Abstract
Uncontrollable stress precipitates negative mental and physical health outcomes. Furthermore, the vicarious experience of stress (e.g. observing another individual experience a direct stressor) can mimic the effects of directly experiencing the stressor. The current experiment examined the behavioral and physiological effects of the vicarious experience of stress using the socially monogamous prairie vole. Male prairie voles were exposed to either an empty open field chamber, or a chamber in which the animal observed a sibling undergoing a concurrent direct physical stressor (tail suspension test) for five minutes. Exploratory and anxiety-like behaviors were recorded in all observers during the test session. Cardiac indices of heart rate and heart rate variability were recorded in a subset of observers prior to, during, and following the test session. Corticosterone levels were measured in all observers and siblings following the test session. When compared to animals exposed to an empty open field chamber, animals that observed a sibling undergo a direct physical stressor exhibited increased heart rate and circulating corticosterone, and decreased heart rate variability. These physiological stress indicators were supported by behavioral changes, including increased freezing followed immediately by orienting of the head toward the center of the apparatus, and decreased locomotion, grooming, and rearing. These preliminary results suggest that prairie voles experience stress vicariously, and provide a foundation for additional studies focused on the underlying mechanisms of vicarious stress. The use of this model may inform our understanding of the social transmission of stress among social species, including humans.
The experience of stress, including observing stress in a loved one, has negative consequences on mental and physical health. This study used a social rodent (prairie voles) to demonstrate that stress transfers among social individuals, consequently producing an increased physiological and behavioral stress response in prairie voles observing their siblings experience stress. This research informs our understanding of the interactions of social experiences and stress in humans.
LAY SUMMARY
Introduction
Negative consequences of uncontrollable and unpredictable stress are pervasive. For instance, Americans report high levels of daily stress, with 20% experiencing extreme stress (American Psychological Association, Citation2017). Many chronic stressors are social in nature, such as interpersonal conflict and/or negative social interactions (American Psychological Association, Citation2017). Social stressors influence depression, anxiety, inflammatory disorders, heart diseases, and premature mortality (Cacioppo & Cacioppo, Citation2018; Glaser & Kiecolt-Glaser, Citation2005).
Unfortunately, the stress experience may not be confined to the individual directly exposed to the stressor. When a member of a social group sees, hears, or has knowledge of another’s stress, the observer may vicariously experience a stress response. For instance, 40% of humans who observe a romantic partner exposed to a psychosocial stressor display physiologically significant increases in cortisol (Engert et al., Citation2014). Additionally, the anterior cingulate cortex (associated with affective aspects of pain) is activated when an individual experiences social exclusion and when observing a friend experience exclusion (Eisenberger et al., Citation2003; Meyer et al., Citation2013). Further, witnessing a traumatic event is currently included as a diagnostic criterion for post-traumatic stress disorder in the Diagnostic and Statistical Manual of Mental Disorders (DSM-5), highlighting the significance of vicarious stress for mental health (American Psychiatric Association, Citation2013; Engert et al., Citation2019).
Vicarious stress also has been characterized in animal models. Mice that observe other mice exposed to biting flies or physical defeat from another animal display increased corticosterone, relative to non-exposed controls (Bailey et al., Citation2004; Kavaliers et al., Citation2003). In addition, rats that witness another rat exposed to social defeat display nearly identical increases in heart rate (HR) and blood pressure to rats that are directly exposed to the defeat (Finnell et al., Citation2017). Related consequences of vicarious stress in rodents include depression- and anxiety-like behaviors, altered social behaviors, and sympathetic hyperactivity (Bailey et al., Citation2004; Burkett et al., Citation2016; Carnevali et al., Citation2017; Finnell et al., Citation2017; Iñiguez et al., Citation2018; Sial et al., Citation2016).
The prairie vole may be an ideal translational model for investigating vicarious stress, as this rodent species demonstrates social monogamy similar to humans, including living in family groups and forming long-term social bonds (Carter & Getz, Citation1993; Young et al., Citation2011). Social stressors produce depression- and anxiety-relevant behaviors, poor stress-coping, and cardiovascular and endocrine disruptions in prairie voles; and this species also responds to the social buffering of stress (Bosch et al., Citation2009; Burkett et al., Citation2016; Grippo et al., Citation2007b; McNeal et al., Citation2014; Smith & Wang, Citation2014; Sun et al., Citation2014).
The current study describes a new paradigm to investigate the potential experience of vicarious stress in prairie voles. This paradigm was developed to test the hypothesis that stress is socially transmitted between two prairie voles, and that observing another prairie vole experience stress will lead to an increased stress response in the observer. To accomplish this goal, sibling pairs of prairie voles were exposed to a social experience that involved the maximum transmission of visual, olfactory, and auditory cues (but prevented direct physical contact between the animals). One prairie vole of the pair was placed in an open field (OF), while either simultaneously observing its sibling exposed to a direct stressor in the form of a tail suspension test (TST) in the same apparatus, or conversely while its sibling was exposed to the TST in an identical separate apparatus. This design allowed for the investigation of specific behavioral and physiological responses in the animals using previously-validated OF and TST paradigms (Bosch et al., Citation2009; Cryan et al., Citation2005; McNeal et al., Citation2017; Olazábal & Young, Citation2005; Seibenhener & Wooten, Citation2015). Further, this design provided an opportunity to conduct primary analyses of behavioral and physiological responses of the sibling pairs in each condition (tested concurrently vs. tested separately), as well as exploratory relationships between specific variables and responses of each animal in the pair. We predicted that prairie voles that directly observed a sibling experience the TST would display increased behavioral and physiological indicators of stress in the OF, relative to animals that experienced the OF alone.
Methods
Animals
Fifty-two pairs of adult (60–90 days of age), reproductively naïve, male prairie voles (35–45 g), were used for the experimental procedures, for a total of 104 experimental animals. Animals were descendants of a wild stock originally captured near Champaign, IL, USA. Animals were maintained on a 14/10 h light/dark cycle (lights on at 6:30am), with a mean ± standard error of the mean (SEM) temperature of 25 ± 1 °C and a relative humidity of 24 ± 1 g/m3. All animals were allowed ad libitum access to food (Purina rabbit chow) and water. Offspring were housed with the family group in large polycarbonate cages (25 × 45 × 60 cm) with food, water, bedding, and cotton nesting material until 21 days of age. At this time, animals were weaned and housed in same-sex sibling pairs in smaller polycarbonate cages (12 × 18 × 28 cm) with food, water, bedding, and cotton nesting material, through the end of the experiment. All procedures were conducted in accordance with the National Institutes of Health Guide for the Care and Use of Laboratory Animals and were approved by the Northern Illinois University Institutional Care and Use Committee.
Experimental design, timeline, and sample sizes
The experimental design and timeline are discussed here (also see ), with all methodological details described in the following sections. All procedures described in the following sections were conducted during the light period, approximately 3–5 h following light onset. The current study utilized a combination of the TST and OF test, to provide a direct physical stressor to one animal (TST), and to simultaneously allow for measurement of exploratory and anxiety-like behavior in an observing animal (OF), while providing maximum auditory, olfactory, and visual transmission between the two animals. Sibling pairs of prairie voles were assigned to one of the following two conditions: concurrent testing condition, which involved one animal being exposed to the direct physical stressor in the TST, while its sibling was exposed to the OF in the same apparatus at the same time, for five minutes (n = 26 sibling pairs); or separate testing condition, which involved one animal exposed to the TST, while its sibling was exposed to the OF, each alone in separate apparatuses in different rooms, but at the same time, for five minutes (n = 26 sibling pairs).
In all animals that experienced the OF (n = 26 concurrent; n = 26 separate), behaviors during the five minute OF were recorded and coded for location within the apparatus, general locomotion, grooming, rearing, and escape attempts. In all animals that experienced the TST (n = 26 concurrent; n = 26 separate), behaviors during the five minute TST were recorded and coded for active movements vs. passive immobility.
