Abstract
Circadian rhythms are ∼24 h fluctuations in physiology and behavior that are synchronized with the light-dark cycle. The circadian system ensures homeostatic balance by regulating multiple systems that respond to environmental stimuli including stress systems. In rats, acute exposure to a series of uncontrollable tailshocks (inescapable stress, IS) produces an anxiety and depression-like phenotype. Anxiety- and fear-related behavioral changes produced by IS are driven by sensitization of serotonergic (5-hydroxytryptamine, 5-HT) neurons in the dorsal raphe nucleus (DRN). Because the circadian and serotonergic systems are closely linked, here we tested whether the DRN-dependent behavioral and neurochemical effects of IS are time of day dependent. Exposure to IS during the light (inactive) phase elicited the expected changes in mood related behaviors. In contrast, rats that underwent IS during the dark (active) phase were buffered against stress-induced changes in juvenile social exploration and shock-elicited freezing, both DRN-dependent outcomes. Interestingly, behavioral anhedonia, which is not a DRN-dependent behavior, was comparably reduced by stress at both times of day. Neurochemical changes complimented the behavioral results: IS-induced activation of DRN 5-HT neurons was greater during the light phase compared to the dark phase. Additionally, 5-HT1AR and 5-HTT, two genes that regulate 5-HT activity were up-regulated during the middle of the light cycle. These data suggest that DRN-dependent behavioral outcomes of IS are time of day dependent and may be mediated by circadian gating of the DRN response to stress.
Lay summary
Here we show that the time of day at which a stressor occurs impacts the behavioral and neurochemical outcomes of the stressor. In particular, animals appear more vulnerable to a stressor that occurs during their rest phase. This work may have important implications for shift-workers and other populations that are more likely to encounter stressors during their rest phase. |
Introduction
Traumatic life events can predispose individuals to a wide range of somatic and neuropsychiatric disorders. In particular, stress is the greatest known risk factor for development of anxiety disorders and depression. Importantly, these disorders are highly comorbid, affecting nearly 25 million individuals worldwide with a lifetime prevalence rate of 21–28% (Kessler et al., Citation2005). However, not all individuals who encounter a stressor acquire psychopathology. Thus, it is of clinical importance to investigate environmental factors that may impact stress vulnerability and resistance/resilience.
Patients with anxiety disorders and depression often display exaggerated anxiety and fear in response to innocuous or mildly aversive stimuli, and these symptoms can be modeled in rodents following exposure to a severe acute stressor. For example, rats subjected to a series of uncontrollable tailshocks (inescapable stress; IS) exhibit reduced social interest, exaggerated fear conditioning, increased post-shock freezing, as well as numerous other changes [reviewed in (Maier & Seligman, Citation2016)]. Many of these behaviors are driven by complex modifications in the serotonergic (5-hydroxytryptamine, 5-HT) system. IS potently activates 5-HT neurons in the mid-caudal dorsal raphe nucleus [DRN (Grahn et al., Citation1999)], causing release of 5-HT within the DRN itself via axon collaterals and in forebrain projection sites that mediate affective behavior (Hale, Shekhar, & Lowry, Citation2012). Prolonged elevation of extracellular 5-HT within the DRN desensitizes DRN 5-HT1A autoreceptors (Rozeske et al., Citation2011), thereby sensitizing 5-HT circuits such that later testing conditions elicit an exaggerated 5-HT response (Amat, Matus-Amat, Watkins, & Maier, Citation1998; Christianson et al., Citation2010). DRN 5-HT hyperarousal is indeed critical for the expression of these behaviors as manipulations that increase DRN activity reproduce IS behavioral outcomes, whereas manipulations that inhibit the DRN prevent them (Christianson et al., Citation2008; Maier, Grahn, & Watkins, Citation1995).
Importantly, the 5-HT system is under circadian control (reviewed in (Daut & Fonken, Citation2019)). The circadian system organizes physiology and behavior across the light-dark cycle in a manner that promotes homeostasis. Circadian (∼24 h) rhythms are initiated in the master clock, located in the suprachiasmatic nucleus (SCN) of the anterior hypothalamus. Timing information is communicated to extra-SCN clocks present in virtually every tissue in the body via neural and humoral routes (Albrecht, Citation2012). The hypothalamic-pituitary-adrenal (HPA) axis plays a major role in entraining some extra-SCN clocks. For example, DRN 5-HT neurons express glucocorticoid receptors (GRs) (Harfstrand et al., Citation1986), and 5-HT synthesis is driven endogenously and modulated by the daily glucocorticoid (CORT) surge (Malek, Sage, Pévet, & Raison, Citation2007). As a result, 5-HT content in the DRN and forebrain projection sites fluctuates across the light-dark cycle, peaking during the active phase in both nocturnal and diurnal species (Barassin et al., Citation2002; Cagampang & Inouye, Citation1994).
The time of stressor onset relative to the diurnal phase appears to be an important factor in determining some stress outcomes (Aslani et al., Citation2014; Cohen et al., Citation2015; Fonken et al., Citation2016; Koch et al., Citation2016; Retana-Marquez, Citation2003; Rybkin, Citation1997). For example, chronic social stress during the light phase induced more “detrimental effects” (e.g. greater weight loss, increased HPA axis activity) than dark phase stress in mice (Koch et al., Citation2016). Furthermore, exposure to a severe acute stressor (10 min predatory scent stress) at the onset of the light (versus dark) phase induced greater stress-associated behavioral changes (Cohen et al., Citation2015). Furthermore, our lab previously demonstrated that rats are resistant to IS-induced changes in immunoregulation when stress and a subsequent inflammatory challenge occurred during the active phase (Fonken et al., Citation2016). However, whether there are time of day differences in vulnerability to IS-induced behavioral changes was unknown and the mechanisms mediating diurnal differences in vulnerability to stressors are not well established. Because DRN 5-HT activity is diurnally modulated, and many of the behavioral sequelae of IS are driven by changes in DRN 5-HT neurons, we hypothesized that: (1) the behavioral effects of IS may depend on the time of day at which the stressor occurs and, (2) changes in DRN 5-HT may underlie diurnal differences in stress susceptibility.
Methods and materials
Animals
Adult male Sprague-Dawley rats (ENVIGO, Indianapolis, IN) rats weighing 225–250 g upon arrival were used in these experiments. Rats were pair-housed (unless otherwise specified) in polycarbonate cages (47 x 23 x 20 cm) in light, temperature, humidity-controlled rooms. Food and water were available ad libitum except during the inescapable stressor procedure and behavioral testing. Rats were given two weeks to acclimate to colony conditions before experimentation began. All rats were maintained on a 12:12 light: dark (LD) cycle with lights on at 0700 [Zeitgeber time 0 (ZT0)]. All experimental procedures conducted during the dark phase used dim red light. All experimental procedures were approved by the University of Colorado Institutional Animal Care and Use Committee.
Experimental design
Experiment 1: does time of stressor exposure modulate the behavioral consequences of stress?
