Abstract
Fischer 344 (F344) rats are characterized by the hyper-reactive hypothalamic-pituitary-adrenal axis to stressful stimuli, while Lewis (LEW) rats are considered to be hypo-reactive. We studied stress-induced cardiovascular, neuroendocrine, and behavioral responses of adult male F344 and LEW rats subjected to the single (120 min) or the repeated restraint stress (daily 120 min for 1 week). Mean arterial pressure (MAP) and heart rate (HR) were measured in the restrained rats (n = 7–8 for each group) via a catheter inserted into the femoral artery. Baroreceptor sensitivity was evaluated using NO donor sodium nitroprusside and α1-adrenoceptor agonist phenylephrine. The plasma levels of adrenocorticotropic hormone (ACTH), corticosterone, aldosterone, and adrenaline were determined before and during the restraint. Exploratory behavior was tested in open field test. F344 rats exerted the augmented stress-induced increase in plasma ACTH, corticosterone, and adrenaline as well as the impaired endocrine adaptation to the repeated stress compared to LEW rats. F344 rats exhibited higher MAP than LEW rats during single and repeated restraint. Moreover, repeatedly restrained F344 showed elevated HR and diminished baroreflex sensitivity. F344 and LEW rats exhibited similar total locomotor activity and the time spent in the center of open field arena, both parameters being decreased by the repeated restraint. The detailed analysis revealed altered pattern of locomotor behavior in F344 rats subjected to repeated restraint. In conclusion, F344 rats showed the impaired endocrine adaptation that resulted in allostatic overload, which might contribute to the impaired cardiovascular and behavioral adaptation to chronic stress observed in this strain.
F344 rats, characterized by HPA axis hyper-reactivity, exhibited higher blood pressure during restraint than LEW rats. Moreover, repeatedly restrained F344 rats showed elevated heart rate and impaired baroreflex sensitivity. It can be concluded that a poor adaptation to the repeated stress in F344 rats is not only limited to the neuroendocrine response but also has important cardiovascular consequences.
Lay summary
Introduction
The most common stress-induced allostatic responses involve the activation of sympathetic nervous system, sympathetic adreno-medullary system, and hypothalamic-pituitary-adrenal (HPA) axis (Koolhaas et al., Citation2011; Ulrich-Lai & Herman, Citation2009). The sympathetic nervous system and adrenal medulla provide the immediate cardiovascular responses to stressor exposure typically consisting of the increase in blood pressure (BP), heart rate (HR), and cardiac output (Vavřínová et al., Citation2019). The stress-induced cardiovascular responses can be modulated by baroreceptor reflex, which is a crucial mechanism keeping BP close to a particular set point (Crestani, Citation2016). The stress-induced activation of HPA axis leads to the elevation of circulating glucocorticoids, which not only promote the mobilization of stored energy but also potentiate numerous sympathetically mediated effects, such as peripheral vasoconstriction (Mocayar Marón et al., Citation2019). The hormonal mediators of stress-induced responses (glucocorticoids and catecholamines) have short-time beneficial effects. Nevertheless, frequent or prolonged exposure to the stressors is associated with allostatic load which represents the cumulative cost of chronic stress (McEwen, Citation1998). The progressive reductions of cardiovascular (Benini et al., Citation2019; Nyhuis et al., Citation2016), endocrine (Bhatnagar et al., Citation2006; Daviu et al., Citation2014), and behavioral (Gagliano et al., Citation2008) responses to the repeated exposure to stressors (adaptation) is an important process that decreases the deleterious consequences of long-term stress on well-being and survival of the organism.
Inbred Lewis (LEW) and Fischer 344 (F344) histocompatible rat strains provide a comparative model for the investigation of interactions between nervous, endocrine and immune systems (Dhabhar et al., Citation1993; Sternberg, Hill, et al., Citation1989). LEW rats are characterized by a high susceptibility to immune/inflammatory challenge resulting from the defective HPA axis responsiveness (Sternberg, Hill, et al., Citation1989; Sternberg, Young, et al., 1989). LEW rats also exhibit HPA axis hypo-reactivity to various stress stimuli, such as restraint stress, open field (OF) exposure, forced swimming test, as compared to F344 rats (Sternberg et al., Citation1992). The HPA axis reactivity of LEW rats in comparison to other “normal” strains (e.g. Sprague Dawley, Brown Norway or Wistar-Kyoto rats) was reported to be similar or decreased, depending on the character of stressful stimuli and on the design of the experiment (Armario et al., Citation1995; Dhabhar et al., Citation1995, Sternberg et al., Citation1992). On the other hand, the HPA axis of F344 rats is hyper-responsive to a number of stressors as well as to immune challenge compared to LEW rats and other rat strains (Armario et al., Citation1995; Dhabhar et al., Citation1993, Citation1995; Sternberg et al., Citation1992). Moreover, F344 rats show a poor adaptation of corticosterone response to the repeated restraint stress compared to LEW and Sprague Dawley rats (Dhabhar et al., Citation1997, Uchida et al., Citation2008).
Exogenous glucocorticoids enhance BP response to the restraint stress (Scheuer et al., Citation2007) and their prolonged administration leads to hypertension development (Ong et al., Citation2009). Moreover, glucocorticoids reduce the gain of baroreceptor reflex control of HR and shift the set point of the baroreflex toward higher BP values (Scheuer & Bechtold, Citation2002). We tested the hypothesis that HPA axis hyper-reactivity in Fischer 344 rats is accompanied by an impaired baroreceptor reflex control of HR and by an exaggerated cardiovascular response to the single restraint stress as compared to LEW rats. We also hypothesized that the impaired ability of Fischer 344 rats to adapt their neuroendocrine response to repeated exposure to stressors might result in allostatic overload and maladaptive cardiovascular and behavioral changes.