In addition to the behavioral dependent measures, which were conducted in all animals, the pairs in each testing condition were further subdivided into either an endocrine subgroup (n = 14 concurrent and n = 14 separate animals that experienced the OF; and n = 14 concurrent and n = 14 separate siblings that experienced the TST) or a cardiac subgroup (n = 12 concurrent and n = 12 separate animals that experienced the OF). Prairie vole sibling pairs in the endocrine subgroup were removed from the testing apparatus, and each sibling of the pair was placed individually into a clean cage for a 10 min undisturbed period. Subsequently, blood was collected for the analysis of circulating corticosterone concentrations. An additional 10 age-matched, pair-housed, undisturbed male animals also had blood collected at approximately the same time of day as the experimental animals, to serve as unmanipulated control subjects for the corticosterone analyses.
Prairie voles in the cardiac subgroup (only animals that experienced the OF) were implanted with a wireless radiotelemetry transmitter 2–3 weeks prior to the testing session. The radiotelemetry device transmitted electrocardiogram (ECG), activity, and body temperature data, which were recorded prior to, during, and following the testing session for the analysis of cardiac reactivity and recovery following the testing session. Prairie vole sibling pairs in the cardiac subgroup were removed from the testing apparatus, and replaced in their home cages (in sibling pairs) for a 12 h undisturbed period. During this 12 h period, radiotelemetry data were recorded for the analysis of physiological and behavioral recovery following the testing session.
Behavioral testing apparatus
A custom-designed testing chamber was used to test pairs of prairie voles in either the concurrent or separate testing groups (). The testing apparatus consisted of a white-bottomed, square box (40 × 40 cm), with a 20 × 20 cm internal section delineated by a red outline on the floor of the apparatus. The OF testing arena was surrounded with transparent Plexiglas sides (40 cm), high enough to prevent escape from subjects placed in the apparatus. This OF section of the apparatus was used to investigate exploratory and other behaviors exhibited by one animal of the sibling pair. Horizontally placed across the top of the box (in the middle) was a copper tube that was used to attach the tail of the second animal of the sibling pair, which hung approximately 25 cm above the apparatus floor. A digital video camera was mounted with an over-head view of the behaviors exhibited within the testing apparatus, so that the behavior of both subjects could be recorded.
Figure 2. Black-and-white image of the testing chamber, with a view directly from above, showing two animals in the chamber (one in the TST and one in the OF). Identical chambers were used for the concurrent and separate testing conditions. In the concurrent group, both the TST model and OF observer were placed in the same chamber at the same time (as shown here); in the separate testing group, the TST animal and OF animal were each tested individually, in two separate, identical chambers in different locations (at the same time).
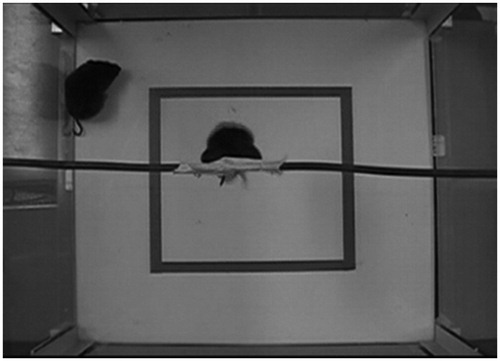
Pairs of animals in the concurrent testing condition were placed into the combined chamber at the same time, such that the animal in the OF chamber had visual, auditory, and olfactory cues from its respective sibling in the TST, but the animals were unable to touch each other. Pairs of animals in the separate testing condition were placed into two different chambers at the same time, located in separate rooms to prevent the transmission of visual, auditory, and olfactory cues between the two animals.
Regardless of concurrent or separate testing conditions, the placement of pairs of prairie voles into the testing chamber was standardized, such that the tail of the animal exposed to the TST was affixed to the copper tube at approximately the same time that the animal exposed to the OF was placed into the arena. The two animals were therefore released from the experimenters’ hands at the same time at the beginning of the test. The removal of pairs of prairie voles from the testing chamber was also standardized, such that the two animals were removed at approximately the same time at the end of the five minute test.
Procedures and specific behavioral measurements in the tail suspension test
One animal from each sibling pair was placed into the TST. The procedure was adapted from previously published methods involving measures of stress-coping behaviors and efficacy of pharmacological antidepressant treatments in rodents, including in prairie voles (Bosch et al., Citation2009; Cryan et al., Citation2005; Grippo et al., Citation2018; McNeal et al., Citation2017). Each animal (regardless of concurrent or separate testing group) was suspended by its tail using adhesive tape to the copper tube and hung in the middle of the testing chamber for five minutes. Care was taken to ensure that the tail was appropriately affixed to the tube without compromising the tail itself during attachment, the five minute test, or detachment, by attaching the tape to the middle of the tail (equidistant between the tip and base of the tail; the tail is approximately 3 cm in length). Following the testing session, the tape was removed, and the tube was cleaned and dried.
Each five minute trial was digitally video recorded and later scored via the Observer XT 8.0 (Noldus; Wageningen, Netherlands), by trained observers. Trials were scored for the following mutually-exclusive, exhaustive categories: (a) duration of active coping behaviors: categorized as active movements via either contortions of the body and/or flailing of the limbs; versus (b) duration of immobility: categorized as no movement of the body or limbs besides those required for respiration; this category also included swinging as a result of momentum from previous active movements (Cryan et al., Citation2005).
Procedures and specific behavioral measurements in the open field
The second animal from each sibling pair was placed into the OF chamber. The procedure was adapted from previously published protocols (Olazábal & Young, Citation2005), and has been used to characterize exploratory and anxiety-relevant behaviors in rodents, including prairie voles (Grippo et al., Citation2014). Each animal (regardless of concurrent or separate testing group) was placed on the floor of the testing chamber for five minutes, at the same time that its sibling was suspended from the TST tube. The arena was cleaned and dried following each test.
All five minute trials were digitally video recorded and later scored via the Observer XT 8.0, by trained observers. Behaviors were defined as the following: (a) instances of freezing: the animal was coded as freezing when the animal was visibly frozen or paused, with the paws visibly splayed; (b) instances of orienting the head toward center of apparatus associated with freezing: this category was coded any time the animal oriented its head toward the center apparatus immediately after an instance of freezing; (c) instances of orienting the head toward center of apparatus unrelated to freezing: this category was coded any time the animal oriented its head toward the center of the apparatus unrelated to a prior freezing episode; (d) instances of escape attempts: the animal was coded as attempting to escape when it engaged in behaviors such as scratching at the walls, corners, or floor of the apparatus where the floor meets the wall, attempting to chew on, scratch at, or pull on the metal bars that hold the walls of the apparatus in place, or jumping at the wall or corner of the apparatus; (e) instances of rearing: a rear was categorized as any time the two front paws left the ground and were subsequently replaced on the ground, either in the air, at the wall, or at the corner of the apparatus; (f) duration of time spent in the center vs. surrounding section (mutually-exclusive, exhaustive categories): the animal was coded as entering a section of the arena when all 4 paws crossed the red line that delineated the respective section; (g) duration of time spent grooming: the animal was coded as grooming when it used its front paws to clean its fur or rub its face and/or body, used its hind paws to scratch itself, or twisted its head and torso around to reach and groom/scratch at its back and hind body (the animal may move around in a tight circle during grooming); and (h) duration of time spent in exploratory motion: the animal was coded as engaging in exploratory motion when all four paws changed physical location, characterized by either forward or backward progress (upward movement using only two paws was excluded – as in the case of a rear; and moving in a tight circle without a physical change in location was excluded – as in the case of grooming).
Radiotelemetry measurements
Approximately 2–3 weeks prior to the testing session, wireless radiofrequency transmitters (TA10ETA-F10; Data Sciences International, St. Paul, Minnesota) were surgically implanted intraperitoneally (ip) into the cardiac subgroup of animals (exposed to the OF). Transmitters were implanted using aseptic techniques, under isoflurane anesthesia mixed with oxygen, during the light period, for continuous recording of ECG, activity, and body temperature variables. Transmitter implantation was conducted as described previously (Grippo et al., Citation2007a). Briefly, after the animal displayed signs of deep anesthesia, the body of the transmitter was implanted into the abdominal cavity, and the positive and negative leads were tunneled subcutaneously and sutured to the muscle on either side of the heart using DII positioning. All incisions were sutured closed with sterile suture. An injection of carprofen (5 mg/kg, ip) was administered following the procedure.