Exposure to IS during the light phase consistently increases anxiety- and depressive-like responses (Maier & Seligman, Citation2016). The DRN is the proximate mediator of the IS-induced reduction in juvenile social exploration (Christianson et al., Citation2008) and exaggerated fear (Maier et al., Citation1993), but not behavioral anhedonia (Christianson et al., Citation2008). Importantly, there are circadian differences in DRN neural activation (Janusonis & Fite, Citation2001). Thus, here we investigated whether time of stressor exposure modulates DRN-dependent and independent behavioral outcomes of IS. To do this, rats were exposed to IS during the middle of the light phase [ZT5-7, (ZT6)] or the middle of the dark phase [ZT15-17, (ZT16)] and then evaluated for changes in sucrose preference, juvenile social exploration, and shock-elicited freezing. Rats were first assessed for baseline sucrose preference during the first 4 h of the dark phase (ZT12-ZT16) on the two days preceding stress. A baseline juvenile social exploration score was obtained 24 h prior to stress. IS-induced changes in sucrose preference were assessed for the first 4 h of each dark phase (ZT12-16) following stress until responding returned to baseline. Note that each post-stress sucrose preference test occurred at different time intervals for ZT6 and ZT16 groups. For example, the first post-stress sucrose preference test occurred 5 h or 19 h post-stress for ZT6 and ZT16 groups, respectively. Next, rats were evaluated for juvenile social exploration 24 h following IS. A separate group of rats were stressed at ZT16 and evaluated for juvenile social exploration 36 h later at ZT6 to dissociate the effect of time of stressor from time of testing. Of note, we did not include a group that was stressed at ZT6 and tested at ZT16 in order to reduce animal use and because time of day differences in juvenile social exploration was not apparent at ZT6 versus ZT16. Finally, rats were evaluated for shock-elicited freezing in a shuttlebox 48 h following IS. The IS procedure and all behavioral testing were performed in the dark under dim red light (see ).
Figure 1. Timeline for experiment 1. Rats acclimated to the facility for two weeks after arrival. Rats underwent baseline testing in sucrose preference (SP) and juvenile social exploration (JSE) tests on the 2 days prior to stress. On experimental Day 0 (D0) rats underwent 100 inescapable tailshocks (inescapable stress; IS) or home cage (HC) control treatment either during the middle of the dark phase or middle of the light phase. Rats were subsequently tested on the SP, JSE and shock-elicited freezing tests.
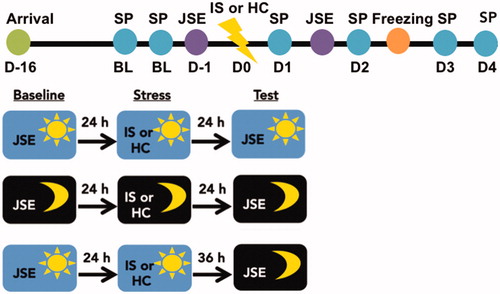
Experiment 2: does time of stressor exposure modulate the HPA axis response to stress?
Acute and chronic stress-induced changes in HPA axis activity mediate some anxiety and depressive-like behaviors in rodents. Exposure to IS (during the light phase) produces a robust acute increase in glucocorticoids. In addition, IS increases basal CORT for 48–96 h following stressor termination, but only at the nadir of the circadian CORT rhythm (Fleshner et al., Citation1995). Thus, glucocorticoid-responsive targets are exposed to high levels of CORT for several days following stress. Previously, we showed that the time of stressor exposure modulates the magnitude of the acute IS-induced CORT response (Fonken et al., Citation2016). Thus, here we investigated whether the stress-induced increase in basal CORT is modulated by the time of stressor exposure. To examine this, rats were exposed to IS or no stress (home cage; HC) at ZT6 or ZT16 and sacrificed 24 h after stress. Trunk blood was collected, serum separated, and CORT concentrations assayed using ELISA (see below).
Experiment 3: do DRN 5-HT factors vary across the light-dark cycle?
DRN 5-HT neuronal activity is regulated by a number of factors involved in the synthesis, release, and reuptake of 5-HT: (1) The TPH2 gene is highly expressed in the DRN and encodes for tryptophan hydroxylase, the rate-limiting enzyme in 5-HT synthesis (Walther, Citation2003); (2) 5-HT1A autoreceptors (5-HT1ARs) are expressed on the soma and dendrites of DRN 5-HT neurons, and upon stimulation, provide negative feedback that inhibits 5-HT release (Stamford, Davidson, McLaughlin, & Hopwood, Citation2000); (3) The serotonin transporter (5-HTT) is responsible for the reuptake of 5-HT back into presynaptic neurons (Horschitz, Hummerich, & Schloss, Citation2001). Abnormal gene expression of each of these factors has been observed in postmortem brains of patients diagnosed with anxiety and/or depression (Boldrini, Underwood, Mann, & Arango, Citation2005). Furthermore, in rodents, exposure to some acute and chronic stress paradigms can alter the expression of these factors, which in turn, may exacerbate stress vulnerability (Donner et al., Citation2018). Here we investigated whether there are time of day differences in the basal expression of these factors. In Experiment 3.1, a separate cohort of stress naive rats was sacrificed every 6 h across the light-dark cycle (ZT0, ZT6, ZT12, ZT16), brains were extracted, and DRN TPH2 (tryptophan hydroxylase), HT1AR (5-HT1AR), and SLC64A (5-HTT) mRNA was assessed using quantitative real-time PCR (see below).
Experiment 4: does time of stressor exposure modulate the neurochemical consequences of stress?
Exposure to IS during the light phase robustly activates DRN 5-HT neurons (Grahn et al., Citation1999) resulting in elevated extracellular 5-HT in the DRN itself as well as in projection regions (Maswood, Barter, Watkins, & Maier, Citation1998). Here we hypothesized that time of day differences in stress susceptibility may be driven, in part, by differential activation of DRN 5-HT neurons during stress at ZT6 versus ZT16. To test this, in Experiment 3.1, rats were exposed to IS or control treatment (HC) during the middle of the light (ZT6) or dark (ZT16) phase and sacrificed approximately 2 h after stress. Previous studies indicate that this is the optimal time point to characterize IS-induced DRN 5-HT changes (Grahn et al., Citation1999). Immunohistochemistry for tryptophan hydroxylase (a marker of 5-HT synthesizing neurons) and c-Fos (a marker of neuronal activation) was performed to quantitate DRN 5-HT activity (see below).
General methods
Inescapable stressor procedure
Rats were placed in Plexiglas tubes (18 cm in length x 8 cm in diameter) with a Plexiglas rod protruding from the rear to which the rat’s tail was taped and affixed with two copper electrodes. Rats were subjected to 100 trials (33 trials at 1.0 mA, 33 trials at 1.3 mA, 34 trials at 1.6 mA) of intermittent 5 sec inescapable tailshocks with a variable inter-trial interval ranging from 30–90 s (average of 60 s). The average duration of this procedure is approximately 100 min. Rats were immediately returned to their home cages upon termination of the final tailshock. During stress, HC control rats remained undisturbed in the colony room.
Sucrose preference
Sucrose preference is a reliable measure of hedonic status in rodents (Matthews, Forbes, & Reid, Citation1995), where reductions in sucrose intake indicate behavioral anhedonia (i.e. a lack of interest in rewarding stimuli). To determine whether time of stressor exposure differentially affects sucrose preference, rats were provided with two solutions, water or water supplemented with 2% sucrose. Rats were acclimated to the bottles for two consecutive days prior to stress for 4 h each during the light phase (ZT2-6) and dark phase (ZT12-16) and an average baseline score was calculated. Not surprisingly, rats developed a strong sucrose preference during the dark phase (91%), but not during the light phase (49%) when drinking behavior is minimal. Rats did not have access to bottles during stress or behavioral testing. The position (left/right) of the bottles was counterbalanced across sessions to prevent preferential responding to one side. Because baseline values in sucrose consumption were low during the light phase, sucrose preference was only evaluated during the first 4 h of the dark phase each night following stress. A percentage of relative 2% sucrose preference was calculated as follows: [2% sucrose consumed/(2% sucrose consumed + water consumed)] x 100. One IS and one HC rat did not establish a reliable baseline sucrose preference (greater than 65%) and were excluded from analysis.