Materials and methods
Animals
Male F344 and LEW rats were purchased from Charles River (Germany) at the age of 4 weeks (n = 16 for each strain). Animals were housed (2–3 in cage) in a temperature-controlled room (23 ± 1 °C), with a 12/12 h light/dark cycle (lights on at 6.00am). Animals had ad libitum access to a standard rat chow (Altromin 1324; Altromin, Lage, Germany) and tap water. At the age of 22–24 weeks, one series of rats (n = 8 for F344, n = 8 for LEW rats) was subjected to a single restraint protocol and the second series to a repeated restraint protocol (n = 8 for F344, n = 7 for LEW rats). Schematic representations of experimental procedures are depicted in . After the end of the experiments, the rats were sacrificed with an overdose of anesthetic and cardiac exsanguination. Thymus and adrenal glands were removed and weighed. Experiments were performed between 12.00 and 2.00pm. All experiments were conducted in accordance with the guidelines of the Animal Protection Law of the Czech Republic and approved by Animal Care and Use Committee of the Institute of Physiology, Czech Academy of Sciences, Prague, Czech Republic.
Restraint stress
The rats were horizontally placed into the transparent plastic cylinder (6.5 cm inner diameter; adjustable in length depending on the animal size) equipped with ventilation holes (Vavřínová et al., 2019). In the single stress protocol, the animals were subjected to one session of restraint stress lasting 120 min. In the repeated stress protocol, the animals were subjected to the same stress paradigm for 8 days ().
BP and HR measurement
One day prior BP measurement, the rats were anesthetized with isoflurane (5% for the induction and 2–3% for the maintenance of anesthesia; Forane, AbbVie, USA). Polyethylene catheters with heparinized saline were inserted into the left femoral artery (PE50) and jugular vein (PE10), tunneled subcutaneously and exteriorized in the interscapular region (Behuliak et al., Citation2018). BP and HR were recorded continuously during the entire single stress session and during the last session of the repeated stress experiment using PowerLab system (ADInstruments Ltd, Bella Vista, NSW, Australia). The courses of BP and HR were divided into 5-min intervals and averaged mean arterial pressure (MAP) and HR were calculated for each interval.
Baroreceptor reflex control of HR
At the end of restraint session in which BP was measured (in the single and the repeated restraint protocol, see ), baroreceptor reflex control of HR was determined. Intravenous infusion of the nitric oxide donor sodium nitroprusside (100 µg/ml at 0.5–4 ml/h; Sigma-Aldrich, St. Louis, MO, USA) or the α1-adrenoceptor agonist phenylephrine (100 µg/ml at 0.5–4 ml/h; Sigma-Aldrich, St. Louis, MO, USA) was performed using an infusion pump to alter BP over a wide range and to cause the reflex tachycardia or bradycardia, respectively. Paired values of MAP and HR changes evoked by sodium nitroprusside and phenylephrine infusion were plotted. Baroreceptor-HR reflex sensitivity was calculated as the slopes of regression lines separately for the nitroprusside-induced tachycardia and for the phenylephrine-induced bradycardia (Behuliak et al., Citation2018).
Blood sampling and measurement of hormone levels
To assess the basal hormone levels in stress-naïve rats (referred to as absolute controls in the single stress experiment) and rats subjected to 7 restraint sessions + 1-day recovery (referred to as the adapted controls in the repeated stress experiment), 500 µl of blood was collected from the femoral artery into K3EDTA coated tubes (Sarstedt, Germany) and replaced with sterile saline. To assess stress-induced changes in the hormone levels, blood was collected 10 and 120 min after the beginning of the single stress session or after the beginning of the last session of the repeated stress experiment. All blood samples were centrifuged (at 4 °C, for 10 min at 1500 g), plasma was frozen on dry ice and stored at –80 °C for further analysis. Plasma levels of hormones were measured using commercial assays: adrenocorticotropic hormone (ACTH) ELISA (MD Bioproducts, Zurich, Switzerland), Corticosterone rat/mouse ELISA (LDN, Nordhorn, Germany), Aldosterone ELISA (LDN) and Adrenaline Research ELISA (LDN) according to the manufacturer’s instructions. The absorbance was read on microplate reader Tecan Infinite M200 (Tecan Group Ltd., Männedorf, Switzerland).
OF test
OF test was performed in stress-naïve rats (before the session 1) and in chronically stressed rats (before the session 7 of the repeated stress experiment, ). The animals were transferred to the experimental room 40 min before the testing to adapt to the environment. The test was performed in the OF arena that comprised a square black box (80 × 80 × 40 cm). The animals were placed individually in the front-left corner of the OF. The behavior was recorded for 10 min by a video camera and analyzed using the tracking software EthoVision (Noldus Information Technology, The Netherlands). The following parameters were evaluated: distance traveled (cm), center time (the total time spent in the central section of the arena), total number and total duration of rearing as well as total number and total duration of grooming bouts. The rearing (an upright posture, both against and away from the wall) and grooming bouts (taking care of the body including scratching, fur licking, and face washing) were analyzed automatically using the EthoVision Multiple Body Point Module to track the nose, body center and tail base point of rats and EthoVision Behavior Recognition Module. The detailed analysis of the distance traveled was performed by dividing 10-min session into 2-min intervals to assess the intra-session habituation to the test environment (Mikulecká et al., Citation2014).