Following immediate recovery from anesthesia, animals were housed for 5 days in custom-designed divided cages as described previously (Grippo et al., Citation2007a), with ad libitum access to 2% sucrose (in addition to ad libitum food and water). This housing condition allowed the siblings visual, olfactory, auditory, and limited tactile interactions through small holes in a clear cage divider, while permitting adequate healing of suture wounds in the instrumented animal. A heat lamp was positioned to cover approximately 1/3 of the cage housing the instrumented animal for the first 2 nights following surgery, allowing the animal to self-regulate body temperature. Following 5 days of recovery in the divided cages, all animals were returned to the standard home cages (with their respective siblings) to recover for an additional 7–10 days. Throughout the recovery period, the animals were observed for the following characteristics of appropriate surgical recovery: (a) visible signs of eating and drinking; (b) adequate urination and defecation; (c) adequate activity level (several periods throughout the light and dark periods of approximately two counts per minute [CPM] or higher); (d) adequate body temperature (approximately 37.oC); and (e) stabilization of the HR signal.
ECG signals were recorded with a radiotelemetry receiver and software provided by the vendor (sampling rate 5 kHz, 12-bit precision digitizing; Dataquest ART Acquisition, Data Sciences International). Activity level was monitored via the receiver (sampling rate 256 Hz). Body temperature was monitored via the receiver (based on calibration specifications for each transmitter from the vendor). Data segments were recorded at hourly intervals during an undisturbed baseline period, which occurred in the home cages of the animals on the morning of the test session (2–5 min of data recorded each hour). Data were recorded continuously during the five minute testing session. Following the test session, both animals of the sibling pair were removed from the apparatus at the same time (regardless of condition), and replaced in the home cage in sibling pairs. Data segments were recorded for an undisturbed 12 h period following the testing session (2–5 min of data recorded each hour), while the animals were in the home cage.
Stable, continuous data from the vendor software (Dataquest ART Analysis, Data Sciences International) were used to evaluate ECG, activity level, and body temperature variables during each phase of the experiment, including the baseline period, the 5 min test session, and at 3, 6, and 12 h during the recovery period. A gross measure of activity was generated using the vendor software based on transmitter signal strength as the animal moved within the OF apparatus or the home cage, which was quantified as CPM. This measure represents a coarse index of physical activity intensity, via low, medium, or high levels of activity (note that this measure does not correlate in a linear manner with the above-referenced manual category of exploratory motion duration during the OF session, as it does not differentiate between horizontal and vertical movements). Body temperature was reported in Celsius. HR was quantified using the number of beats per minute (BPM), from the ECG signal recorded using the vendor software. HR variability was quantified using time series methods. The R-R intervals were analyzed for variations using the standard deviation of all normal-to-normal (N-N) intervals (SDNN index), and root mean square of successive differences between normal heartbeats (RMSSD), generated from the ECG signal recorded using the vendor software. These indices represent the convergence of sympathetic and parasympathetic inputs on the cardiac pacemaker and parasympathetically-mediated cardiac changes, respectively, as measures of neural control of autonomic function (Shaffer & Ginsberg, Citation2017). The measures of HR, SDNN index, and RMSSD were specifically investigated here based on previous evidence that both short- and long-term environmental changes and stressors result in alterations of these variables (Grippo et al., Citation2007b; McNeal et al., Citation2014; McNeal et al., Citation2019; Shaffer & Ginsberg, Citation2017).
Corticosterone measurements
Animals in the endocrine subgroup were evaluated for circulating corticosterone levels. Following the five minute test session, both animals of the sibling pair were removed from the apparatus at the same time (regardless of condition), and placed individually into clean cages for an undisturbed period of 10 min. This time period was specifically chosen based on previous evidence demonstrating that corticosterone levels may be elevated approximately 10–15 min following the end of a short-term environmental experience in prairie voles (McNeal et al., Citation2017). At the end of this 10-min period, animals were anesthetized with a combination of ketamine and xylazine (ketamine, 67 mg/kg sc, NLS Animal Health, Owings Mills, MD; xylazine, 13.33 mg/kg sc, NLS Animal Health). Blood was collected from all sibling pairs (whether in the concurrent or separate testing group) at the same time in separate locations, without visual, auditory, or olfactory cues. To provide a comparison of undisturbed, basal corticosterone levels, blood was also collected from a group of unmanipulated animals (n = 10), which had no prior manipulations, and were picked up directly from the home cage. These unmanipulated control subjects were similar in reproductive status, age, and body weight to the experimental animals; and the time of day of blood collection was matched to animals from the experimental groups.
Each prairie vole (regardless of concurrent, separate, or unmanipulated control group) was transported into the sample collection room and anesthetized within one minute of being transported. Using procedures validated previously in prairie voles (McNeal et al., Citation2017), blood was sampled beginning two minutes following the anesthetic injection, to avoid possible differences in anesthesia influence on hormone levels. Blood was collected from the periorbital sinus, via a heparinized capillary tube, during a period of 1.5 min (resulting in approximately 0.5–1 ml of blood from each animal), to avoid possible rapid fluctuations in hormone levels. This time frame resulted in blood being collected from animals in the separate and concurrent groups at 13.5 min following the end of the test session, and at 3.5 min following the anesthetic injection. Blood was placed immediately on ice, centrifuged at 4 °C, at 1100 g, for 15 min to obtain plasma. Plasma aliquots were stored at −80 °C until assayed for circulating corticosterone.
Plasma concentrations of corticosterone were measured using a commercial enzyme-linked immunosorbent assay kit, according to the kit instructions (ADI-900-097, Enzo Life Sciences, Farmingdale, NY), which has been previously validated and published by our laboratory for use in prairie voles (McNeal et al., Citation2017). Plasma was diluted in assay buffer as necessary (1:500), based on preliminary validation experiments (McNeal et al., Citation2014, Citation2017), to yield results reliably within the linear portion of the standard curve. The minimum detection limit was 0.027 ng/ml, and inter- and intra-assay coefficients of variation are < 5%. Cross-reactivity with other steroids or peptides is < 1.7%.
Data analyses
Primary hypothesis-driven comparisons
For all hypothesis-driven comparisons, repeated variables were analyzed with mixed design analyses of variance (ANOVA), with group (concurrent or separate) as the between-group factor and time as the within-group factor. Single independent means were compared using single-factor ANOVAs and/or either independent-group or repeated-measures Student’s t-tests. A Bonferroni correction was applied to all multiple comparisons. The present data did not violate the assumptions of homogeneity of variance or independence using Levene’s tests, with one exception (number of rears in the OF; t-tests assuming unequal variances were used for these comparisons, as noted).
Data were analyzed using SPSS for Windows, version 24 (IBM, Armonk, NY), and StatPlus for Mac, version v6 (AnalystSoft Inc., Walnut, CA). All data are presented as means and SEM. A probability value of p < .05 was considered statistically significant using 2-tailed tests for overall significance testing. For t-tests involving multiple comparisons, a result was considered to be statistically significant when it was below the adjusted probability value.
For all behavioral analyses in the TST and OF, digital video files were scored off-line by two or three coders, who were blind to the hypotheses of the experiment. For each behavioral category, raters were required to reach at least 90% inter-rater reliability, using a training strategy described previously (McNeal et al., Citation2019). Briefly, if the agreement between two trained raters did not initially reach 90% inter-rater reliability for a specific behavioral category, a third rater scored the entire five minute file, followed by discussions among all raters to determine whether there was a systematic reason why the initial raters did not agree, and/or to devise a strategy to improve agreement among the raters (for example, one or both initial raters would re-score the entire five minute file after a discussion of the interpretation of a particular behavioral category). For all behavioral categories, the scores between two raters (or among three raters, as relevant) were averaged.