Juvenile social exploration test
To evaluate propensity to engage in a social interaction, an anxiety-like measure, a juvenile social exploration test was performed as previously described (Christianson et al., Citation2008) at 24 or 36 h after stress at either ZT6 or ZT16. Briefly, each rat was placed in a novel cage for 60 min prior to introduction of a novel male juvenile conspecific (28 ± 3 day old). An observer, blind to treatment, scored investigative behavior (e.g. allogrooming, sniffing, pinning, and following) initiated by the adult experimental rat during a 3 min period using a program designed in LATEX. A baseline juvenile social exploration score was established 24 h prior to stress using a different juvenile conspecific.
Shock-elicited freezing
Freezing after footshock is a measure of fear associated with the cues present in the testing apparatus, rather than an unconditioned response to the footshock itself (Fanselow, Lester, & Helmstetter, Citation1988). To evaluate fear, rats were placed in an illuminated two-way shuttlebox (red illumination during the dark phase). After a 5 min acclimation period, rats received two fixed ratio-1 (FR-1) escape trials, which each consisted of a 0.8 mA shock delivered to the grid floor of the shuttlebox. The shock terminated when the rat made a single crossing of the shuttlebox divider. Note that IS rats do not freeze more than HC rats prior to presentation of FR-1 trials and subsequent FR-1 escape latencies are equivalent between groups (Maier, Citation1990). An observer, blind to treatment, scored behavior as either freezing or not freezing every 10 sec for a 20 min period after these 2 footshock trials. Freezing was defined as the absence of all body and vibrissae movement beyond that necessary for respiration (Maier, Citation1990).
Tissue collection
Rats received a lethal intraperitoneal injection of sodium pentobarbital (200 mg/kg). Rats were euthanized once completely unresponsive to hindpaw pinch. For immunohistochemistry, rats were perfused with ice-cold saline (0.9%) followed by 4% paraformaldehyde (pH 7.4) and brains rapidly extracted and post-fixed overnight in 4% paraformaldehyde. Brains were transferred to 30% sucrose for 3 days and subsequently flash-frozen in isopentane (−35° C for 80 s). For Western blot and qPCR analysis, brains were rapidly extracted, and flash-frozen in isopentane. Samples were stored at 80 °C until processed.
Corticosterone ELISA
Approximately 24 h after stress, rats were euthanized and trunk blood was centrifuged (14,000 x g for 10 min at 4 °C) and serum separated. An enzyme immunoassay for CORT (Assay Designs, Inc., Ann Arbor, MI) was run in triplicate according to the manufacturer’s instructions. For the assay, serum samples were treated with a steroid displacement reagent and diluted 1:40 with assay buffer. The low and high limits of detectability were 0.002 μg/dL and 4.966 μg/dL.
Immunohistochemistry
Brains were sectioned onto Superfrost Plus slides at 18 microns using a cryostat and stored at −20 C. Slides were defrosted and washed 3x in 0.1 M PBS and blocked in 10% NGS for 1 h. Slides were then incubated at room temperature overnight in 0.1 M PBS + 0.3% Triton-X with the following primary antibodies: rabbit anti-c-fos at 1:500 (Santa Cruz; cat No. sc-52, 1:500) and sheep anti-tryptophan hydroxylase (EMD Millipore; cat No. AB1541, 1:200). Slides were washed 3x in 0.1 M PBS and incubated at room temperature in 1 M PBS + Tx with the following secondary antibodies: goat anti-rabbit at 1:500 (Alexafluor 594, A11037) and donkey anti-sheep at 1:500 (Alexafluor 488, A11015.). Slides were washed three times in 0.1 M PBS and cover-slipped with Vectashield Hardset Antifade Mounting Medium and stored at 4 °C in a light-protective box until imaging.
Imaging
Images were acquired at 10x and 20x magnification on a Nikon N-SIM structured illumination super-resolution and A1 laser scanning confocal microscope. Image analysis was performed using Fiji. Cell counts were determined by averaging two slices in the middle (A/P −1.00 mm) and caudal (−0.70 mm) regions of the DRN (coordinates expressed relative to lambda) for each animal.
Quantitative real-time PCR (qPCR)
Brains were mounted on a freezing cryostat and 1 mm3 micropunch was excised from the DRN (−7.5 mm A/P, −0.0 mm M/L, −8.5 D/V relative to bregma; Total RNA was isolated from tissue using a standard method of phenol:chloroform extraction as described in our prior work (Fonken et al., Citation2016). 500 ng of RNA was reversed transcribed to cDNA using Superscript II according to the manufacturer’s instructions. Primers were designed using Genbank at the National Center for Biotechnology Information (NCBI). Primers were designed to span exon-exon boundaries to exclude amplification of genomic DNA (see ). Primer specificity was verified by melt curve analysis. PCR amplification of cDNA was performed using the Quantitect SYBR Green PCR Kit (Qiagen, Valencia CA) with a MyiQ Single-Color Real-Time PCR Detection System (BioRad, Hercules, CA). Gene expression was determined in triplicate and is expressed relative to a housekeeping gene β-actin.
Figure 2. Stress-induced behavioral changes in juvenile social exploration and shock-elicited freezing, but not sucrose preference, are modulated by time of stressor exposure. Rats received IS or no stress (HC) at ZT6 or ZT16 followed by behavioral testing. (A) Sucrose preference testing occurred during the first 4 h of the dark phase on the two days prior to stress (average baseline, BL) and each of four days after stress (B-C) Juvenile social exploration testing occurred 24 h or 36 h following stress. The ZT6-ZT6 group was stressed at ZT6 and tested 24 h later at ZT6; the ZT16-ZT16 group was stressed at ZT16 and tested 24 h later at ZT16; and the ZT6-ZT16 group was stressed at ZT6 and tested 36 h later at ZT16 (D-E) Fear testing as measured by shock elicited freezing in the shuttlebox occurred 48 h after stress (D) Mean FR-1 escape latencies for FR-1 trials (E) Mean intervals freezing, in 2 min blocks, immediately following two FR-1 trials in a shuttlebox. Data are presented as mean ± SEM. *p ≤ .05, **p ≤ .01, ***p ≤ .001, #p ≤ .0001.
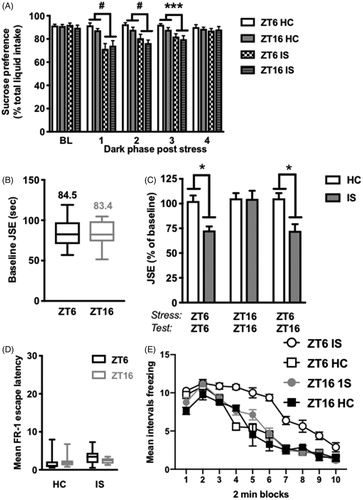
Table 1. PCR primer sequences for 5-HT system genes.