Statistical analysis
The data are expressed as the means ± SEM. Separate two-way ANOVAs were performed when comparing the effect of strain (LEW vs. F344 rats) within the single or the repeated restraint protocol. For the comparison of time courses of BP and HR, two-way repeated measures ANOVA with the main effect of strain and time (within-subject repeated factor) was used. The slopes of baroreflex sensitivity were analyzed using two-way ANOVA with the main effects of strain and baroreflex arm (tachycardic vs. bradycardic response). Plasma hormone levels were analyzed using two-way ANOVA with the main effects of strain and stress (stress-naïve vs. 10 min restraint or 120 min restraint). The effect of repeated stress adaptation (the single vs. the repeated restraint protocol) on all abovementioned parameters was analyzed using two-way ANOVA separately within the particular rat strain. The behavioral parameters in the OF test were analyzed by repeated measures two-way ANOVA with between-group factor strain and within-subject factor (stress-naïve vs. repeatedly restrained rats). Follow-up comparisons of the means comprising the main effects or simple effects of significant interactions were conducted using Bonferroni post hoc test. The differences were considered to be significant at p < .05.
Results
Body and organ weights
F344 rats had a lower body weight than LEW rats (355 ± 3 g vs. 462 ± 7 g; p < .001). The repeated restraint led to the body weight decrease in both strains (F344 rats: –15 ± 2 g in; LEW rats −17 ± 2 g; p < .001 in both strains). The relative thymus weight was lower in F344 compared to LEW rats and the repeated restraint caused thymic atrophy in both strains. F344 and LEW rats did not differ in the relative adrenal weights and the repeated restraint resulted in adrenal hypertrophy in both strains ().
Table 1. The relative adrenal and thymus weights in F344 and LEW rats exposed to a single or to a repeated restraint stress.
BP and HR
MAP and HR decreased with the time spent in the restrainer during both the single and the repeated restraint stress in both strains (). F344 rats had a higher MAP than LEW rats in both the single and the repeated restraint (). HR was similar in F344 and LEW rats in the single stress experiment (), while the repeated stress was associated with a higher HR in F344 than in LEW rats (). F344 rats had lower MAP between 65 and 120 min during the repeated restraint compared to the single restraint (repeated stress adaptation: F(1,253) = 4.53, p = .057; time: F(23,253) = 10.19, p < .001; interaction: F(23,253) = 3.18, p < .001). LEW rats had lower MAP during the whole period of repeated restraint when compared to LEW rats stressed only once (repeated stress adaptation: F(1,299) = 20.43, p < .001; time: F(23,253) = 7.95, p < .001; interaction: F(1,299) = 1.07, p = .374). The repeatedly stressed F344 rats had moderately higher HR compared to F344 rats stressed once (repeated stress adaptation: F(1,253) = 2.06, p = .179; time: F(23,253) = 14.85, p < .001; interaction: F(23,253) = 2.78, p < .001). LEW rats had a similar HR response during the single and the repeated stress (repeated stress adaptation: F(1,299) = 3.66, p = .078; time: F(23,253) = 8.96, p < .001; interaction: F(23,299) = 0.72, p = .830).
Figure 2. The effect of single and repeated restraint on the cardiovascular response in F344 and LEW rats. The time-course of mean arterial pressure (A, B) and heart rate (C, D) during single (A, C) and repeated (B, D) restraint. Each point represents a group mean ± SEM of the averaged 5-min interval; n = 6–7 for F344 rats, n = 7–8 for LEW rats. The main effects of strain (LEW vs. F344 rats) and time (within-animal repeated factor) were tested by repeated measures two-way ANOVA and the results are listed below each graph. Bonferroni post hoc test was performed.
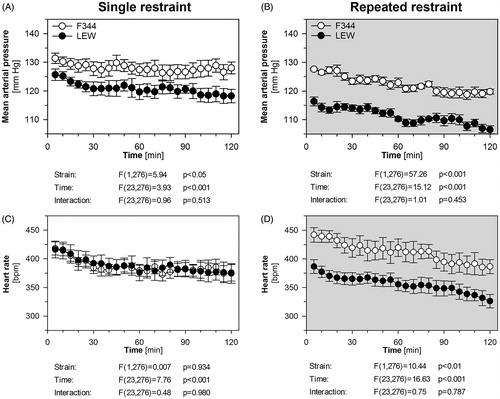
Baroreceptor-HR reflex
Baroreceptor-HR reflex sensitivity was calculated as the slopes of regression lines from either nitroprusside-induced MAP reduction associated with a reflex tachycardia or phenylephrine-induced MAP elevation associated with a reflex bradycardia. After a single restraint stress, F344 and LEW rats had similar baroreflex sensitivity of both arms of baroreceptor-HR reflex (). The slope of tachycardic response to sodium nitroprusside was –5.03 ± 0.96 bpm/mm Hg in F344 rats and –7.02 ± 1.79 bpm/mm Hg in LEW rats. The slope of bradycardic response to phenylephrine was –0.71 ± 0.22 and –1.92 ± 0.66 bpm/mm Hg in F344 and LEW rats, respectively. The repeatedly stressed F344 and LEW differed in their tachycardic response to nitroprusside but the bradycardic response to phenylephrine was similar in both strains (). The slope of the tachycardic response to sodium nitroprusside was –1.91 ± 0.84 and –7.21 ± 1.58 bpm/mm Hg in repeatedly stressed F344 and LEW rats, respectively. There was a similar slope of the bradycardic response to phenylephrine in F344 and LEW rats (–1.59 ± 0.13 bpm/mm Hg vs. –1.43 ± 0.78 bpm/mm Hg). The repeated stress was associated with an alteration of the baroreceptor-HR reflex in F344 rats (repeated stress adaptation: F(1,22) = 2.39, p = .137; baroreflex arm: F(1,22) = 10.32, p < .01; interaction: F(1,22) = 7.67, p < .05). Specifically, the tachycardic response to nitropruside was diminished in the repeatedly stressed F344 rats (Bonferroni post hoc test: p < .01). The repeated stress had no effect on the baroreceptor-HR reflex in LEW rats (repeated stress adaptation: F(1,21) = .01, p = .920; baroreflex arm: F(1,21) = 13.87, p < .01; interaction: F(1,21) = .05, p = .819).