Three pairs of animals were excluded from relevant analyses (two in the concurrent group and one in the separate group) due to problems during the TST, including either the animal’s tail slipping out of the tape and the animal subsequently falling to the floor of the apparatus (in which case the test was aborted immediately), or the animal gaining access to the bar (such that the animal was able to hold onto the bar itself with its paws and/or was able to chew on the tape). These exclusions resulted in final analyzed sample sizes for each measure as follows: (a) behaviors, n = 25 pairs (both OF and TST) in the separate condition and n = 24 pairs (both OF and TST) in the concurrent condition; (b) cardiac function, activity, and body temperature, n = 12 animals in the separate condition (OF only) and n = 10 animals in the concurrent condition (OF only); and (c) endocrine activity, n = 13 pairs in the separate condition (both OF and TST) and n = 14 pairs in the concurrent condition (both OF and TST), which were compared to the unmanipulated control group (n = 10).
Exploratory comparisons
To gain a more detailed understanding of how the dependent variables were inter-related in the observer animals (in the OF), as well as how the behavior of the animal in the TST was associated with the behavior or physiological reactivity of the animal in the OF, exploratory comparisons were conducted using Pearson’s r correlation coefficient. Active movements of the animal in the TST (generated from manual scoring of active behaviors during that test) were compared with the following variables, in the separate and concurrent groups separately: HR in the animal experiencing the OF (BPM); and activity level in the animal experiencing the OF (CPM, generated from the radiotelemetry transmitter). In the animals that experienced the OF, HR (BPM) also was compared with instances of freezing (#) in the separate and concurrent groups separately. Statistical significance is not reported for correlations given the small sample sizes; these comparisons were conducted for exploratory purposes only. The same pairs of animals that experienced TST problems (noted above) were excluded from these comparisons.
Results
Behavioral responses
Behavioral responses in animals that experienced the OF are displayed in (instances of behaviors) and (durations of behaviors). Animals in the concurrent group exhibited significantly greater instances of freezing, t(46) = 5.27, p < .0001, and post-freezing orienting toward the center of the apparatus, t(46) = 5.38, p < .0001, during the testing session, relative to the separate group. However, animals in the concurrent group exhibited significantly fewer instances of orienting toward the center unrelated to freezing, relative to the separate group, t(46) = 3.31, p < .002. Animals in the concurrent group also displayed fewer instances of rearing during the testing session, relative to the separate group, t(30) = 2.31, p < .02, t-test assuming unequal variances; however this reduction was driven specifically by a reduction in rearing in the surrounding section of the OF arena, 29.9 ± 3.5 rears and 19.3 ± 1.0 rears in separate and concurrent groups, respectively; t(30) = 2.89, p < .007; t-test assuming unequal variances. The number of rears in the central section of the OF arena was increased in the concurrent testing group, relative to the separate group, 0.4 ± 0.1 rears and 2.2 ± 0.4 rears in the separate and concurrent groups, respectively; t(30) = 4.19, p < .001; t-test assuming unequal variances]. Further, a significant reduction in the duration of time spent in exploratory motion, t(46) = 1.99, p < .05, and duration of grooming, t(46) = 2.19, p < .03, was observed in the concurrent group, relative to the separate group.
Figure 3. Mean (+SEM) instances of behaviors (Panel A), including freezing, post-freezing orienting toward the center of the apparatus, non-freezing-associated orienting toward the center of the apparatus, and rearing; and durations of behaviors (Panel B), including the duration of exploratory motion and grooming, exhibited during the five minute OF test session in prairie voles tested either separately from a sibling (separate; n = 25, all analyzed subjects), or concurrently with a sibling in the TST in the same apparatus (concurrent; n = 24, all analyzed subjects). *p <.05 vs. separate group.
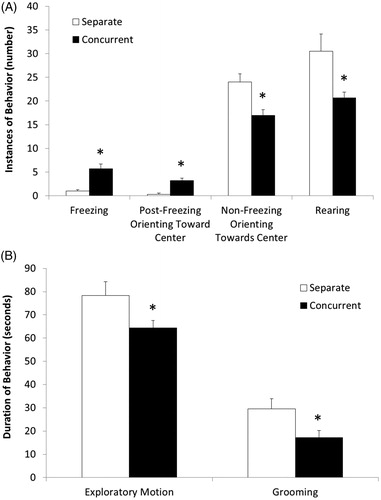
No group differences were observed in the number of escape attempts or duration of time spent exploring the center vs. surround section of the apparatus (p > .05 for all comparisons; data not shown).
In animals that experienced the TST, no group differences in duration of immobility were observed during the five minute test session (immobility duration in the separate group: 122.7 ± 11.5 s; immobility duration in the concurrent group: 136.4 ± 16.3s; p > .05).
Cardiac and physical activity responses
A mixed-design ANOVA conducted on HR comparing the separate and concurrent groups during the baseline (pre-test) period, five minute OF session, and 3, 6, and 12 h following the OF session yielded a main effect of time, F(4,119) = 42.81, p < .001, and a group by time interaction, F(4,119) = 2.50, p < .04 (). Student’s t-tests (with a Bonferroni correction) demonstrated that both groups displayed higher HR values vs. their respective baseline values, separate: t(11) = 5.53, p < .0002; concurrent: t(9) = 8.11, p < .001, and HR was significantly higher in the concurrent group during the OF session vs. the separate group, t(20) = 2.07, p < .02. HR was elevated in both groups, relative to their respective baseline values, at three hours following the OF, separate: t(11) = 3.73, p < .003; concurrent: t(9) = 2.90, p < .01. Both groups displayed HR values that were comparable to their respective baseline values by six hours following the OF, which was maintained at 12 h following the OF (p > .05 for all comparisons).
Figure 4. Mean (+SEM) HR (Panel A), physical activity level (Panel B), SDNN index (Panel C), and RMSSD (Panel D) at baseline (pre-test), during the five minute OF session, and at 3, 6, and 12 h following the OF session, in prairie voles tested either separately from a sibling (separate; n = 12 analyzed subjects in the OF, cardiac subgroup only), or concurrently with a sibling in the TST in the same apparatus (concurrent; n = 10 analyzed subjects in the OF, cardiac subgroup only). *p < .05 vs. separate group at the same time point; #p < .05 vs. respective baseline value in both the separate and concurrent groups; $p < .05 vs. respective baseline value in the concurrent group only.
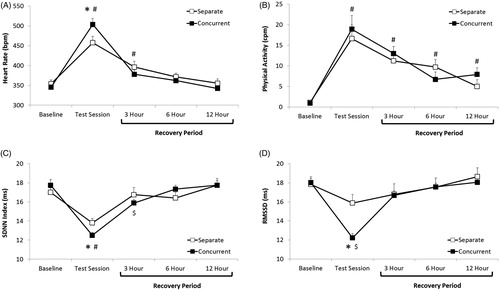
A mixed-design ANOVA conducted on general activity level (derived from the radiotelemetry transmitter) comparing the separate and concurrent groups during the baseline (pre-test) period, five minute OF session, and 3, 6, and 12 h following the testing session yielded a main effect of time, F(4,119) = 21.88, p < .001 (). Given the lack of a group effect or interaction, the separate and concurrent groups were pooled for the purposes of pairwise comparisons across the time points (with a Bonferroni correction). Activity level was significantly higher than baseline values for both groups (pooled) at all time points, during the OF session: t(20) = 8.03, p < .001; three hours following the OF: t(20) = 8.78, p < .001; six hours following the OF: t(20) = 5.25, p < .001; 12 h following the OF: t(20) = 5.65, p < .001.
A mixed-design ANOVA conducted on SDNN index comparing the separate and concurrent groups during the baseline (pre-test) period, five minute OF session, and 3, 6, and 12 h following the testing session yielded a main effect of time, F(4,119) = 16.65, p < .001, and a group by time interaction, F(4,119) = 3.86, p < .006 (). Student’s t-tests (with a Bonferroni correction) demonstrated that both groups displayed lower SDNN index values relative to their respective baseline values, separate: t(11) = 4.68, p < .001; concurrent: t(9) = 8.73, p < .001, and the SDNN index was lower in the concurrent group versus the separate group during the OF session, t(20) = 2.36, p < .02. The SDNN index in the separate group was comparable to this group’s respective baseline value by three hours following the OF session, which was maintained at both 6 and 12 h following the OF session (p > .05 for all comparisons). The SDNN index in the concurrent group remained lower than its respective baseline value three hours following the test session, t(9) = 2.74, p < .02, but was comparable to its respective baseline value by six hours following the OF session, and was maintained at 12 h following the OF (p > .05 for both comparisons).
