significantly increased the MI (26.83 ± 0.83% in distal colon, p < .05, n = 4) which was more pronounced on the muscle strips obtained from the proximal colon (53.6 ± 0.65%, p < .05, n = 4). Both in proximal and distal colon, the apelin-induced increases in MI were no longer observed when preparations were pretreated with 10−6 M APJ receptor antagonist F13A (2.68 ± 0.86%, 10.20 ± 1.02% in proximal and distal colon preparations, respectively, p < .05, n = 4, . At the end of each protocol, to test whether muscarinic receptors and cholinergic transmission were intact; the preparations were treated with 10−6 M carbachol which caused remarkable high-frequency contractions both in proximal and distal colon ().
Figure 9. In-vitro assessment of the effect of apelin-13 on colonic smooth muscle preparations. Representative traces showing the response of the proximal (A) and distal (B) colon preparations. Pretreatment of F13A (10−6 M) blocked the stimulatory effect of 10−6 M apelin-13 on contractions and MI (C). Treatment of 10−6 M carbachol caused remarkable smooth muscle contractions in both colonic segments. *p < .05; **p < .01 vs lower apelin concentrations, #p < .05; ##p < .01 vs 10−6 M apelin-13, n = 4 in all groups.
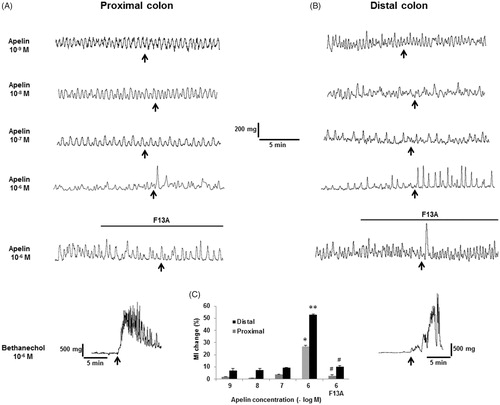
4. Discussion
In the present study, using rats loaded with ARS, our results revealed that exposure to stress induces the upregulation of both CRF and apelin in myenteric ganglia of the distal colon, while no significant immunoreactivity was observed in the proximal part. The ARS-induced acceleration in CT was restored by pretreatment of nonselective CRF receptor antagonist astressin and APJ receptor antagonist F13A. Robust expression of APJ receptor was observed within the submucosal and muscular layers in proximal and distal colon. Apelin and APJ receptors are also investigated in distal colonic myenteric ganglia. Both apelin and APJ receptors are colocalized with CRF1 in the myenteric plexus neurons of distal colon. I.v. administration of apelin-13 exhibited a dual-action pattern on colonic motility which was comprised of a rapid increase in contractions and long-lasting inhibitory phase. Peripheral administration of CRF increased the amplitudes of colonic contractions which were abolished by pretreatment of F13A suggesting that APJ receptors play a pivotal role in CRF-induced alterations in colonic motor functions. Addition of 10−6 M apelin-13 into the organ bath caused increases in the amplitudes of the spontaneous colonic contractions which were no longer observed with pretreatment of F13A.
Consistent with the previous data of other groups (Hussain et al., Citation2017; Larauche et al., 2009; Nozu et al., Citation2013), our CT measurements demonstrated that ARS-induced increased rate of the CT was abolished completely by pretreatment of a nonselective CRF receptor antagonist astressin. On the other hand, using immunofluorescence labeling, we have detected the CRF1 immunoreactivity in colonic myenteric ganglia, suggesting that released CRF from peripheral sources activates myenteric neurons through CRF1 which results in increase in motility. The present immunohistochemical analyses in whole thickness tissue sections revealed that ARS-induced upregulated CRF expression was predominantly observed in distal colon. Apart from myenteric plexus, CRF was expressed in the distal part of the colon with the highest levels in the submucosal and muscle layers in NS rats. In contrary, Yuan and colleagues previously demonstrated a strong immunoreactivity in cells located in the epithelia, crypts and lamina propria in rat proximal colon (Yuan et al., Citation2010) which might be attributed to the sensitivity of antibody used in the particular study.