Figure 3. The blood pressure and heart rate response of F344 and LEW rats to the intravenous administration of sodium nitroprusside and phenylephrine after single restraint (A) and repeated restraint (B). Baroreceptor-heart rate reflex sensitivity was calculated as the slopes of regression lines separately for the nitroprusside-induced tachycardia and for the phenylephrine-induced bradycardia. Each point represents a group mean ± SEM, n = 6–7 for F344 rats, n = 5–6 for LEW rats. The slopes of baroreflex sensitivity were analyzed using two-way ANOVA with main effects of strain (LEW vs. F344 rats) and baroreflex arm (tachycardic vs. bradycardic response) and the results are listed below each graph. Bonferroni post hoc test was performed.
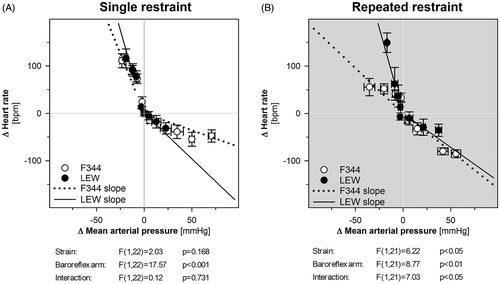
Basal hormone levels in plasma
The basal plasma levels of ACTH, corticosterone, aldosterone and adrenaline were measured in absolute control (stress-naïve) and in adapted control rats (7× restraint and 1-day recovery). F344 and LEW rats did not differ in their basal plasma levels of ACTH, corticosterone, and adrenaline, whereas the basal levels of aldosterone were lower in F344 than LEW rats (). The adapted control rats of both strains had higher corticosterone, aldosterone, and adrenaline levels than stress-naïve animals ().
Table 2. The basal plasma levels of ACTH, corticosterone, aldosterone, and adrenaline in absolute control (stress-naïve) and adapted control (7× restraint and 1-day recovery) F344 and LEW rats.
Stress-induced changes in plasma hormone levels
ACTH plasma levels were elevated after 10 min of single or repeated restraint stress in rats of both strains and this increase was significantly higher in F344 rats (). After 120 min of restraint, ACTH plasma levels returned to the pre-stress values in both strains. Plasma ACTH levels were similar in F344 rats subjected to single or repeated restraint (repeated stress adaptation: F(1,34) = .08, p = .776; stress exposure: F(2,34) = 124.60, p < .001; interaction: F(2,34) = 2.51, p = .096). On the other hand, the repeatedly stressed LEW rats had lower stress-induced ACTH levels than the animals in the single stress experiment (repeated stress adaptation: F(1,36) = 19.23, p < .001; stress exposure: F(2,36) = 62.20, p < .001; interaction: F(2,36) = 4.58, p < .05). In LEW rats, the plasma ACTH was lower 10 min after the beginning of the repeated stress compared to the single stress experiment (Bonferroni post hoc test: p < .001).
Figure 4. The effect of single and repeated restraint on the plasma hormone levels in F344 and LEW rats. Adrenocorticotropic hormone (ACTH; A, B), corticosterone (C, D), aldosterone (E, F), and adrenaline (G, H) in single (left panels) and repeated (right panels) restraint protocol. Results are expressed as group means ± SEM; n = 6–8 for F344 rats, n = 7–8 for LEW rats. Plasma hormone levels were analyzed using two-way ANOVA with the main effects of strain (LEW vs. F344 rats) and stress (stress-naive vs. 10 min restraint or 120 min restraint), the results are listed below each graph. Bonferroni post hoc test was performed, *p < .05 versus respective control group, †p < .05 F344 versus LEW rats.
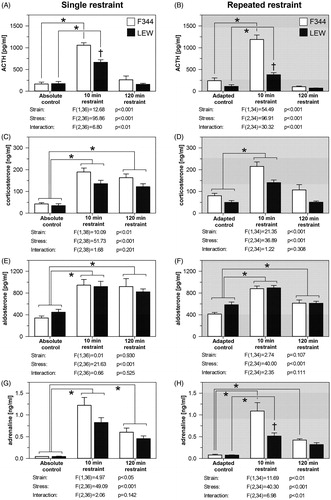
The plasma corticosterone levels were elevated after 10 and 120 min of single restraint in both strains (). In the repeated restraint experiment, the plasma corticosterone was elevated only after 10 min, while it returned to the pre-stress values in both strains after 120 min (). Stress-induced plasma corticosterone levels were higher in F344 than in LEW rats in both single and repeated restraint experiments. In comparison to the single restraint, rats of both strains had lower stress-induced corticosterone levels after 120 min of repeated restraint stress, F344 rats (repeated stress adaptation: F(1,35) = .03, p = .864; stress exposure: F(2,35) = 38.27, p < .001; interaction: F(2,35) = 4.843, p < .05) and LEW rats (repeated stress adaptation: F(1,37) = 3.58, p = .066; stress exposure: F(2,37) = 38.05, p < .001; interaction: F(2,37) = 8.96, p < .001), which was also confirmed by Bonferroni post hoc test (p < .05 in F344 rats; p < .001 in LEW rats).