A mixed-design ANOVA, conducted on RMSSD, comparing the separate and concurrent groups during the baseline (pre-test) period, five minute OF session, and 3, 6, and 12 h following the testing session yielded a main effect of time, F(4,119) = 11.15, p < .0001, and a group by time interaction, F(4,119) = 2.43, p < .05 (). Student’s t-tests (with a Bonferroni correction) demonstrated that RMSSD during the OF session was lower in the concurrent group versus its respective baseline RMSSD value, t(9) = 6.93, p < .0001, and versus the separate group, t(20) = 3.62, p < .002. RMSSD in the separate group during the OF session did not differ significantly from this group’s respective baseline value (p > .05 with Bonferroni correction). The RMSSD values in both groups were comparable to their respective baseline values by three hours following the OF session. These values were maintained at both 6 and 12 h following the OF session (p > .05 for all comparisons).
Body temperature responses
A mixed-design ANOVA conducted on body temperature (derived from the radiotelemetry transmitter) comparing the separate and concurrent groups during the baseline (pre-test) period, five minute OF session, and 3, 6, and 12 h following the testing session did not yield significant main effects of group or time, or a group by time interaction (p > .05 for all comparisons; data not shown; no follow-up tests were conducted).
Endocrine responses
In animals that experienced the OF, a single-factor ANOVA (with a Bonferroni correction) comparing the separate, concurrent, and unmanipulated control groups yielded a main effect of group, F(2,35) = 51.43, p < .001 (). Student’s t-tests (with a Bonferroni correction) demonstrated that the concurrent group displayed higher levels of corticosterone relative to the separate group, t(25) = 4.45, p < .001; and that both the separate, t(22) = 5.69, p < .001, and concurrent groups, t(23) = 11.17, p < .001, displayed higher levels of corticosterone versus the unmanipulated control group.
Figure 5. Mean (+SEM) circulating corticosterone levels 10 min following the end of the OF or TST in prairie voles tested either separately from a sibling (separate; n = 13 analyzed subjects in the OF, n = 13 analyzed subjects in the TST, endocrine subgroup only), or concurrently with a sibling in the same apparatus (concurrent; n = 14 analyzed subjects in the OF, n = 14 analyzed subjects in the TST, endocrine subgroup only), relative to an unmanipulated control group (n = 10). *p ≤ .05 for specific pairwise comparison; ^p <.05 vs. all experimental groups.
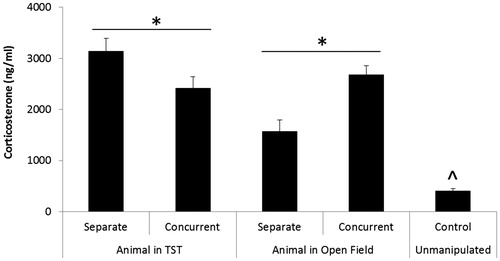
In animals that experienced the TST, a single-factor ANOVA (with a Bonferroni correction) comparing the separate, concurrent, and unmanipulated control groups yielded a main effect of group, F(2,31) = 48.73, p < .001 (). Student’s t-tests (with a Bonferroni correction) demonstrated that the separate group displayed slightly higher levels of corticosterone relative to the concurrent group, t(25) = 2.17, p = 0.05; but that both the separate, t(22) = 10.44, p < .001, and concurrent groups, t(23) = 8.39, p < .001, displayed higher levels of corticosterone versus the unmanipulated control group.
Exploratory analyses
Exploratory correlational analyses conducted in animals with radiotelemetry transmitters indicated that some variables appeared to be systematically associated in the concurrent group, with the same associations not observed in the separate group (). In the concurrent group only, the level of active movements in the animal experiencing the TST was positively correlated with both general activity level (r = .43) and HR (r = .33) of the animal in the OF. By contrast, no systematic correlation was observed between either of these measures in the separate group (r = −.21 and r = .00 for general activity level and HR, respectively). Further, in the concurrent group only, freezing instances during the OF were positively correlated with HR (r = .58); by contrast, no correlation between these variables was observed in the separate group (r = .00).
Figure 6. Scatterplots (and best fitting lines) from exploratory comparisons, showing associations between active movements of the model animal in the TST vs. HR in the observer in the OF (Panels A and B); active movements of the model animal in the TST vs. general activity of the observer in the OF (Panels C and D); and instances of freezing vs. HR of the observer in the OF (Panels E and F). Scatterplots for the concurrent group are shown on the left side (Panels A, C, and E); and scatterplots for the separate group are shown on the right side (Panels B, D, and F).
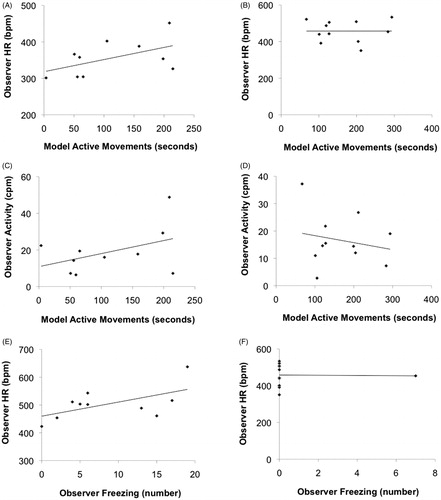
Discussion
The current study investigated whether the presence of a directly stressed sibling would elicit physiological indices of stress and associated behavioral changes in prairie vole observers. To accomplish this goal, a combination of the TST and OF was utilized to promote the induction of direct stress in one animal, while also simultaneously allowing for the measurement of behavioral and physiological reactivity in the observer animal. The results provide preliminary evidence that observing a sibling experience a direct physical stressor increases cardiac and endocrine indicators of stress, coupled with behavioral changes, in an observer animal. These findings may shed light on the transmission of stress among social species.
The physiological responses of observers in the present study support the hypothesis that observing a sibling experience a direct physical stressor increases stress reactivity in the observer. For instance, observers in the concurrent condition exhibited significantly elevated HR and decreased HR variability during the OF session (vs. separate condition). These alterations, which were not the result of increased behavioral activation or differences in physical activity in the concurrent group, suggest greater cardiac reactivity and altered neural control of autonomic and cardiac responses, including both increased sympathetic drive and reduced parasympathetic drive (Shaffer & Ginsberg, Citation2017; Thayer & Lane, Citation2009). Further, the autonomic measures support previous evidence that the OF itself is stressful (Pattij et al., Citation2002), as prairie voles in both the separate and concurrent conditions exhibited an increase in HR and a reduction in SDNN index during the five minute test session, relative to undisturbed home cage baseline measures. However, RMSSD – which specifically reflects parasympathetic regulation of the heart via vagal nerve influence (Shaffer & Ginsberg, Citation2017; Thayer & Lane, Citation2009) – was significantly reduced only in the concurrent condition (vs. respective baseline measures). This dissociation between SDNN index and RMSSD may reflect differential neural control of autonomic reactivity as a function of vicarious stress, such that the experience of observing a sibling experience a direct stressor specifically influences the parasympathetic limb of the autonomic nervous system. By contrast, experiencing the OF alone in the present design does not specifically reduce parasympathetic regulation of the heart.
In addition to altered cardiac and autonomic reactivity characterized by increased HR and reduced HRV, observers in the concurrent condition also displayed significantly increased corticosterone following the OF session, suggesting increased endocrine responsiveness to the separate condition (Carnevali et al., Citation2017; Finnell et al., Citation2017; McEwen, Citation2008). These alterations support studies indicating that witnessing another animal experience an aversive stimulus alters endocrine and cardiovascular parameters representative of increased stress in the observer, including elevated blood pressure, HR, and circulating corticosterone (Burkett et al., Citation2016; Carnevali et al., Citation2017; Finnell et al., Citation2017; Langford et al., Citation2006).