The wide expression pattern of apelin/APJ receptor system has been demonstrated in peripheral tissues including the GI tract (Fournel et al., Citation2015; Susaki et al., Citation2005; Tatemoto et al., Citation1998; Wang et al., Citation2009). Within gut, the majority of apelin mRNA has been detected in gastric produced exocrine and endocrine cells (Susaki et al., Citation2005; Wang et al., Citation2009) while it was detected in duodenum, jejunum, ileum and colon to a lesser extent (Wang et al., Citation2004). In particular, APJ receptor has been also detected in neurons of the enteric nervous system (Bülbül et al., Citation2019; Fournel et al., Citation2015) suggesting its putative actions in GI tract through a neurocrine/paracrine mechanism at the enteric level. The increased intestinal apelin content has been shown in subjects with inflammatory bowel disease (Han et al., Citation2007), similarly upregulated intestinal expression of apelin has been shown both in rats and mice exposed to experimental colitis (Han et al., Citation2007). In line with the latter data, exposure to WIRS for 360 min has been reported to induce the expression of gastric apelin in rats (Birsen et al., Citation2017; Izgut-Uysal et al., Citation2014). Apart from the stressed conditions, little is known regarding the effect of peripheral apelin on GI motor functions, however, we have recently demonstrated in rats that exogenous apelin-13 (100 µg, i.p.) delayed CT which was completely reversed by preadministration of APJ receptor antagonist F13A (Bülbül et al., Citation2017).
In the present study, we investigated the stress-induced alterations in colonic motor functions which are predominantly regulated by central autonomic and enteric neuronal pathways. Our histological data demonstrated the expression of apelin in colonic myenteric ganglia which exhibited a remarkable increase upon stress. On the other hand, within GI tract, apelin and APJ receptor are produced by enterocytes (Wang et al., Citation2004, Citation2009), furthermore, apelin has been previously demonstrated to be released in the lumen by enterocytes in response to glucose to aid glucose absorption (Dray et al., Citation2013). Indeed, numerous humoral factors could reach the enteric neurons to modulate their activities. These molecules could be transported through circulation in an endocrine fashion, while others could act from the intestinal lumen which are able to be transcytosed from the lumen (Fournel et al., Citation2015) Despite, our data demonstrated that colonic myenteric neurons coexpress apelin with CRF1, it appears to be reasonable that secreted apelin by enterocytes upon stress could reach the myenteric neurons in a paracrine fashion which in turn acts through APJ receptors in musculature and/or enteric neurons.
To test whether peripheral exogenous apelin alters colonic motility, we recorded in-vivo proximal and distal colonic motility in anesthetized rats. Interestingly, immediately after the i.v. administration of apelin-13 a rapid stimulatory effect on colonic spontaneous contractions was observed. In fact, the action of apelin on GI musculature has not been identified. The APJ signaling has been considered to be mediated by Gi/o and/or Gq/11 coupling (Masri, Morin, Pedebernade, Knibiehler, & Audigier, Citation2006). Additionally, using vascular smooth muscle cells, Hashimoto and colleagues previously demonstrated that apelin/APJ signaling is coupled to pertussis toxin-sensitive G proteins that mediate phosphorylation of the myosin light chain, a major regulatory event in initiating smooth muscle contraction (Hashimoto et al., Citation2006). Therefore, the rapid stimulatory phase occurred following apelin injection could be explained by a direct action of apelin-13 on smooth muscle cells that initiates rapid contractions. The present in-vivo motility recordings demonstrated that apelin-13 induced a long-lasting inhibitory phase that produced a late-onset reduction in the frequency of the colonic contractions. The lack of the relevant inhibitory phase in the in-vitro recordings performed in the colonic muscle strips suggests a contribution of an endocrine or paracrine mediator. In rodents, apelin-13 has been previously demonstrated to stimulate the release of cholecystokinin (CCK) (Flemstrom, Makela, & Purhonen, Citation2011; Wattez et al., Citation2013), a well-defined alimentary hormone with its inhibitory actions on GI motor functions, suggesting that peripheral apelin may alter the GI motor functions through the mediation of CCK.