Statistical analyses
All experiments utilized a between-subjects design and the effect of treatment was analyzed with unpaired t-test (time of day), one-way (time of day), two-way (stress x time of day), or 2 x 2 repeated measures ANOVA (stress x time of day x time). Data analyses for all tests were performed using Prism software (Graphpad, La Jolla, CA), except for 2 x 2 repeated-measures ANOVA, which was performed using Statview (SPSS, Chicago, IL). Individual values that were ≥2 standard deviations from the mean were deemed statistical outliers and excluded from analysis. Values in graphs are represented as the group mean ± standard error of the mean.
Results
Experiment 1: stress-induced behavioral alterations are time of day dependent
Here we investigated whether time of stressor exposure differentially modulates the effect of stress on sucrose preference, juvenile social exploration, and fear as assessed by shock-elicited freezing.
Sucrose preference
There was no difference between groups in baseline sucrose preference (stress x ZT at baseline timepoint: p > .05). Stress induced a reduction in sucrose preference on each of the first three dark phases following IS (interaction of time (day) x stress; main effect of stress on day 1: F(1, 32) = 23.23; main effect of stress on day 2: F(1, 31) = 22.13; main effect of stress on day 3: F(1, 31) = 17.12, p < .05 in all cases, ). Sucrose preference returned to baseline by the fourth dark phase following stress (p > .05). The time of day of stressor exposure had no effect on sucrose intake as stress at ZT6 and ZT16 produced equivalent reductions in sucrose preference across each testing period (p > .05). The same pattern of results was observed for absolute sucrose intake (data not shown).
Figure 3. Basal CORT concentrations 24 h after stress during the light or dark phase. Rats received IS or no stress (HC) at ZT6 or ZT16 and were sacrificed 24 h later. (A) Absolute serum CORT concentrations (B) Fold increase in CORT concentrations relative to HC. Data are presented as mean ± SEM. **p ≤ .01, #p ≤ .0001.
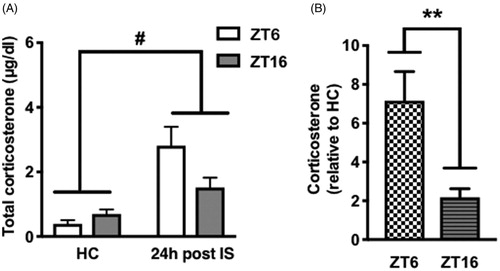
Juvenile social exploration
There was no effect of time of day on baseline social exploration scores, rats tested at ZT6 and ZT16 spent equivalent amounts of time investigating a juvenile conspecific (p > .05, ). Time of day of stressor exposure did, however, differentially influence social exploration 24 h after stress. There was a significant interaction between stress and ZT (F(2, 46) = 4.065, p < .05). As expected, rats stressed at ZT6 and tested 24 h later exhibited the typical stress-induced reduction in social investigation (post hoc, p < .05, ). However, rats stressed at ZT16 and tested 24 h later did not show this reduction. In order to determine whether this effect was due to the ZT of stressor exposure or ZT of behavioral testing, we exposed a separate cohort of rats to stress at ZT6 and tested them 36 h later at ZT16. Importantly, rats stressed at ZT6 and tested 36 h later at ZT16 exhibited the typical stress-induced reduction in social investigation (p < .05), indicating that ZT of stressor exposure, not ZT of behavioral testing, is critical in mediating this particular behavioral effect of IS.
Shock-elicited freezing
Average escape latencies for FR-1 trials in the shuttlebox were equivalent across all groups (stress x ZT at FR-1 trial, p > .05, ) indicating that there was no effect of ZT or prior stress on instrumental responding. Thus, all groups received the same duration footshocks. There was a significant interaction of stress x ZT x trial blocks (time), such that ZT of stressor exposure differentially influenced shock-elicited freezing following FR-1 trials (F(9, 243) = 3.738, p < .05, ). As expected, rats stressed at ZT6 exhibited exaggerated shock-elicited freezing 48 h after stress in the shuttlebox compared to non-stressed controls. Interestingly, rats that were stressed at ZT16 did not show this effect and were indistinguishable from controls. Importantly, there was no difference in freezing between HC rats tested at ZT6 and ZT16 (p > .05) indicating that freezing does not differ by time of day.
Experiment 2: stress-induced increase in basal CORT is not time of day dependent
One possible explanation for the behavioral outcomes produced by stress at ZT6, but not ZT16, is that the stress-induced increase in basal CORT 24 h after IS was different across the two ZTs. This might result in a different CORT signal to stress-responsive brain regions that drive activation of DRN 5-HT neurons at the time of stress or during subsequent behavioral testing. We previously showed that immediately following termination of IS, the magnitude of change in CORT was greater in rats receiving stress at ZT6 compared to ZT16 (Fonken et al., Citation2016). Here we tested whether the stress induced increase in basal CORT concentrations is different 24 h after IS. As expected, there was a significant effect of stress (F(1, 24) = 22.11, p < .0001) on absolute CORT concentrations and an interaction between stress and ZT (F(1, 24) = 5.387, p < .05; ) with a trend toward reduced CORT concentrations in rats that received stress at ZT16 (post hoc analysis did not reveal a statistically significant difference in absolute CORT concentrations between ZT6 and ZT16 groups 24 h after stress; p > .05). Furthermore, the fold increase in CORT was substantially greater during the light as compared to the dark phase (effect of ZT t(12) = 3.185, p < .01; ).
Figure 4. Circadian gene expression of DRN 5-HT factors. (A) Digital template reproduced from the rat brain atlas (Paxinos & Watson, Citation2007) denoting the location of DRN micropunch (circled area). Tissue was collected from stress-naïve rats every 6 h across the light-dark cycle. (B-D) TPH2, SLC6A4, and 5-HT1AR mRNA concentrations are expressed relative to housekeeping gene β-actin and presented as mean ± SEM. *p ≤ .05.
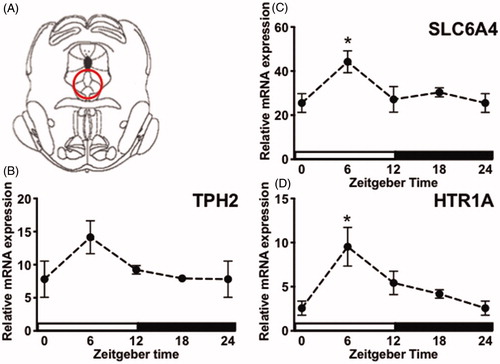
Experiment 3: 5-HT factors are rhythmically expressed in the DRN
Here we tested whether factors that regulate 5-HT are rhythmically expressed in the DRN (coordinates shown in under basal conditions. There was a significant effect of ZT on 5-HT1AR (F(3, 26) = 4.337, p < .05; ) and SLC6A4 (F(3, 27) = 3.478, p < .05; ), but not TPH2 (F(3, 26) = 2.281, p > .05; ) mRNA expression in the DRN, although the trend was for increased TPH2 at ZT6. Post hoc analyses revealed that 5-HT1AR and SLC6A4 mRNA were significantly elevated at ZT6 compared to ZT0. Importantly, others have reported that TPH2 mRNA in the midbrain raphe nuclei peaks at ZT10 (Malek, Dardente, Pevet, & Raison, Citation2005), a ZT that was not included in the present experiment. Thus, some components of 5-HT signaling are rhythmically expressed in the DRN and peak during the middle of the light phase.