The plasma aldosterone levels were elevated after 10 and 120 min of single and repeated restraint stress in both strains (). The repeatedly stressed F344 rats had similar stress-induced aldosterone levels as F344 rats stressed once (repeated stress adaptation: F(1,34) = 2.10, p = .156; stress exposure: F(2,34) = 22.03, p < .001; interaction: F(2,34) = 2.56, p = .091). In comparison to the single restraint, LEW rats had significantly lower stress-induced aldosterone levels after 120 min of repeated restraint stress (repeated stress adaptation: F(1,36) = 0.47, p = .495; stress exposure: F(2,36) = 22.19, p < .001; interaction: F(2,36) = 4.41, p < .05), confirmed by Bonferroni post hoc test (p < .05).
The plasma levels of adrenaline were increased after 10 and 120 min of single restraint in both strains. Stress-induced plasma adrenaline levels were higher in F344 than in LEW rats (). The adrenaline levels were also elevated in repeatedly stressed F344 rats after both 10 and 120 min, whereas in LEW rats the adrenaline levels were significantly elevated only after 10 min of repeated restraint. Moreover, this elevation after 10 min of repeated restraint was lower in LEW compared to F344 rats (). The once and repeatedly stressed F344 rats had similar stress-induced adrenaline levels (repeated stress adaptation: F(1,34) = 0.23, p = .331; stress exposure: F(2,34) = 46.23, p < .001; interaction F(2,34) = 0.50, p = .609). In comparison to the single restraint, LEW rats had significantly lower stress-induced adrenaline levels after 10 min of repeated restraint stress (repeated stress adaptation: F(1,36) = 7.51, p < .01; stress exposure: F(2,36) = 47.61, p < .001; interaction: F(2,36) = 3.76, p < .05), confirmed by Bonferroni post hoc test (p < .05).
OF test
The total distance traveled in OF was similar in the stress-naïve F344 and LEW rats. Repeated stress was associated with a decreased total distance traveled in both strains (). The animals were highly active in the first 2-min interval of the session while their locomotor activity decreased with time (intra-session habituation). The stress-naïve F344 and LEW rats did not differ in their intra-session habituation of locomotor activity during the first OF session (). After the repeated restraint, F344 and LEW rats differed in the intra-session habituation, since the locomotor activity of F344 rats did not change during OF session (). F344 rats were less active in the first 2-min interval but more active in the last 2-min interval of the session than LEW rats (Bonferroni post hoc tests: p < .001 and p < .05, respectively). The repeatedly stressed LEW rats were generally less active than the stress-naïve rats but the time course of intra-session habituation was similar (repeated stress adaptation: F(1,24) = 11.41, p < .05; time: F(4,24) = 52.62, p < .001; interaction: F(4,24) = 2.27, p = .091). On the other hand, we observed a difference in the intra-session habituation between the stress-naïve and the repeatedly stressed F344 rats (repeated stress adaptation: F(1,28) = 3.32, p = .111; time: F(4,28) = 1.54, p = .217; interaction: F(4,28) = 2.95, p < .05). The repeatedly stressed F344 rats were less active in the first 2-min interval of the session than the stress-naïve F344 rats (Bonferroni post hoc test: p < .001). This result suggests that the exposure of F344 rats to the repeated stress lead to the impairment of habituation. The time spent in the center of the OF was similar in F344 and LEW rats. The animals spent less time in the center of arena after the repeated restraint (). No strain differences were found in the rearing duration. The total time spent in the rearing decreased following the repeated stress (). F344 rats spent more time in grooming than LEW rats, but the repeated stress did not affect their grooming behavior (). Similar results were obtained when analyzing the rearing and the grooming frequency (data not shown).
Figure 5. The behavior of F344 and LEW rats in the open field test before and after repeated restraint. The total distance traveled (A), the time-course of locomotor activity in the open field in stress-naïve (B) and repeatedly restrained (C) rats, time spent in the center (D), total time spent in rearing (E) and grooming (F) behavior. The results are expressed as group means ± SEM; n = 8 for F344 rats, n = 7 for LEW rats. The main effects of strain (LEW vs. F344 rats) and protocol (stress-naïve vs. repeated restraint) were tested by repeated measures two-way ANOVA and the results are listed below each graph. Bonferroni post hoc test was performed, *p < .05 stress-naïve versus repeated restraint group.
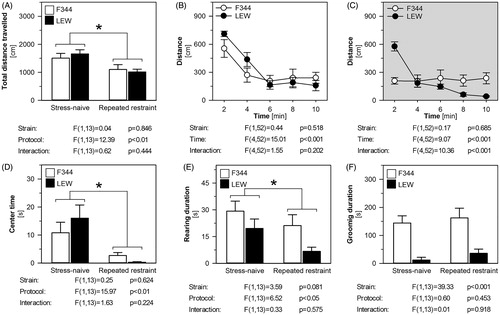
Discussion
Our study revealed several differences in the stress-induced cardiovascular, neuroendocrine, and behavioral responses between stress hyper-responsive F344 and hypo-responsive LEW rats subjected to a single or a repeated restraint stress. As expected, in the both single and the repeated stress F344 rats showed augmented response of plasma ACTH, corticosterone, and adrenaline compared to LEW rats, but we did not observe any strain-dependent difference in plasma aldosterone levels. Moreover, F344 rats exhibited poor adaptation of plasma ACTH and adrenaline response to the repeated restraint. Higher corticosterone levels in F344 rats might contribute to the increased BP observed in this strain during the single or the repeated restraint. We also found an elevated HR and an impaired baroreflex sensitivity in repeatedly restrained F344 rats compared to LEW rats. The repeated exposure to stress decreased total locomotor activity, time spent in the center of the OF arena and the rearing behavior in both strains. The detailed analysis revealed an impaired habituation of locomotor behavior of F344 rats subjected to the repeated restraint.