The present data obtained from in-vitro organ bath studies have revealed that apelin-13 at a dose of 10−6 M produced smooth muscle contractions through APJ receptor, while the observed response was greater in distal colon preparations. In contrast to our results, Yang and colleagues previously tested the in-vitro effects of apelin-13 on mouse distal colon preparations and observed no response to 10−8–10−6 M concentrations. In fact, species differences in the APJ distribution have been reported (Pope, Roberts, Lolait, & O’Carroll, Citation2012), therefore, we cannot exclude the possibility that exogenous apelin-induced alterations in colon could exhibit a variation due to the intensity of the APJ receptor expression.
Taken together, our present data demonstrated that ARS-induced acceleration in CT was restored by peripheral preadministration of astressin or F13A. Moreover, apelin immunoreactivity in colonic myenteric ganglia was increased remarkably by ARS, whereas CRF1 was found to be coexpressed both with APJ receptor and apelin in colonic myenteric neurons suggesting the role of apelinergic system in neurocrine fashion. Therefore, the enteric apelinergic system and CRF1 appear to be responsible for the alterations predominantly in distal colonic motility under stressed conditions. APJ receptor antagonism appears to be a potential pharmacological strategy for the treatment of stress-related GI motor disorders including IBS.
Disclosure statement
No conflicts of interest were reported by the authors.
Additional information
Funding
References
- Babygirija, R., Zheng, J., Bülbül, M., Cerjak, D., Ludwig, K., & Takahashi, T. (2010). Sustained delayed gastric emptying during repeated restraint stress in oxytocin knockout mice. Journal of Neuroendocrinology, 22, 1181–1186. doi:10.1111/j.1365-2826.2010.02069.x
- Barreau, F., Cartier, C., Leveque, M., Ferrier, L., Moriez, R., Laroute, V., … Bueno, L. (2007). Pathways involved in gut mucosal barrier dysfunction induced in adult rats by maternal deprivation: Corticotrophin-releasing factor and nerve growth factor interplay. The Journal of Physiology, 580, 347–356. 1doi:10.1113/jphysiol.2006.120907
- Birsen, İ., Gemici, B., Acar, N., Üstünel, İ., & İzgüt-Uysal, V.N. (2017). The role of apelin in the healing of water-immersion and restraint stress-induced gastric damage. The Journal of Physiological Sciences, 67, 373–385. doi:10.1007/s12576-016-0469-9
- Bulbul, M., Babygirija, R., & Cerjak, D. (2012). Impaired adaptation of gastrointestinal motility following chronic stress in maternally separated rats [Research Support, Non-U.S. Gov't]. American Journal of Physiology-Gastrointestinal and Liver Physiology, 302, G702–11. doi:10.1152/ajpgi.00447.2011
- Bulbul, M., Izgut-Uysal, V.N., & Sinen, O. (2016). Central apelin mediates stress-induced gastrointestinal motor dysfunction in rats [Research Support, Non-U.S. Gov't]. American Journal of Physiology-Gastrointestinal and Liver Physiology, 310, G249–61. doi:10.1152/ajpgi.00145.2015
- Bülbül, M., Sinen, O., Birsen, İ., & Izgüt-Uysal, V. (2017). Peripheral apelin-13 administration inhibits gastrointestinal motor functions in rats: The role of cholecystokinin through CCK1 receptor-mediated pathway. Neuropeptides, 63, 91–97. doi:10.1016/j.npep.2016.12.001