Experiment 4: stress-induced DRN 5-HT activation is time of day dependent
Because we saw time of day differences in factors regulating 5-HT in the DRN, we tested whether stress-induced activation of DRN 5-HT neurons was different at the two ZTs. We hypothesized that stress at ZT6 more potently activates DRN 5-HT neurons compared to ZT16. There was a significant interaction between stress and ZT, such that rats that were stressed at ZT6 exhibited a greater percentage of TPH-positive neurons that expressed c-fos in the middle (F(1, 32) = 76.54, p < .0001, ) and caudal (F(1, 32) = 8.175, p = .0007; ) aspects of the DRN compared to rats that were stressed at ZT16. There was no ZT difference in the number of TPH-positive neurons or c-fos expressing nuclei in controls across the two ZTs (p > .05, data not shown). This indicates that stress-induced activation of DRN 5-HT neurons is modulated by time of day.
Figure 5. Stress-induced activation of DRN 5-HT neurons is modulated by time of day. Representative image of stressed-induced activation of DRN 5-HT neurons at ZT6 (A) TPH-immunoreactive (ir) neurons (green) (B) fos-ir nuclei (red) (C) Tph-positive neurons expressing fos in the middle and caudal aspects of the DRN. Data are presented as mean ± SEM. ***p ≤ .001, #p ≤ .0001.
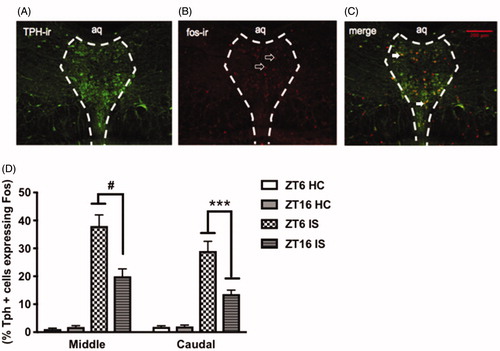
Discussion
The circadian system is highly adaptive as it allows organisms to anticipate recurring changes across the light-dark cycle. Thus, it follows that an organism may be better equipped to cope with a stressor during its active phase when it is more likely to encounter disruptive stimuli. The consequences of IS and most other stressors are primarily characterized in nocturnal (night-active) rodents when stressor exposure occurs during the light phase. Thus, the present study evaluated whether the time of day during which a stressor occurs is a critical determinant of its behavioral and neurochemical consequences. Furthermore, we characterized diurnal differences in DRN 5-HT signaling, which may regulate aspects of the behavioral stress response. Here we showed that rats exposed to stress during the light phase exhibited increased social avoidance, exaggerated fear, and reduced sucrose preference, all consistent with previous work (reviewed in (Maier & Seligman, Citation2016)). Interestingly, rats exposed to stress during the dark phase appeared resistant to behavioral effects that are mediated by stress-induced changes in the 5-HT system (i.e. anxiety and fear).
As expected, rats exposed to stress during the middle of the light phase exhibited an increase in anxiety-like behavior. This was characterized by a marked reduction in juvenile social exploration 24 h after stress (ZT6). In contrast, rats exposed to stress during the dark phase appeared resistant to the stressor. However, it was unclear whether this effect was due to the time of stressor exposure or rather, an artifact produced by time of behavioral testing. In order to tease these possibilities apart, rats were stressed at ZT6 and tested 36 h after stress (ZT16). These rats still exhibited a significant reduction in juvenile social exploration, indicating that the time of stressor exposure is the critical factor producing this particular effect.
Results from a freezing test provided further support for time of day differences in vulnerability to stress. As expected, rats exposed to stress during the middle of the light phase exhibited potentiated fear after 2 footshocks delivered in a shuttlebox. This was characterized by high rates of freezing in response to footshock 48 h after stress (ZT6) compared to non-stressed controls. In contrast, rats stressed during the middle of the dark phase again appeared resistant to stress: rats stressed during the middle of the dark phase did not display exaggerated post-shock fear in the shuttlebox.
These results are in agreement with previous findings demonstrating behavioral responses to both acute and chronic stressors are modulated by time of stress exposure (Aslani et al., Citation2014; Cohen et al., Citation2015; Fonken et al., Citation2016; Koch et al., Citation2016; Retana-Marquez, Citation2003; Rybkin, Citation1997). For example, both chronic mild stress and chronic social defeat stress induced greater behavioral changes when the stressors occurred during the inactive phase (Aslani et al., Citation2014; Koch et al., Citation2016). Furthermore, rats exposed to acute stress (predator scent) at the beginning of the inactive phase (ZT0) exhibited exacerbated behavioral responses (as indicated by performance on the elevated plus maze and acoustic startle paradigm) compared to those exposed to stress at the beginning of the active phase (ZT12) (Cohen et al., Citation2015). NPY regulation of the HPA axis was implicated in the diurnal differences in susceptibility to predator scent stress (Cohen et al., Citation2015).
Stress-induced glucocorticoids have previously been implicated in mediating diurnal differences in response to stress (Cohen et al., Citation2015). For example, juvenile, but not adult rodents stressed during the dark phase exhibited prolonged increases in CORT along with stress-induced behavioral changes (Romeo, Karatsoreos, & McEwen, Citation2006). This led us to evaluate serum CORT concentrations post-stress. There was no significant time of day difference in stress-induced glucocorticoid release (Fonken et al., Citation2016) or in basal glucocorticoids when evaluated 24 h after stress. However, the change in CORT concentrations differed between the light and dark phase, with a greater magnitude of change occurring during the light (inactive) phase (∼7-fold increase) versus the dark phase (∼2-fold increase; refer to ). Further, glucocorticoid receptor number and affinity vary across the light-dark cycle (Dallman, Citation1993; Nader, Chrousos, & Kino, Citation2009) and there are time of day differences in corticosteroid binding globulin (Hsu & Kuhn, Citation1988). Taken together, this suggests that stress-induced changes in glucocorticoid signaling likely differ between the light versus dark phase.
In agreement with our findings, previous reports have shown a greater CORT response to stressors that occur during the inactive phase compared to the active phase (Fonken et al., Citation2016; Retana-Marquez, Citation2003). However, other experiments have reported the inverse pattern (Kalsbeek, Ruiter, la Fleur, Van Heijningen, & Buijs, Citation2003). The Dallman laboratory extensively characterized circadian regulation of the HPA axis, demonstrating diurnal differences in both activation by stress and inhibition by CORT (reviewed in (Dallman, Citation1993)). Interestingly, even within the same paper there were discrepancies in whether stressors induce greater activation of the HPA axis during the light versus the dark phase (Engeland, Shinsako, Winget, Vernikos-Danellis, & Dallman, Citation1977). Two factors appear important for explaining these discrepancies: (1) differences in stressor severity/intensity (Engeland et al., Citation1977; Spencer & Deak, Citation2017) and, (2) the type of stressor (physical versus psychological) (Kalsbeek et al., Citation2003).