Basal plasma levels of ACTH, corticosterone, and adrenaline were similar in F344 and LEW rats. The basal ACTH and corticosterone levels were higher than reported previously (Dhabhar et al., Citation1993; Moncek et al., Citation2001), which might be a result of sensitization of animals subjected to procedure of catheterization. F344 rats showed exaggerated ACTH, corticosterone, and adrenaline responses to the acute stress in compared to LEW rats, which is in accordance with previously published results (Dhabhar et al., Citation1993; Elenkov et al., Citation2008; Moncek et al., Citation2001; Sternberg et al., Citation1992). The basal plasma aldosterone levels were lower in F344 rats and aldosterone response to the restraint was similar in F344 and LEW rats. Aldosterone is the major mineralocorticoid involved in the regulation of fluid volume and electrolyte balance. Its secretion is triggered by increased plasma angiotensin II and/or potassium levels, whereas ACTH is considered be a secondary regulator of aldosterone production (Hattangady et al., Citation2012). The renin-angiotensin-aldosterone system plays an important role in the regulation of BP and its excessive activation contributes to the pathophysiology of hypertension. F344 rats subjected to the single restraint exhibited elevated BP in comparison to LEW rats, but this finding cannot be attributed to the strain-differences in plasma aldosterone levels. However, the elevated plasma adrenaline and corticosterone levels in F344 rats might contribute to higher BP observed in this strain during the single restraint. The administration of glucocorticoids enhances BP response to the restraint stress (Scheuer et al., Citation2007). It is widely accepted that glucocorticoids exert permissive effects on cardiovascular system through the potentiation of catecholamine action in both vascular and cardiac tissue (Sapolsky et al., Citation2000; Ullian, Citation1999). Recently we documented in Wistar rats that the suppression of glucocorticoid synthesis (pharmacological adrenalectomy) resulted in the decreased sensitivity of BP to noradrenaline administration (Bencze et al., Citation2020). Moreover, Yagil and Krakoff (Citation1988) demonstrated that dexamethasone administration restored BP sensitivity to noradrenaline in surgically adrenalectomized Sprague-Dawley rats. The exaggerated corticosterone levels in restrained F344 rats were probably caused by the increased plasma levels of ACTH, which might be associated with higher stress-induced mRNA expression of corticotropin-releasing hormone (CRH) in the paraventricular hypothalamic nucleus reported in F344 compared to LEW rats (Sternberg et al., Citation1992). However, LEW rats are characterized by a defective synthesis and secretion of CRH to inflammatory and stress stimuli (Sternberg, Young, et al., Citation1989; Sternberg et al., Citation1992). Taken together, higher BP in F344 rats subjected to the single restraint might be associated with the augmented neuroendocrine response of HPA axis in hyper-reactive F344 rats compared to hypo-reactive LEW rats.
In the repeated restraint protocol, F344 rats showed exaggerated stress-induced response of plasma ACTH, corticosterone, and adrenaline compared to LEW rats similarly to the single restraint protocol. The adaptability to repeated restraint was analyzed separately within the particular rat strain. The adaptation of corticosterone response was observed in repeatedly restrained rats of both strains only at the end of stress session. LEW rats also showed reduced response of plasma ACTH and adrenaline to the repeated restraint. It was demonstrated that the repeated exposures to homotypic stressor lead to the progressive reduction of the HPA and adrenaline response in Sprague Dawley or Wistar rats (De Boer et al., Citation1990; Rabasa et al., Citation2015). In contrast, F344 rats showed no adaptation of plasma ACTH and adrenaline response to the repeated exposure to homotypic stressor. The poor endocrine adaptation in F344 rats compared to LEW and Sprague Dawley rats was already reported by other laboratories (Dhabhar et al., Citation1997; Uchida et al., Citation2008). The impaired adaptation of stress-induced adrenaline response observed in F344 rats is in line with our previous study in which 3-day variable stress protocol led to a greater induction of mRNA expression of genes involved in adrenaline biosynthesis, tyrosine hydroxylase (Th) and phenylethanolamine N-methyltransferase (Pnmt), in adrenal medulla of F344 rats compared to LEW rats (Ergang et al., Citation2015). The transcription of Pnmt gene is regulated by glucocorticoids (Tai et al., Citation2002), thus higher plasma adrenaline level in F344 rats might be associated with higher corticosterone levels in this strain. In the repeated restraint protocol, F344 rats had not only a higher BP but also an increased HR and an impaired baroreflex sensitivity (attenuated reflex tachycardia) compared to LEW rats. These cardiovascular changes in the repeatedly restrained F344 rats might be related to higher corticosterone levels and to the attenuated endocrine adaptation of these animals. Glucocorticoid type II receptor antagonist mifepristone lowered HR in conscious Sprague Dawley rats (Scheuer & Bechtold, Citation2002) and in mice subjected to chronic stress (Balkaya et al., Citation2011). Moreover, glucocorticoids are known to attenuate baroreflex function (Scheuer & Bechtold, Citation2002).
Thymic atrophy is often referred to as a marker of chronic stress (Gruver & Sempowski, Citation2008) and it is caused by increased glucocorticoid levels (Tarcic et al., Citation1998). Accordingly, HPA axis hyper-reactive F344 rats had lower relative thymus weight compared to LEW rats and the repeated restraint caused thymic atrophy in both strains. Chronic stress usually leads to adrenal hypertrophy and/or hyperplasia, which is associated with increased maximal corticosterone responses to ACTH (Ulrich-Lai et al., Citation2006). In our study, the repeated restraint resulted in adrenal hypertrophy in both strains, but it was not accompanied by an augmented stress-induced corticosterone or adrenaline release. This finding suggests that repeated exposure to restraint (homotypic stressor) became more predictable and controllable stimulus (Koolhaas et al., Citation2011).