- Bülbül, M., Sinen, O., İzgüt-Uysal, V.N., Akkoyunlu, G., Öztürk, S., & Uysal, F. (2019). Peripheral apelin mediates stress-induced alterations in gastrointestinal motor functions depending on the nutritional status. Clinical and Experimental Pharmacology and Physiology, 46, 29–39. doi:10.1111/1440-1681.13032
- Chatzaki, E., Crowe, P.D., Wang, L., Million, M., Tache, Y., & Grigoriadis, D.E. (2004). CRF receptor type 1 and 2 expression and anatomical distribution in the rat colon [Research Support, U.S. Gov't, P.H.S.]. Journal of Neurochemistry, 90, 309–316. doi:10.1111/j.1471-4159.2004.02490.x
- Dodiya, H.B., Forsyth, C.B., & Voigt, R.M. (2018). Chronic stress-induced gut dysfunction exacerbates Parkinson's disease phenotype and pathology in a rotenone-induced mouse model of Parkinson's disease [Review]. Neurobiology of Disease, 21. doi:10.1016/j.nbd.2018.12.012
- Dray, C., Sakar, Y., Vinel, C., Daviaud, D., Masri, B., Garrigues, L., … Ducroc, R. (2013). The intestinal glucose-apelin cycle controls carbohydrate absorption in mice [Comparative Study Research Support, Non-U.S. Gov't]. Gastroenterology, 144, 771–780. doi:10.1053/j.gastro.2013.01.004
- Estienne, M., Claustre, J., Clain-Gardechaux, G., Paquet, A., Taché, Y., Fioramonti, J., & Plaisancié, P. (2010). Maternal deprivation alters epithelial secretory cell lineages in rat duodenum: Role of CRF-related peptides. Gut, 59, 744–751. doi:10.1136/gut.2009.190728
- Farhin, S., Wong, A., Delungahawatta, T., Amin, J.Y., Bienenstock, J., Buck, R., & Kunze, W.A. (2019). Restraint stress induced gut dysmotility is diminished by a milk oligosaccharide (2'-fucosyllactose) in vitro. PLoS One, 14, e0215151. doi:10.1371/journal.pone.0215151
- Flemstrom, G., Makela, K., & Purhonen, A.K. (2011). Apelin stimulation of duodenal bicarbonate secretion: Feeding-dependent and mediated via apelin-induced release of enteric cholecystokinin [Research Support, Non-U.S. Gov't]. Acta Physiologica, 201, 141–150. doi:10.1111/j.1748-1716.2010.02175.x
- Fournel, A., Drougard, A., & Duparc, T. (2015). Apelin targets gut contraction to control glucose metabolism via the brain. Gut, 66(2), 258–269. doi:10.1136/gutjnl-2015-310230
- Han, S., Wang, G., Qiu, S., de la Motte, C., Wang, H.-Q., Gomez, G., … Greeley, G.H. (2007). Increased colonic apelin production in rodents with experimental colitis and in humans with IBD [Research Support, N.I.H., Extramural Research Support, Non-U.S. Gov't]. Regulatory Peptides, 142, 131–137. doi:10.1016/j.regpep.2007.02.002
- Hashimoto, T., Kihara, M., Ishida, J., Imai, N., Yoshida, S-I., Toya, Y., … Umemura, S. (2006). Apelin stimulates myosin light chain phosphorylation in vascular smooth muscle cells [Research Support, Non-U.S. Gov't]. Arteriosclerosis, Thrombosis, and Vascular Biology, 26, 1267–1272. Jundoi:10.1161/01.ATV.0000218841.39828.91
- Hussain, Z., Kim, H.W., Huh, C.W., Lee, Y.J., & Park, H. (2017). The effect of peripheral CRF peptide and water avoidance stress on colonic and gastric transit in Guinea pigs. Yonsei Medical Journal, 58, 872–877. doi:10.3349/ymj.2017.58.4.872