Not all consequences of IS are driven by DRN 5-HT hyperactivity. For example, while previous work indicates that the DRN 5-HT hyperactivity causes reduced juvenile social exploration and increased post-shock freezing, behavioral anhedonia does not appear dependent on the DRN (Christianson et al., Citation2008; Maier et al., Citation1995). Thus, we next wanted to establish whether time of day differences in the stress response generalized to behavioral changes that are not dependent on the DRN. Interestingly, IS-induced reductions in sucrose preference were equivalent regardless of time of stressor exposure. Pharmacological inhibition of DRN 5-HT neurons during stress prevents the IS-induced reduction in juvenile social exploration (Christianson et al., Citation2008) and potentiated fear (Maier et al., Citation1995), but not reduced sucrose preference (Christianson et al., Citation2008). Therefore, the DRN is likely not involved in stress-induced reductions in sucrose preference. The DRN sends a robust 5-HTergic projection to the amygdala, a key structure mediating fear and anxiety (reviewed in (Bocchio, McHugh, Bannerman, Sharp, & Capogna, Citation2016)). Additionally, IS produces a large efflux of 5-HT in the amygdala both at the time of IS and during subsequent behavioral testing (Christianson et al., Citation2008). Furthermore, amygdalar lesion prevents IS-induced potentiated fear in the shuttlebox (Maier et al., Citation1993) and intra-amygdala antagonism of 5-HT2C receptors prevents IS-induced social avoidance (Christianson et al., Citation2010). These findings indicate that IS-induced sensitization of an amygdala projecting DRN circuit likely drives stress-induced alterations in anxiety and fear. Taken together, these results suggest that DRN-dependent behavioral outcomes may be particularly susceptible to the time at which a stressor occurs.
This led us to suspect that there might be time of day differences in IS-induced activation of DRN 5-HT neurons. Here we found that IS during the light phase produced a much greater percentage of tryptophan hydroxylase-positive neurons in the mid and caudal DRN that co-express c-fos, suggesting that the DRN 5-HT response to stress is circadian gated. The 5-HT system is under circadian control and as a result 5-HT content exhibits 24 h fluctuations in the DRN and afferent projection regions (Barassin et al., Citation2002; Cagampang & Inouye, Citation1994). Our results do not establish how time of day modulates IS-induced activation of DRN 5-HT neurons. However, previous work indicates that DRN 5-HT activity is regulated by light: the DRN receives direct input from melanopsin-expressing intrinsically photosensitive retinal ganglion cells (ipRGCs (Shen & Semba, Citation1994)). Additionally, the DRN receives indirect circadian input from the SCN via the dorsomedial hypothalamus and subparaventricular zone (Deurveilher & Semba, Citation2005).
DRN 5-HT activity is also regulated by autoinhibitory 5-HT1ARs (Sprouse & Aghajanian, Citation1987). Exposure to IS during the light phase transiently desensitizes 5-HT1ARs rendering them incapable of producing negative feedback for at least 72 h (Rozeske et al., Citation2011). As a result, DRN 5-HT neurons become sensitized such that exposure to subsequent stimuli elicits a robust 5-HT response. Time of day differences in IS-induced activation of DRN 5-HT neurons thus might be driven by diurnal variation in 5-HT1AR availability. In other words, DRN 5-HT neurons may be more susceptible to stress-induced sensitization at ZT6. To begin to test this idea, we examined whether DRN 5-HT1AR mRNA expression varies across the light-dark cycle. Contrary to our hypothesis, we found that expression of 5-HT1AR was greatest at ZT6, suggesting that DRN autoinhibition might be greater during the light phase. However, it is important to note that mRNA expression does not always correlate with functional protein (Greenbaum, Colangelo, Williams, & Gerstein, Citation2003). Another consideration here is that we did not distinguish between cell types, as some non-5-HT neurons in the DRN also express 5-HT1ARs, although these neurons are largely confined to the rostral DRN (Day et al., Citation2004).
Another potential mechanism for the production of diurnal differences in IS-induced neurochemical effects is that DRN 5-HT neurons are more potently activated by afferent projections from stress-responsive brain regions during the light phase. During IS, convergent excitation from multiple arousal systems activates DRN 5-HT neurons. One of these inputs is a glutamatergic projection from the lateral habenula (Kalen, Karlson, & Wiklund, Citation1985). Indeed, activation of the lateral habenula is both necessary and sufficient for producing the behavioral outcomes that follow IS during the light phase (Dolzani et al., Citation2016). In mice, the margin of the LbH receives input from melanopsin-expressing ipRGCs, suggesting that it is light responsive (Hattar, Citation2002). Indeed, LbH neurons exhibit higher baseline firing rates during the light phase compared to the dark phase in vitro (Zhao & Rusak, Citation2005). Additionally, restraint stress activates the LbH in a time of day dependent manner (Chastrette, Pfaff, & Gibbs, Citation1991). Other known inputs to the DRN may also be under circadian control. For example, extracellular norepinephrine in the locus coeruleus also exhibits diurnal variation that co-varies with DRN 5-HT (Agren, Koulu, Saavedra, Potter, & Linnoila, Citation1986).
Although not addressed in this study, previous work indicates that there may be sex differences in circadian regulation of stress responses (Huynh, Krigbaum, Hanna, & Conrad, Citation2011). There are sex differences in both the neuroinflammatory (Fonken et al., Citation2016) and behavioral responses to IS (Baratta et al., Citation2019), suggesting that sex is an important variable to consider in future work.
The present study provides clear support that the diurnal phase during which a potent stressor occurs can potentially influence the outcome of that event. Thus, temporal factors may explain, in part, why individuals who encounter seemingly identical stressors have widely disparate responses to the stressor. These results also indicate that individuals who are more likely to encounter a traumatic event during their inactive phase (e.g. shift workers) may be at a greater risk to develop anxiety disorders and depression. Future stress research should consider time of day as an important experimental variable.
Notes on contributors
L.K.F., R.A.D., and S.F.M designed experiments; R.A.D, L.K.F. and J.R.R. performed experiments; R.A.D. analyzed the data; R.A.D, J.R.R., L.R.W, S.F.M. and L.K.F. contribute to writing and editing the paper.
Disclosure statement
No potential conflict of interest was reported by the authors.