The repeated exposure to stress decreased total locomotor activity in the OF test in both strains. Repeatedly stressed F344 rats did not show intra-session habituation of locomotor activity, characterized by a decrement in the exploratory behavior occurring within the time period of an exposure to novel environment (Bolivar, Citation2009; Mikulecká et al., Citation2014). However, we observed markedly lower activity of repeatedly stressed F344 rats during the first 2-min interval of the OF session, which might be caused by enhanced transient anxiety or better memory of the first exposure to OF. The exposure to the repeated stress increased anxiety-like behavior assessed by the amount of time spent in the center part of the OF in both strains. Moreover, the exposure to the repeated restraint decreased the time spent in the rearing in both strains, which indicates that chronic stress affects the nonspecific excitability (emotionality) of animals (Lever et al., Citation2006; Mikulecká et al., Citation2019). The grooming behavior is a specific rodent behavioral response to the stressful situation aiming to restore the homeostatic status and to lower the anxiety produced by a stressful experience (van Erp et al., Citation1994). CRH is considered to be involved in the regulation of grooming behavior (Morley & Levine, Citation1982). Recently, it has been shown that the photoactivation of CRH neurons in paraventricular hypothalamic nucleus induced the grooming behavior in mice (Füzesi et al., Citation2016). In our study, the time spent in the grooming behavior was enhanced in F344 rats which is in line with other reports (Chaouloff et al., Citation1995; Sternberg et al., Citation1992). Accordingly, the higher stress-induced expression of CRH was reported in the paraventricular hypothalamic nucleus of F344 rats (Sternberg et al., Citation1992).
Several limitations of the current study need to be addressed. First, the cardiovascular parameters were measured by a direct recording of arterial pressure by fluid-filled catheters. The important advantage of the catheter system is that it allows intravenous infusions of vasoactive drugs for the measurement of baroreceptor-HR reflex. However, anesthesia and surgical procedure could be considered as stressors, which might alter the cardiovascular parameters and plasma levels of hormones. Second, the baroreflex sensitivity was measured only in the restrained rats. Stress conditions affects baroreceptor-HR reflex, facilitating the tachycardia and shifting the set point for the bradycardic response toward the higher BP values (Crestani et al., Citation2010). Third, the exposure to the OF test can also be seen as a mildly stressful procedure. Thus, we decided not to expose the rats in the single restraint protocol to any potential stressor. Consequently, we performed OF test two times in animals subjected to the repeated restraint protocol (). We are aware that the first exposure to OF might influence the results of the second OF session. However, it has been shown that the habituation in rodents is diminished with increasing intervals between testing sessions (Platel & Porsolt, Citation1982).
In summary, our study demonstrated that HPA axis hyper-reactivity of F344 rats is accompanied by a higher BP during restraint. The repeated restraint revealed an elevated HR and an impaired baroreflex sensitivity in F344 rats compared to LEW rats. From the behavioral point of view, the repeated restraint stress affected the anxiety/emotional behavior in both strains. Repeatedly restrained F344 rats exhibited altered pattern of locomotor behavior. Thus, a poor adaptation to the repeated stress in F344 rats is not limited to the neuroendocrine response but also has important cardiovascular and behavioral consequences. Studying the differences between LEW and F344 rats could provide a further useful information for the elucidation of the detrimental effects of allostatic overload on the cardiovascular system.
Disclosure statement
No potential conflict of interest was reported by the authors.
Additional information
Funding
Notes on contributors
Martin Vodička
Martin Vodicˇka designed the study, performed restraint stress and helped with open field test.
Anna Vavřínová
Anna Vavrˇínová designed the study, performed blood sampling and measurement of hormone levels and wrote the manuscript.
Anna Mikulecká
Martin Vodicˇka designed the study, performed restraint stress and helped with open field test.
Anna Mikulecká performed and analyzed open field test.
Josef Zicha
Martin Vodicˇka designed the study, performed restraint stress and helped with open field test.
Josef Zicha analyzed baroreflex measurement, helped with data interpretation and critical revision of manuscript.
Michal Behuliak
Martin Vodicˇka designed the study, performed restraint stress and helped with open field test.
Michal Behuliak performed blood pressure and baroreflex measurement, prepared figures, performed statistical analyses and wrote the manuscript.
References
- Armario, A., Gavaldà, A., & Martí, J. (1995). Comparison of the behavioural and endocrine response to forced swimming stress in five inbred strains of rats. Psychoneuroendocrinology, 20(8), 879–890. https://doi.org/https://doi.org/10.1016/0306-4530(95)00018-6
- Balkaya, M., Prinz, V., Custodis, F., Gertz, K., Kronenberg, G., Kroeber, J., Fink, K., Plehm, R., Gass, P., Laufs, U., & Endres, M. (2011). Stress worsens endothelial function and ischemic stroke via glucocorticoids. Stroke, 42(11), 3258–3264. https://doi.org/https://doi.org/10.1161/STROKEAHA.110.607705
- Behuliak, M., Bencze, M., Polgárová, K., Kuneš, J., Vaněčková, I., & Zicha, J. (2018). Hemodynamic response to gabapentin in conscious spontaneously hypertensive rats. Hypertension (Dallas, Texas: 1979), 72(3), 676–685. https://doi.org/https://doi.org/10.1161/HYPERTENSIONAHA.118.09909
- Bencze, M., Vavřínová, A., Zicha, J., & Behuliak, M. (2020). Pharmacological suppression of endogenous glucocorticoid synthesis attenuated blood pressure and heart rate response to acute restraint in Wistar rats. Physiological Research, 69(3).