- Izgut-Uysal, V.N., Gemici, B., & Birsen, I. (2014). The protective effect of apelin against water-immersion and restraint stress-induced gastric damage [Research Support, Non-U.S. Gov't]. The Journal of Physiological Sciences, 64, 279–289. doi:10.1007/s12576-014-0317-8
- Kimura, T., Amano, T., Uehara, H., Ariga, H., Ishida, T., Torii, A., … Yamato, S. (2007). Urocortin I is present in the enteric nervous system and exerts an excitatory effect via cholinergic and serotonergic pathways in the rat colon. American Journal of Physiology-Gastrointestinal and Liver Physiology, 293, G903–G910. doi:10.1152/ajpgi.00066.2007
- Larauche, M., Gourcerol, G., Wang, L., Pambukchian, K., Brunnhuber, S., Adelson, D.W., … Taché, Y. (2009). Cortagine, a CRF1 agonist, induces stresslike alterations of colonic function and visceral hypersensitivity in rodents primarily through peripheral pathways [Comparative Study Research Support, N.I.H., Extramural Research Support, U.S. Gov't, Non-P.H.S.]. American Journal of Physiology-Gastrointestinal and Liver Physiology, 297, G215–27. doi:10.1152/ajpgi.00072.2009
- Liu, S., Ren, W., Qu, M.-H., Bishop, G.A., Wang, G.-D., Wang, X.-Y., … Wood, J.D. (2010). Differential actions of urocortins on neurons of the myenteric division of the enteric nervous system in guinea pig distal colon [Research Support, N.I.H., Extramural Research Support, Non-U.S. Gov't]. British Journal of Pharmacology, 159, 222–236. doi:10.1111/j.1476-5381.2009.00516.x
- Martinez, V., Wang, L., & Million, M. (2004). Urocortins and the regulation of gastrointestinal motor function and visceral pain [Research Support, U.S. Gov't, Non-P.H.S. Research. Peptides, 25, 1733–1744. doi:10.1016/j.peptides.2004.05.025
- Martinez, V., Wang, L., & Rivier, J. (2004). Central CRF, urocortins and stress increase colonic transit via CRF1 receptors while activation of CRF2 receptors delays gastric transit in mice [Research Support, Non-U.S. Gov't Research Support, U.S. Gov't, P.H.S.]. The Journal of Physiology, 556, 221–234. doi:10.1113/jphysiol.2003.059659
- Masere, C., Nakade, Y., Zheng, J., Babygirija, R., Ludwig, K., & Takahashi, T. (2009). Chronic restraint stress has no more stimulatory effects on colonic motility in rats. Neuroscience Letters, 453, 147–150. doi:10.1016/j.neulet.2009.02.042
- Masri, B., Morin, N., Pedebernade, L., Knibiehler, B., & Audigier, Y. (2006). The apelin receptor is coupled to Gi1 or Gi2 protein and is differentially desensitized by apelin fragments [Comparative Study Research Support, Non-U.S. Gov't]. Journal of Biological Chemistry, 281, 18317–18326. doi:10.1074/jbc.M600606200
- Miampamba, M., Maillot, C., Million, M., & Taché, Y. (2002). Peripheral CRF activates myenteric neurons in the proximal colon through CRF(1) receptor in conscious rats [Research Support, Non-U.S. Gov't Research Support, U.S. Gov't, P.H.S.]. American Journal of Physiology-Gastrointestinal and Liver Physiology, 282, G857–G865. doi:10.1152/ajpgi.00434.2001
- Million, M., Zhao, J.F., & Luckey, A. (2013). The newly developed CRF1-receptor antagonists, NGD 98-2 and NGD 9002, suppress acute stress-induced stimulation of colonic motor function and visceral hypersensitivity in rats [Research Support, N.I.H., Extramural Research Support, Non-U.S. Gov't Research Support, U.S. Gov't, Non-P.H.S.]. PLoS One, 8, e73749. doi:10.1371/journal.pone.0073749