Augmenting the physiological data in the present study, the behavioral results further support the hypothesis that animals in the concurrent condition experienced an altered stress response relative to those in the separate condition. Animals in the concurrent condition displayed decreased exploratory movements around the testing apparatus, coupled with increased freezing and specific orientation of the head toward the area of the apparatus that contained the sibling in the TST, immediately following an episode of freezing. Locomotion and freezing are discussed in other rodent models as indices of exploratory and anxiety-like behavior (Carola et al., Citation2002; Gould et al., Citation2009; Seibenhener & Wooten, Citation2015; Walsh & Cummins, Citation1976). Reduced locomotion and increased freezing behavior may indicate a high-stress state in prairie voles observing a sibling exposed to the direct TST stressor. Similarly, in rats that witnessed a cagemate exposed to footshocks, those witnesses that had prior experience with receiving a footshock displayed an increase in vicarious freezing behavior, relative to naïve witnesses that had no prior experience with footshocks (Atsak et al., Citation2011).
Animals in the concurrent condition also exhibited decreased rearing and grooming relative to animals in the separate condition. Previous interpretations of these behaviors have differed, depending on the paradigms and models used. For example, increased grooming may reflect an anxiety-like behavior in female prairie voles following immobilization stress, as an increase in grooming behavior was observed following exposure to immobilization stress in females that recovered alone after immobilization, relative to females that recovered in the presence of a male partner (Smith & Wang, Citation2014). Conversely, when grooming is considered in a novel environment, this behavior is positively correlated with the amount of time spent in the novel environment (Choleris et al., Citation2001), and is observed when rodents establish a home base in a new environment (Wallace et al., Citation2008). Similarly, rearing behavior may represent an animal’s attempt to explore a novel environment, and therefore both increased and decreased rearing behaviors have been discussed in the context of stress and anxiety (Borta & Schwarting, Citation2005; Seibenhener & Wooten, Citation2015). Rearing exposes the animal to danger, such that it may represent a reduction in anxiety-like behavior, as the animal perceives the environment as safe enough to complete the behavior (Weisstaub et al., Citation2006). Considering these interpretations together with the other behavioral and physiological outcomes in the present study, the decreased rearing and grooming behaviors in the concurrent condition suggest that these observers experienced a heightened state of stress, vigilance, and anxiety-like behavior, with comparatively less time directed toward self-care or self-soothing behavior. Consistent with this interpretation, studies from rats and mice indicate that witnessing other animals experience aversive stimuli can vicariously produce a fear response in the observers (Meyza et al., Citation2017).
Previous hypotheses may explain the mechanisms underlying vicarious stress in humans, including psychological processes such as empathy or emotional contagion. Empathy may support basic processes that are beneficial to the survival of humans, such as social facilitation of alarm, mother-infant responsiveness, feeding, mating, and emotional reactivity (Levenson, Citation2003; Preston & De Waal, Citation2002). Empathetic processes facilitate rapid information flow throughout a social group, via individuals recognizing and matching their emotional states to other group members. Activating representations of another individual’s emotional state may automatically generate the appropriate autonomic and somatic responses, unless inhibited within the observer (Preston & De Waal, Citation2002). A sub-category of empathy that may be related to vicarious stress is emotional contagion – automatically converging emotionally with another individual’s experience, such as through facial expressions, vocalizations, or movements (Hatfield et al., Citation1992). Emotional contagion may serve the purpose of alerting, calming, and promoting empathy within social groups (Levenson, Citation2003). Processes that may mediate emotional contagion have been described using non-human animal models (Atsak et al., Citation2011; Sivaselvachandran et al., Citation2018), further supporting the hypothesis that animal models are useful tools for investigating the social transmission of behavioral and physiological processes. The exploratory correlational analyses in the present study may lend support to hypotheses of emotional contagion. In the concurrent condition, both the physical activity level and HR of the observer were positively correlated with the respective sibling’s level of active movements in the TST. By contrast, this pattern was not observed in animals in the separate condition (as expected, given that an animal in this condition had no visual, auditory, or olfactory cues from its respective sibling in the TST).
Because the design used here involves dynamic interactions between two animals, the results from the present study may likewise contribute to our understanding of social buffering against increased stress. When an individual experiences a stressor, the presence of a comparatively less-stressed individual may decrease the stress response (Hennessy et al., Citation2009; Hostinar et al., Citation2014; Smith & Wang, Citation2014). Predictions based on this social buffering hypothesis are supported by the current corticosterone results, given that animals undergoing the TST in the concurrent condition (with a sibling present) displayed slightly lower corticosterone than those in the separate condition. It may be possible that the mechanisms responsible for vicarious stress and social buffering are related. A feedback system may exist between dyads in a stressful experience in which there is an inequality of stress (i.e. one member is more stressed than the other). Over time, as the members of the dyad influence one another, the stress levels of both individuals may reach equilibrium, with the levels higher than the initial level of the less-stressed individual but lower than the initial state of the more-stressed individual. As this possible mechanism may be of interest in therapeutic settings (or in other social relationships), it is an important topic for future research.
This study provides preliminary evidence that the current experimental paradigm can be used to investigate vicarious stress in a socially monogamous rodent model. However, some elements inherent to the study design may limit certain conclusions. This paradigm was designed to necessarily include a dynamic social situation between two familiar animals, to gain insight into the specific social transmission of information about the state of stress in each animal. Therefore, the state of one animal may lead to upregulation or downregulation (or some combination of both) of stress in the other animal, which may in turn dynamically influence additional behavioral states of each specific animal. For instance, contrary to previous studies focused on stress, anxiety, and exploration in the OF, a common measure of anxiety-like behavior – a reduction in the amount of time spent in the center section of the arena (Olazábal & Young, Citation2005; Seibenhener & Wooten, Citation2015) – did not differ between animals in the concurrent and separate conditions. However, the driving factor that influenced exploration of the center of the apparatus may have differed as a function of the condition. Specifically, the attention of the observer in the OF may have been drawn to its sibling in the TST – which is supported by increased orientation toward the center following an episode of freezing, as well as increased rearing in the center of the apparatus – such that the drive to avoid the center was overpowered by the drive to investigate the sibling. By contrast, animals in the separate condition had no specific stimulus to draw their attention or behavior toward the center of the arena – consistent with the observation that this group exhibited almost no freezing or associated post-freezing orientation toward the center. Therefore, the decision to enter the center of the apparatus in this group may have been a function of general exploration of a novel environment. Future studies will benefit from considering additional designs or modifications of the apparatus, for instance by placing a TST bar over an observer’s familiar home cage – which may avoid the aforementioned competing demands in the concurrent condition.
Related to the dynamic social situation, the present apparatus was specifically designed to maximize all possible sensory cues (except tactile) between two animals in the concurrent condition. However, in so doing, the design also produced the inexorable consequence of additional communication transmitted between the animals in the concurrent condition, relative to the separate condition. It is possible that some aspects of altered behavior and physiology of an animal in the concurrent condition were a function of general disruptions produced by its sibling (i.e. sound, movement), rather than interpretation of a specific state of stress in the sibling. However, this alternate explanation is neither parsimonious nor supported by the present behavioral and physiological differences between the concurrent and separate groups. First, rodents use several auditory, olfactory, pheromonal, neural, and behavioral communication strategies, therefore it is likely to be specifically via this socially-transmitted information that the state of stress in another rodent is interpreted by an observer [see for instance (Sterley et al., Citation2018)]. The differential correlations between the behavioral responses of the animal in the TST and its respective sibling observer in the concurrent vs. separate groups support this interpretation. Second, given the bi-directional social transmission of information inherent in the present design, if general movement or noise were responsible for the changes, higher corticosterone levels in the animal exposed to the TST would be expected in the concurrent condition, vs. the separate condition. Conversely, the animals experiencing the TST in the concurrent condition displayed lower corticosterone levels than those in the separate condition, suggesting that the stress state of the animal experiencing the TST may have been matched to or down-regulated by its sibling being present in the OF apparatus in the concurrent group. The comprehensive set of responses in both siblings, from each condition, therefore suggests that the stress state of each animal was a function of its knowledge about the state of stress experienced by its familiar sibling. To confirm this hypothesis, future studies should compare the behavioral and physiological responses of animals in the concurrent condition with additional control conditions, such as the presence of an unfamiliar animal, an anesthetized (familiar or unfamiliar) animal, an inanimate object, and various sensory signals that represent another animal (e.g. auditory or olfactory cues), but without an animal present. Additionally, the investigation of sex differences would be prudent, given that males and females may differ in their experiences of social stress.