Additional information
Funding
References
- Agren, H., Koulu, M., Saavedra, J.M., Potter, W.Z., & Linnoila, M. (1986). Circadian covariation of norepinephrine and serotonin in the locus coeruleus and dorsal raphe nucleus in the rat. Brain Research, 397, 353–358. doi:10.1016/0006-8993(86)90638-4
- Albrecht, U. (2012). Timing to perfection: the biology of central and peripheral circadian clocks. Neuron, 74, 246–260. doi:10.1016/j.neuron.2012.04.006
- Amat, J., Matus-Amat, P., Watkins, L.R., & Maier, S.F. (1998). Escapable and inescapable stress differentially alter extracellular levels of 5-HT in the basolateral amygdala of the rat. Brain Research, 812, 113–120. doi:10.1016/S0006-8993(98)00960-3
- Aslani, S., Harb, M.R., Costa, P.S., Almeida, O.F.X., Sousa, N., & Palha, J.A. (2014). Day and night: diurnal phase influences the response to chronic mild stress. Frontiers in Behavioral Neuroscience, 8, 82. doi:10.3389/fnbeh.2014.00082
- Barassin, S., Raison, S., Saboureau, M., Bienvenu, C., Maître, M., Malan, A., & Pévet, P. (2002). Circadian tryptophan hydroxylase levels and serotonin release in the suprachiasmatic nucleus of the rat. European Journal of Neuroscience, 15, 833–840. doi:10.1046/j.1460-9568.2002.01928.x
- Baratta, M.V., Gruene, T.M., Dolzani, S.D., Chun, L.E., Maier, S.F., & Shansky, R.M. (2019). Controllable stress elicits circuit-specific patterns of prefrontal plasticity in males, but not females. Brain Structure and Function, 224, 1831–1843. doi:10.1007/s00429-019-01875-z
- Bocchio, M., McHugh, S.B., Bannerman, D.M., Sharp, T., & Capogna, M. (2016). Serotonin, amygdala and fear: Assembling the puzzle. Frontiers in Neural Circuits, 10, 24. doi:10.3389/fncir.2016.00024
- Boldrini, M., Underwood, M.D., Mann, J.J., & Arango, V. (2005). More tryptophan hydroxylase in the brainstem dorsal raphe nucleus in depressed suicides. Brain Research, 1041, 19–28. doi:10.1016/j.brainres.2005.01.083
- Cagampang, F.R., & Inouye, S.T. (1994). Diurnal and circadian changes of serotonin in the suprachiasmatic nuclei: regulation by light and an endogenous pacemaker. Brain Research, 639, 175–179. doi:10.1016/0006-8993(94)91780-9
- Chastrette, N., Pfaff, D.W., & Gibbs, R.B. (1991). Effects of daytime and nighttime stress on Fos-like immunoreactivity in the paraventricular nucleus of the hypothalamus, the habenula, and the posterior paraventricular nucleus of the thalamus. Brain Research, 563, 339–344. doi:10.1016/0006-8993(91)91559-J
- Christianson, J.P., Paul, E.D., Irani, M., Thompson, B.M., Kubala, K.H., Yirmiya, R., … Maier, S.F. (2008). The role of prior stressor controllability and the dorsal raphe nucleus in sucrose preference and social exploration. Behavioural Brain Research, 193, 87–93. doi:10.1016/j.bbr.2008.04.024
- Christianson, J.P., Ragole, T., Amat, J., Greenwood, B.N., Strong, P.V., Paul, E.D., … Maier, S.F. (2010). 5-hydroxytryptamine 2C receptors in the basolateral amygdala are involved in the expression of anxiety after uncontrollable traumatic stress. Biological Psychiatry, 67, 339–345. doi:10.1016/j.biopsych.2009.09.011
- Cohen, S., Vainer, E., Matar, M.A., Kozlovsky, N., Kaplan, Z., Zohar, J., … Cohen, H. (2015). Diurnal fluctuations in HPA and neuropeptide Y-ergic systems underlie differences in vulnerability to traumatic stress responses at different Zeitgeber Times. Neuropsychopharmacology, 40, 774–790. doi:10.1038/npp.2014.257
- Dallman, M.F. (1993). Stress update Adaptation of the hypothalamic-pituitary-adrenal axis to chronic stress. Trends in Endocrinology & Metabolism, 4, 62–69. doi:10.1016/S1043-2760(05)80017-7
- Daut, R.A., & Fonken, L.K. (2019). Circadian regulation of depression: A role for serotonin. Frontiers in Neuroendocrinology, 54, 100746. doi:10.1016/j.yfrne.2019.04.003
- Day, H.E.W., Greenwood, B.N., Hammack, S.E., Watkins, L.R., Fleshner, M., Maier, S.F., & Campeau, S. (2004). Differential expression of 5HT-1A, α1b Adrenergic, CRF-R1, and CRF-R2 receptor mRNA in serotonergic, γ-aminobutyric acidergic, and catecholaminergic cells of the rat dorsal raphe nucleus. The Journal of Comparative Neurology, 474, 364–378. doi:10.1002/cne.20138
- Deurveilher, S., & Semba, K. (2005). Indirect projections from the suprachiasmatic nucleus to major arousal-promoting cell groups in rat: Implications for the circadian control of behavioural state. Neuroscience, 130, 165–183. doi:10.1016/j.neuroscience.2004.08.030
- Dolzani, S.D., Baratta, M.V., Amat, J., Agster, K.L., Saddoris, M.P., Watkins, L.R., & Maier, S.F. (2016). Activation of a habenulo-raphe circuit is critical for the behavioral and neurochemical consequences of uncontrollable stress in the male rat. ENEURO, 3, ENEURO.0229-16.2016. doi:10.1523/ENEURO.0229-16.2016
- Donner, N.C., Kubala, K.H., Hassell Jr, J.E., Lieb, M.W., Nguyen, K.T., Heinze, J.D., … Lowry, C.A. (2018). Two models of inescapable stress increase tph2 mRNA expression in the anxiety-related dorsomedial part of the dorsal raphe nucleus. Neurobiology of Stress, 8, 68–81. doi:10.1016/j.ynstr.2018.01.003
- Engeland, W.C., Shinsako, J., Winget, C.M., Vernikos-Danellis, J., & Dallman, M.F. (1977). Circadian patterns of stress-induced ACTH secretion are modified by corticosterone responses. Endocrinology, 100, 138–147. doi:10.1210/endo-100-1-138
- Fanselow, M.S., Lester, L.S., & Helmstetter, F.J. (1988). Changes in feeding and foraging patterns as an antipredator defensive strategy - a laboratory simulation using aversive-stimulation in a closed economy. Journal of the Experimental Analysis of Behavior, 50, 361–374. doi:10.1901/jeab.1988.50-361
- Fleshner, M., Deak, T., Spencer, R.L., Laudenslager, M.L., Watkins, L.R., & Maier, S.F. (1995). A long-term increase in basal levels of corticosterone and a decrease in corticosteroid-binding globulin after acute stressor exposure. Endocrinology, 136, 5336–5342. doi:10.1210/endo.136.12.7588279
- Fonken, L.K., Weber, M.D., Daut, R.A., Kitt, M.M., Frank, M.G., Watkins, L.R., & Maier, S.F. (2016). Stress-induced neuroinflammatory priming is time of day dependent. Psychoneuroendocrinology, 66, 82–90. doi:10.1016/j.psyneuen.2016.01.006
- Grahn, R.E., Will, M.J., Hammack, S.E., Maswood, S., McQueen, M.B., Watkins, L.R., & Maier, S.F. (1999). Activation of serotonin-immunoreactive cells in the dorsal raphe nucleus in rats exposed to an uncontrollable stressor. Brain Research, 826, 35–43. doi:10.1016/S0006-8993(99)01208-1
- Greenbaum, D., Colangelo, C., Williams, K., & Gerstein, M. (2003). Comparing protein abundance and mRNA expression levels on a genomic scale. Genome Biology, 4, 117. doi:10.1186/gb-2003-4-9-117
- Hale, M.W., Shekhar, A., & Lowry, C.A. (2012). Stress-related serotonergic systems: implications for symptomatology of anxiety and affective disorders. Cellular and Molecular Neurobiology, 32, 695–708. doi:10.1007/s10571-012-9827-1