- Nakade, Y., Tsuchida, D., Fukuda, H., Iwa, M., Pappas, T.N., & Takahashi, T. (2006). Restraint stress augments postprandial gastric contractions but impairs antropyloric coordination in conscious rats [Research Support, N.I.H., Extramural]. American Journal of Physiology-Regulatory, Integrative and Comparative Physiology, 290, R616–24. doi:10.1152/ajpregu.00161.2005
- Newson, M.J.F., Roberts, E.M., Pope, G.R., Lolait, S.J., & O'Carroll, A.-M. (2009). The effects of apelin on hypothalamic-pituitary-adrenal axis neuroendocrine function are mediated through corticotrophin-releasing factor- and vasopressin-dependent mechanisms [Research Support, Non-U.S. Gov't]. Journal of Endocrinology, 202, 123–129. doi:10.1677/JOE-09-0093
- Nozu, T., Kumei, S., Takakusaki, K., & Okumura, T. (2014). Water-avoidance stress enhances gastric contractions in freely moving conscious rats: Role of peripheral CRF receptors [Research Support, Non-U.S. Gov't]. Journal of Gastroenterology, 49, 799–805. doi:10.1007/s00535-013-0828-8
- Nozu, T., Tsuchiya, Y., Kumei, S., Takakusaki, K., & Okumura, T. (2013). Peripheral corticotropin-releasing factor (CRF) induces stimulation of gastric contractions in freely moving conscious rats: Role of CRF receptor types 1 and 2 [Research Support, Non-U.S. Gov't]. Neurogastroenterology & Motility, 25, 190–197. doi:10.1111/nmo.12050
- Ochi, M., Tominaga, K., Tanaka, F., Tanigawa, T., Shiba, M., Watanabe, T., … Arakawa, T. (2008). Effect of chronic stress on gastric emptying and plasma ghrelin levels in rats. Life Sciences, 82, 862–868. doi:10.1016/j.lfs.2008.01.020
- Pope, G.R., Roberts, E.M., Lolait, S.J., & O’Carroll, A.-M. (2012). Central and peripheral apelin receptor distribution in the mouse: Species differences with rat [Research Support, Non-U.S. Gov't]. Peptides, 33, 139–148. doi:10.1016/j.peptides.2011.12.005
- Rodino-Janeiro, B.K., Alonso-Cotoner, C., & Pigrau, M. (2015). Role of corticotropin-releasing factor in gastrointestinal permeability. Journal of Neurogastroenterology and Motility, 21, 33–50. doi:10.5056/jnm14084
- Susaki, E., Wang, G., Cao, G., Wang, H.-Q., Englander, E.W., & Greeley, G.H. (2005). Apelin cells in the rat stomach [Research Support, N.I.H., Extramural Research Support, U.S. Gov't, P.H.S.]. Regulatory Peptides, 129, 37–41. doi:10.1016/j.regpep.2005.01.013
- Tache, Y., & Bonaz, B. (2007). Corticotropin-releasing factor receptors and stress-related alterations of gut motor function [Research Support, N.I.H., Extramural Research Support, Non-U.S. Gov't [Research Support, U.S. Gov't, Non-P.H.S.Review]. Journal of Clinical Investigation, 117, 33–40. doi:10.1172/JCI30085
- Tache, Y., Kiank, C., & Stengel, A. (2009). A role for corticotropin-releasing factor in functional gastrointestinal disorders [Research Support, N.I.H., Extramural Research Support, Non-U.S. Gov't Research Support, U.S. Gov't, Non-P.H.S.Review]. Current Gastroenterology Reports, 11, 270–277. doi:10.1007/s11894-009-0040-4
- Tache, Y., Larauche, M., & Yuan, P.Q. (2018). Brain and gut CRF signaling: Biological actions and role in the gastrointestinal tract [Research Support, N.I.H., Extramural Research Support, U.S. Gov't, Non-P.H.S. Review]. Current Molecular Pharmacology, 11, 51–71.