The present study demonstrates that observing a sibling experience a direct physical stressor leads to a heightened physiological stress response and associated behavioral changes that may reflect an anxiety-like state in prairie voles. These results provide a framework for future studies to investigate the underlying mechanisms responsible for vicarious stress among social species, as well as the interactions between vicarious stress and social experiences (such as social buffering of stress responses). A better understanding of these mechanisms will contribute valuable knowledge about the experience of vicarious stress in humans.
Acknowledgements
The authors would like to thank Blessy Johnson, Ashley Dagner, William Colburn, Elliott Ihm, and Matthew Woodbury for valuable assistance.
Disclosure statement
The authors declare no conflicts of interest.
Additional information
Funding
Notes on contributors
Joshua Wardwell
Joshua Wardwell received a PhD in psychology from Northern Illinois University, and currently works in education and therapy settings, and as a freelance scientific editor.
W. Tang Watanasriyakul
W. Tang Watanasriyakul is a current PhD student in psychology at Northern Illinois University.
Marigny C. Normann
Marigny Normann is a current MA student in psychology at Northern Illinois University.
Oreoluwa I. Akinbo
Oreoluwa Akinbo is a current MA student in psychology at Northern Illinois University.
Neal McNeal
Neal McNeal received a PhD in psychology from Northern Illinois University, and currently is a Lieutenant in the United States Navy.
Sarah Ciosek
Sarah Ciosek has a BA from Northern Illinois University.
Miranda Cox
Miranda Cox has a BS from Northern Illinois University.
Nicole Holzapfel
Nicole Holzapfel has a BS from Northern Illinois University.
Samantha Sujet
Samantha Sujet has a BS from Northern Illinois University.
Angela J. Grippo
Angela J. Grippo is an Associated Professor in the Department of Psychology at Northern Illinois University.
References
- American Psychiatric Association. (2013). Diagnostic and statistical manual of mental disorders (5th ed.). Arlington, VA: American Psychiatric Association.
- American Psychological Association. (2017). Stress in America: The state of our nation. Retrieved from www.stressinamerica.org
- Atsak, P., Orre, M., Bakker, P., Cerliani, L., Roozendaal, B., Gazzola, V., … Keysers, C. (2011). Experience modulates vicarious freezing in rats: A model for empathy. PLoS One, 6, e21885. doi:10.1371/journal.pone.0021855
- Bailey, M.T., Avitsur, R., Engler, H., Padgett, D.A., & Sheridan, J.F. (2004). Physical defeat reduces the sensitivity of murine splenocytes to the suppressive effects of corticosterone. Brain, Behavior and Immunity, 18, 416–424. doi:10.1016/j.bbi.2003.09.012
- Borta, A., & Schwarting, R. (2005). Inhibitory avoidance, pain reactivity, and plus-maze behavior in Wistar rats with high versus low rearing activity. Physiology & Behavior, 84, 387–396. doi:10.1016/j.physbeh.2005.01.009
- Bosch, O.J., Nair, H.P., Ahern, T.H., Neumann, I.D., & Young, L.J. (2009). The CRF system mediates increased passive stress-coping behavior following the loss of a bonded partner in a monogamous rodent. Neuropsychopharmacology, 34, 1406–1415. doi:10.1038/npp.2008.154
- Burkett, J.P., Andari, E., Johnson, Z.V., Curry, D.C., de Waal, F.B.M., & Young, L.J. (2016). Oxytocin-dependent consolation behavior in rodents. Science, 351, 375–378. doi:10.1126/science.aac4785
- Cacioppo, J.T., & Cacioppo, S. (2018). Loneliness in the modern age: An evolutionary theory of loneliness (ETL). Advances in Experimental Social Psychology, 58, 127–197.
- Carnevali, L., Montano, N., Statello, R., Coudé, G., Vacondio, F., Rivara, S., … Sgoifo, A. (2017). Social stress contagion in rats: Behavioural, autonomic and neuroendocrine correlates. Psychoneuroendocrinology, 82, 155–163. doi:10.1016/j.psyneuen.2017.05.017
- Carola, V., D'Olimpio, F., Brunamonti, E., Mangia, F., & Renzi, P. (2002). Evaluation of the elevated plus-maze and open-field tests for the assessment of anxiety-related behaviour in inbred mice. Behavioural Brain Research, 134, 49–57. doi:10.1016/S0166-4328(01)00452-1
- Carter, C.S., & Getz, L.L. (1993). Monogamy and the prairie vole. Scientific American, 268, 100–106. doi:10.1038/scientificamerican0693-100
- Choleris, E., Thomas, A.W., Kavaliers, M., & Prato, F.S. (2001). A detailed ethological analysis of the mouse open field test: Effects of diazepam, chlordiazepoxide and an extremely low frequency pulsed magnetic field. Neuroscience and Biobehavioral Reviews, 25, 235–260. doi:10.1016/s0149-7634(01)00011-2
- Cryan, J.F., Mombereau, C., & Vassout, A. (2005). The tail suspension test as a model for assessing antidepressant activity: Review of pharmacological and genetic studies in mice. Neuroscience & Biobehavioral Reviews, 29, 571–625. doi:10.1016/j.neubiorev.2005.03.009
- Eisenberger, N.I., Lieberman, M.D., & Williams, K.D. (2003). Does rejection hurt? An fMRI study of social exclusion. Science, 302, 290–292. doi:10.1126/science.1089134
- Engert, V., Linz, R., & Grant, J.A. (2019). Embodied stress: The physiological resonance of psychosocial stress. Psychoneuroendocrinology, 105, 138–146. doi:10.1016/j.psyneuen.2018.12.221
- Engert, V., Plessow, F., Miller, R., Kirschbaum, C., & Singer, T. (2014). Cortisol increase in empathic stress is modulated by emotional closeness and observation modality. Psychoneuroendocrinology, 45, 192–201. doi:10.1016/j.psyneuen.2014.04.005
- Finnell, J.E., Lombard, C.M., Padi, A.R., Moffitt, C.M., Wilson, L.B., Wood, C.S., & Wood, S.K. (2017). Physical versus psychological social stress in male rats reveals distinct cardiovascular, inflammatory and behavioral consequences. PLoS One, 12, e0172868 doi:10.1371/journal.pone.0172868
- Glaser, R., & Kiecolt-Glaser, J.K. (2005). Stress-induced immune dysfunction: Implications for health. Nature Reviews Immunology, 5, 243–251. doi:10.1038/nri1571
- Gould, T.D., Dao, D.T., & Kovacsics, C.E. (2009). The open field test. In T. Gould (Ed.), Mood and anxiety related phenotypes in mice neuromethods (Vol. 42). Totowa, NJ: Humana Press.
- Grippo, A.J., Ihm, E.D., Wardwell, J., McNeal, N., Scotti, M.L., Moenk, D.A., … Preihs, K. (2014). The effects of environmental enrichment on depressive- and anxiety-related behaviors in socially isolated prairie voles. Psychosomatic Medicine, 76, 277–284. doi:10.1097/PSY.0000000000000052
- Grippo, A.J., Lamb, D.G., Carter, C.S., & Porges, S.W. (2007). Cardiac regulation in the socially monogamous prairie vole. Physiology & Behavior, 90, 386–393. doi:10.1016/j.physbeh.2006.09.037
- Grippo, A.J., Lamb, D.G., Carter, C.S., & Porges, S.W. (2007). Social isolation disrupts autonomic regulation of the heart and influences negative affective behaviors. Biological Psychiatry, 62, 1162–1170. doi:10.1016/j.biopsych.2007.04.011
- Grippo, A.J., Scotti, M.A., Wardwell, J., McNeal, N., Bates, S.L., Chandler, D.L., … Jadia, N. (2018). Cardiac and behavioral effects of social isolation and experimental manipulation of autonomic balance. Autonomic Neuroscience, 214, 1–8. doi:10.1016/j.autneu.2018.08.002
- Hatfield, E., Cacioppo, J., & Rapson, R.L. (1992). Emotional contagion. In M. S. Clark (Ed.), Review of personality and social psychology (Vol. 14 Emotion and Social Behavior, pp. 151–177). Newbury Park, CA: Sage.