- Harfstrand, A., Fuxe, K., Cintra, A., Agnati, L.F., Zini, I., Wikstrom, A.C., … Steinbusch, H. (1986). Glucocorticoid receptor immunoreactivity in monoaminergic neurons of rat brain. Proceedings of the National Academy of Sciences, 83, 9779–9783. doi:10.1073/pnas.83.24.9779
- Hattar, S. (2002). Melanopsin-containing retinal ganglion cells: architecture, projections, and intrinsic photosensitivity. Science, 295, 1065–1070. doi:10.1126/science.1069609
- Horschitz, S., Hummerich, R., & Schloss, P. (2001). Down-regulation of the rat serotonin transporter upon exposure to a selective serotonin reuptake inhibitor. Neuroreport, 12, 2181–2184. doi:10.1097/00001756-200107200-00027
- Hsu, B.R., & Kuhn, R.W. (1988). The role of the adrenal in generating the diurnal variation in circulating levels of corticosteroid-binding globulin in the rat. Endocrinology, 122, 421–426. doi:10.1210/endo-122-2-421
- Huynh, T.N., Krigbaum, A.M., Hanna, J.J., & Conrad, C.D. (2011). Sex differences and phase of light cycle modify chronic stress effects on anxiety and depressive-like behavior. Behavioural Brain Research, 222, 212–222. doi:10.1016/j.bbr.2011.03.038
- Janusonis, S., & Fite, K.V. (2001). Diurnal variation of c-Fos expression in subdivisions of the dorsal raphe nucleus of the Mongolian gerbil (Meriones unguiculatus). The Journal of Comparative Neurology, 440, 31–42. doi:10.1002/cne.1368
- Kalen, P., Karlson, M., & Wiklund, L. (1985). Possible excitatory amino acid afferents to nucleus raphe dorsalis of the rat investigated with retrograde wheat germ agglutinin and D-[3H]aspartate tracing. Brain Research, 360, 285–297. doi:10.1016/0006-8993(85)91244-2
- Kalsbeek, A., Ruiter, M., la Fleur, S.E., Van Heijningen, C., & Buijs, R.M. (2003). The diurnal modulation of hormonal responses in the rat varies with different stimuli. Journal of Neuroendocrinology, 15, 1144–1155. doi:10.1111/j.1365-2826.2003.01112.x
- Kessler, R.C., Berglund, P., Demler, O., Jin, R., Merikangas, K.R., & Walters, E.E. (2005). Lifetime prevalence and age-of-onset distributions of DSM-IV disorders in the National Comorbidity Survey Replication. Archives of General Psychiatry, 62, 593–602. doi:10.1001/archpsyc.62.6.593
- Koch, C.E., Bartlang, M.S., Kiehn, J.T., Lucke, L., Naujokat, N., Helfrich-Förster, C., … Oster, H. (2016). Time-of-day-dependent adaptation of the HPA axis to predictable social defeat stress. Journal of Endocrinology, 231, 209–221. doi:10.1530/JOE-16-0163
- Maier, S.F. (1990). Diazepam modulation of stress-induced analgesia depends on the type of analgesia. Behavioral Neuroscience, 104, 339–347. doi:10.1037/0735-7044.104.2.339
- Maier, S.F., & Seligman, M.E. (2016). Learned helplessness at fifty: Insights from neuroscience. Psychological Review, 123, 349–367. doi:10.1037/rev0000033
- Maier, S.F., Grahn, R.E., & Watkins, L.R. (1995). 8-OH-DPAT microinjected in the region of the dorsal raphe nucleus blocks and reverses the enhancement of fear conditioning and interference with escape produced by exposure to inescapable shock. Behavioral Neuroscience, 109, 404–412. doi:10.1037/0735-7044.109.3.404
- Maier, S.F., Grahn, R.E., Kalman, B.A., Sutton, L.C., Wiertelak, E.P., & Watkins, L.R. (1993). The role of the amygdala and dorsal raphe nucleus in mediating the behavioral consequences of inescapable shock. Behavioral Neuroscience, 107, 377–388. doi:10.1037/0735-7044.107.2.377
- Malek, Z.S., Dardente, H., Pevet, P., & Raison, S. (2005). Tissue-specific expression of tryptophan hydroxylase mRNAs in the rat midbrain: anatomical evidence and daily profiles. European Journal of Neuroscience, 22, 895–901. doi:10.1111/j.1460-9568.2005.04264.x
- Malek, Z.S., Sage, D., Pévet, P., & Raison, S. (2007). Daily rhythm of tryptophan hydroxylase-2 messenger ribonucleic acid within raphe neurons is induced by corticoid daily surge and modulated by enhanced locomotor activity. Endocrinology, 148, 5165–5172. doi:10.1210/en.2007-0526
- Maswood, S., Barter, J.E., Watkins, L.R., & Maier, S.F. (1998). Exposure to inescapable but not escapable shock increases extracellular levels of 5-HT in the dorsal raphe nucleus of the rat. Brain Research, 783, 115–120. doi:10.1016/S0006-8993(97)01313-9
- Matthews, K., Forbes, N., & Reid, I.C. (1995). Sucrose consumption as an hedonic measure following chronic unpredictable mild stress. Physiology & Behavior, 57, 241–248. doi:10.1016/0031-9384(94)00286-E
- Nader, N., Chrousos, G.P., & Kino, T. (2009). Circadian rhythm transcription factor CLOCK regulates the transcriptional activity of the glucocorticoid receptor by acetylating its hinge region lysine cluster: potential physiological implications. The Faseb Journal, 23, 1572–1583. doi:10.1096/fj.08-117697
- Paxinos, G., & Watson, C. The Rat Brain in Stereotaxic Coordinates. 2007, London: Academic Press.
- Retana-Marquez, S. (2003). Body weight gain and diurnal differences of corticosterone changes in response to acute and chronic stress in rats. Psychoneuroendocrinology, 28, 207–227. doi:10.1016/S0306-4530(02)00017-3
- Romeo, R.D., Karatsoreos, I.N., & McEwen, B.S. (2006). Pubertal maturation and time of day differentially affect behavioral and neuroendocrine responses following an acute stressor. Hormones and Behavior, 50, 463–468. doi:10.1016/j.yhbeh.2006.06.002
- Rozeske, R.R., Evans, A.K., Frank, M.G., Watkins, L.R., Lowry, C.A., & Maier, S.F. (2011). Uncontrollable, but not controllable, stress desensitizes 5-HT1A receptors in the dorsal raphe nucleus. Journal of Neuroscience, 31, 14107–14115. doi:10.1523/JNEUROSCI.3095-11.2011
- Rybkin, I.I. (1997). Effect of restraint stress on food intake and body weight is determined by time of day. American Journal of Physiology, 273, R1612–22. doi:10.1152/ajpregu.1997.273.5.R1612
- Shen, H., & Semba, K. (1994). A direct retinal projection to the dorsal raphe nucleus in the rat. Brain Research, 635, 159–168. doi:10.1016/0006-8993(94)91435-4
- Spencer, R.L., & Deak, T. (2017). A users guide to HPA axis research. Physiology & Behavior, 178, 43–65. doi:10.1016/j.physbeh.2016.11.014
- Sprouse, J.S., & Aghajanian, G.K. (1987). Electrophysiological responses of serotoninergic dorsal raphe neurons to 5-HT1A and 5-HT1B agonists. Synapse, 1, 3–9. doi:10.1002/syn.890010103
- Stamford, J.A., Davidson, C., McLaughlin, D.P., & Hopwood, S.E. (2000). Control of dorsal raphe 5-HT function by multiple 5-HT(1) autoreceptors: parallel purposes or pointless plurality?. Trends in Neurosciences, 23, 459–465. doi:10.1016/S0166-2236(00)01631-3
- Walther, D.J. (2003). Synthesis of serotonin by a second tryptophan hydroxylase isoform. Science, 299, 76–76. doi:10.1126/science.1078197
- Zhao, H., & Rusak, B. (2005). Circadian firing-rate rhythms and light responses of rat habenular nucleus neurons in vivo and in vitro. Neuroscience, 132, 519–528. doi:10.1016/j.neuroscience.2005.01.012