- Tache, Y., Martinez, V., & Million, M. (2001). Stress and the gastrointestinal tract III. Stress-related alterations of gut motor function: Role of brain corticotropin-releasing factor receptors [Research Support, U.S. Gov't, P.H.S. Review]. American Journal of Physiology-Gastrointestinal and Liver Physiology, 280, G173–G177. doi:10.1152/ajpgi.2001.280.2.G173
- Tache, Y., & Million, M. (2015). Role of corticotropin-releasing factor signaling in stress-related alterations of colonic motility and hyperalgesia. Journal of Neurogastroenterology and Motility, 21, 8–24. doi:10.5056/jnm14162
- Tatemoto, K., Hosoya, M., Habata, Y., Fujii, R., Kakegawa, T., Zou, M.-X., … Fujino, M. (1998). Isolation and characterization of a novel endogenous peptide ligand for the human APJ receptor. Biochemical and Biophysical Research Communications, 251, 471–476. doi:10.1006/bbrc.1998.9489
- Wang, G., Anini, Y., Wei, W., Qi, X., O’Carroll, A.-M., Mochizuki, T., … Greeley, G.H. (2004). Apelin, a new enteric peptide: Localization in the gastrointestinal tract, ontogeny, and stimulation of gastric cell proliferation and of cholecystokinin secretion [Research Support, U.S. Gov't, P.H.S.]. Endocrinology, 145, 1342–1348. doi:10.1210/en.2003-1116
- Wang, G., Kundu, R., Han, S., Qi, X., Englander, E.W., Quertermous, T., & Greeley, G.H. (2009). Ontogeny of apelin and its receptor in the rodent gastrointestinal tract. Regulatory Peptides, 158, 32–39. doi:10.1016/j.regpep.2009.07.016
- Wattez, J.-S., Ravallec, R., Cudennec, B., Knauf, C., Dhulster, P., Valet, P., … Lesage, J. (2013). Apelin stimulates both cholecystokinin and glucagon-like peptide 1 secretions in vitro and in vivo in rodents [Research Support, Non-U.S. Gov't]. Peptides, 48, 134–136. doi:10.1016/j.peptides.2013.08.005
- Yuan, P.Q., Million, M., & Wu, S.V. (2007). Peripheral corticotropin releasing factor (CRF) and a novel CRF1 receptor agonist, stressin1-A activate CRF1 receptor expressing cholinergic and nitrergic myenteric neurons selectively in the colon of conscious rats [Research Support, N.I.H., Extramural Research Support, U.S. Gov't, Non-P.H.S.]. Neurogastroenterology & Motility, 19, 923–936. doi:10.1111/j.1365-2982.2007.00978.x
- Yuan, P.Q., Wu, V., & Chang, L. (2007). Cellular localization of corticotropin-releasing factor (CRF) receptor 1 (CRF1) in human colon and its expression in the jejunum and colon of irritable bowel syndrome (IBS) female and male patients. Gastroenterology, 132, A190–A190.
- Yuan, P.-Q., Wu, S.V., Wang, L., & Taché, Y. (2010). Corticotropin releasing factor in the rat colon: Expression, localization and upregulation by endotoxin [Research Support, N.I.H., Extramural Research Support, U.S. Gov't, Non-P.H.S.]. Peptides, 31, 322–331. doi:10.1016/j.peptides.2009.11.012
- Zheng, J., Dobner, A., Babygirija, R., Ludwig, K., & Takahashi, T. (2009). Effects of repeated restraint stress on gastric motility in rats. American Journal of Physiology. Regulatory, Integrative and Comparative Physiology, 296, R1358–65. doi:10.1152/ajpregu.90928.2008