- Hennessy, M.B., Kaiser, S., & Sachser, N. (2009). Social buffering of the stress response: Diversity, mechanisms, and functions. Frontiers in Neuroendocrinology, 30, 470–482. doi:10.1016/j.yfrne.2009.06.001
- Hostinar, C.E., Sullivan, R.M., & Gunnar, M.R. (2014). Psychobiological mechanisms underlying the social buffering of the hypothalamic-pituitary-adrenocortical axis: A review of animal models and human studies across development. Psychological Bulletin, 140, 256–282. doi:10.1037/a0032671
- Iñiguez, S.D., Flores-Ramirez, F.J., Riggs, L.E., Alipo, J.B., Garcia, I., Hernandez, M.A., … Castillo, S.A. (2018). Vicarious social defeat stress induces depression-related outcomes in female mice. Biological Psychiatry, 83, 9–17. doi:10.1016/j.biopsych.2017.07.014
- Kavaliers, M., Colwell, D.D., & Choleris, E. (2003). Learning to fear and cope with a natural stressor: Individually and socially acquired corticosterone and avoidance responses to biting flies. Hormones and Behavior, 43, 99–107. doi:10.1016/S0018-506X(02)00021-1
- Langford, D.J., Crager, S.E., Shehzad, Z., Smith, S.B., Sotocinal, S.G., Levenstadt, J.S., … Mogil, J.S. (2006). Social modulation of pain as evidence for empathy in mice. Science, 312, 1967–1970. doi:10.1126/science.1128322
- Levenson, R.W. (2003). Blood, sweat, and fears: The autonomic architecture of emotion. Annals of the New York Academy of Sciences, 1000, 348–366. doi:10.1196/annals.1280.016
- McEwen, B.S. (2008). Central effects of stress hormones in health and disease: Understanding the protective and damaging effects of stress and stress mediators. European Journal of Pharmacology, 583, 174–185. doi:10.1016/j.ejphar.2007.11.071
- McNeal, N., Appleton, K.M., Johnson, A.K., Scotti, M.L., Wardwell, J., Murphy, R., … Grippo, A.J. (2017). The protective effects of social bonding on behavioral and pituitary-adrenal axis reactivity to chronic mild stress in prairie voles. Stress, 20, 175–182. doi:10.1080/10253890.2017.1295444
- McNeal, N., Scotti, M.L., Wardwell, J., Chandler, D.L., Bates, S.L., LaRocca, M.A., … Grippo, A.J. (2014). Disruption of social bonds induces behavioral and physiological dysregulation in male and female prairie voles. Autonomic Neuroscience, 180, 9–16. doi:10.1016/j.autneu.2013.10.001
- McNeal, N., Watanasriyakul, W.T., Normann, M.C., Akinbo, O.I., Dagner, A., Ihm, E., … Grippo, A.J. (2019). The negative effects of social bond disruption are partially ameliorated by sertraline administration in prairie voles. Autonomic Neuroscience, 219, 5–18. doi:10.1016/j.autneu.2019.03.003
- Meyer, M.L., Masten, C.L., Ma, Y., Wang, C., Shi, Z., Eisenberger, N.I., & Han, S. (2013). Empathy for the social suffering of friends and strangers recruits distinct patterns of brain activation. Social Cognitive and Affective Neuroscience, 8, 446–454. doi:10.1093/scan/nss019
- Meyza, K.Z., Bartal, I.-A., Monfils, M.H., Panksepp, J.B., & Knapska, E. (2017). The roots of empathy: Through the lens of rodent models. Neuroscience & Biobehavioral Reviews, 76, 216–234. doi:10.1016/j.neubiorev.2016.10.028
- Olazábal, D.E., & Young, L.J. (2005). Variability in ‘‘spontaneous’’ maternal behavior is associated with anxiety-like behavior and affiliation in naïve juvenile and adult female prairie voles (Microtus ochrogaster). Developmental Psychobiology, 47, 166–178. doi:10.1002/dev.20077
- Pattij, T., Groenink, L., Hijzen, T.H., Oosting, R.S., Maes, R.A.A., van der Gugten, J., & Olivier, B. (2002). Autonomic changes associated with enhanced anxiety in 5-HT1A receptor knockout mice. Neuropsychopharmacology, 27, 380–390. doi:10.1016/S0893-133X(02)00317-2
- Preston, S.D., & De Waal, F. (2002). Empathy: Its ultimate and proximate bases. Behavioral and Brain Sciences, 25, 1–20. doi:10.1017/S0140525X02000018
- Seibenhener, M.L., & Wooten, M.C. (2015). Use of the open field maze to measure locomotor and anxiety-like behavior in mice. Journal of Visualized Experiments, e52434, 1–6. doi:10.3791/52434
- Shaffer, F., & Ginsberg, J.P. (2017). An overview of heart rate variability metrics and norms. Frontiers in Public Health, 5, 258. doi:10.3389/fpubh.2017.00258
- Sial, O.K., Warren, B.L., Alcantara, L.F., Parise, E.M., & Bolaños-Guzmán, C.A. (2016). Vicarious social defeat stress: Bridging the gap between physical and emotional stress. Journal of Neuroscience Methods, 258, 94–103. doi:10.1016/j.jneumeth.2015.10.012
- Sivaselvachandran, S., Acland, E.L., Abdallah, S., & Martin, L.J. (2018). Behavioral and mechanistic insight into rodent empathy. Neuroscience & Biobehavioral Reviews, 91, 130–137. doi:10.1016/j.neubiorev.2016.06.007
- Smith, A.S., & Wang, Z. (2014). Hypothalamic oxytocin mediates social buffering of the stress response. Biological Psychiatry, 76, 281–288. doi:10.1016/j.biopsych.2013.09.017
- Sterley, T.-L., Baimoukhametova, D., Füzesi, T., Zurek, A.A., Daviu, N., Rasiah, N.P., … Bains, J.S. (2018). Social transmission and buffering of synaptic changes after stress. Nature Neuroscience, 21, 393–403. doi:10.1038/s41593-017-0044-6
- Sun, P., Smith, A.S., Lei, K., Liu, Y., & Wang, Z. (2014). Breaking bonds in male prairie vole: Long-term effects on emotional and social behavior, physiology, and neurochemistry. Behavioural Brain Research, 265, 22–31. doi:10.1016/j.bbr.2014.02.016
- Thayer, J.F., & Lane, R.D. (2009). Claude Bernard and the heart–brain connection: Further elaboration of a model of neurovisceral integration. Neuroscience & Biobehavioral Reviews, 33, 81–88. doi:10.1016/j.neubiorev.2008.08.004
- Wallace, D.G., Martin, M.M., & Winter, S.S. (2008). Fractionating dead reckoning: Role of the compass, odometer, logbook, and home base establishment in spatial orientation. Die Naturwissenschaften, 95, 1011–1026. doi:10.1007/s00114-008-0410-z
- Walsh, R.N., & Cummins, R.A. (1976). The open-field test: A critical review. Psychological Bulletin, 83, 482–504. doi:10.1037/0033-2909.83.3.482
- Weisstaub, N.V., Zhou, M., Lira, A., Lambe, E., González-Maeso, J., Hornung, J.P., … Gingrich, J.A. (2006). Cortical 5-HT2A receptor signaling modulates anxiety-like behaviors in mice. Science, 313, 536–540. doi:10.1126/science.1123432
- Young, K.A., Gobrogge, K.L., Liu, Y., & Wang, Z. (2011). The neurobiology of pair bonding: Insights from a socially monogamous rodent. Frontiers in Neuroendocrinology, 32, 53–69. doi:10.1016/j.yfrne.2010.07.006