- Benini, R., Oliveira, L. A., Gomes-de-Souza, L., & Crestani, C. C. (2019). Habituation of the cardiovascular responses to restraint stress in male rats: Influence of length, frequency and number of aversive sessions. Stress (Amsterdam, Netherlands), 22(1), 151–161. https://doi.org/https://doi.org/10.1080/10253890.2018.1532992
- Bhatnagar, S., Vining, C., Iyer, V., & Kinni, V. (2006). Changes in hypothalamic-pituitary-adrenal function, body temperature, body weight and food intake with repeated social stress exposure in rats. Journal of Neuroendocrinology, 18(1), 13–24. https://doi.org/https://doi.org/10.1111/j.1365-2826.2005.01375.x
- Bolivar, V. J. (2009). Intrasession and intersession habituation in mice: From inbred strain variability to linkage analysis. Neurobiology of Learning and Memory, 92(2), 206–214. https://doi.org/https://doi.org/10.1016/j.nlm.2009.02.002
- Chaouloff, F., Kulikov, A., Sarrieau, A., Castanon, N., & Mormède, P. (1995). Male Fischer 344 and Lewis rats display differences in locomotor reactivity, but not in anxiety-related behaviours: Relationship with the hippocampal serotonergic system. Brain Research, 693(1–2), 169–178. https://doi.org/https://doi.org/10.1016/0006-8993(95)00733-7
- Crestani, C. C. (2016). Emotional stress and cardiovascular complications in animal models: A review of the influence of stress type. Frontiers in Physiology, 7, 251. https://doi.org/https://doi.org/10.3389/fphys.2016.00251
- Crestani, C. C., Tavares, R. F., Alves, F. H., Resstel, L. B., & Correa, F. M. (2010). Effect of acute restraint stress on the tachycardiac and bradycardiac responses of the baroreflex in rats. Stress (Amsterdam, Netherlands), 13(1), 61–72. https://doi.org/https://doi.org/10.3109/10253890902927950
- Daviu, N., Rabasa, C., Nadal, R., & Armario, A. (2014). Comparison of the effects of single and daily repeated immobilization stress on resting activity and heterotypic sensitization of the hypothalamic-pituitary-adrenal axis. Stress (Amsterdam, Netherlands), 17(2), 176–185. https://doi.org/https://doi.org/10.3109/10253890.2014.880834
- De Boer, S. F., Koopmans, S. J., Slangen, J. L., & Van der Gugten, J. (1990). Plasma catecholamine, corticosterone and glucose responses to repeated stress in rats: Effect of interstressor interval length. Physiology & Behavior, 47(6), 1117–1124. https://doi.org/https://doi.org/10.1016/0031-9384(90)90361-7
- Dhabhar, F. S., McEwen, B. S., & Spencer, R. L. (1993). Stress response, adrenal steroid receptor levels and corticosteroid-binding globulin levels–a comparison between Sprague-Dawley, Fischer 344 and Lewis rats. Brain Research, 616(1–2), 89–98. https://doi.org/https://doi.org/10.1016/0006-8993(93)90196-T
- Dhabhar, F. S., McEwen, B. S., & Spencer, R. L. (1997). Adaptation to prolonged or repeated stress-comparison between rat strains showing intrinsic differences in reactivity to acute stress. Neuroendocrinology, 65(5), 360–368. https://doi.org/https://doi.org/10.1159/000127196
- Dhabhar, F. S., Miller, A. H., McEwen, B. S., & Spencer, R. L. (1995). Differential activation of adrenal steroid receptors in neural and immune tissues of Sprague Dawley, Fischer 344, and Lewis rats. Journal of Neuroimmunology, 56(1), 77–90. https://doi.org/https://doi.org/10.1016/0165-5728(94)00135-B
- Elenkov, I. J., Kvetnansky, R., Hashiramoto, A., Bakalov, V. K., Link, A. A., Zachman, K., Crane, M., Jezova, D., Rovensky, J., Dimitrov, M. A., Gold, P. W., Bonini, S., Fleisher, T., Chrousos, G. P., & Wilder, R. L. (2008). Low- versus high-baseline epinephrine output shapes opposite innate cytokine profiles: Presence of Lewis- and Fischer-like neurohormonal immune phenotypes in humans? Journal of Immunology (Baltimore, MD: 1950), 181(3), 1737–1745. https://doi.org/https://doi.org/10.4049/jimmunol.181.3.1737
- Ergang, P., Vodička, M., Soták, M., Klusoňová, P., Behuliak, M., Řeháková, L., Zach, P., & Pácha, J. (2015). Differential impact of stress on hypothalamic-pituitary-adrenal axis: Gene expression changes in Lewis and Fisher rats. Psychoneuroendocrinology, 53, 49–59. https://doi.org/https://doi.org/10.1016/j.psyneuen.2014.12.013
- Füzesi, T., Daviu, N., Wamsteeker Cusulin, J. I., Bonin, R. P., & Bains, J. S. (2016). Hypothalamic CRH neurons orchestrate complex behaviours after stress. Nature Communications, 7, 11937. https://doi.org/https://doi.org/10.1038/ncomms11937
- Gagliano, H., Fuentes, S., Nadal, R., & Armario, A. (2008). Previous exposure to immobilisation and repeated exposure to a novel environment demonstrate a marked dissociation between behavioral and pituitary-adrenal responses. Behavioural Brain Research, 187(2), 239–245. https://doi.org/https://doi.org/10.1016/j.bbr.2007.09.006
- Gruver, A. L., & Sempowski, G. D. (2008). Cytokines, leptin, and stress-induced thymic atrophy. Journal of Leukocyte Biology, 84(4), 915–923. https://doi.org/https://doi.org/10.1189/jlb.0108025
- Hattangady, N. G., Olala, L. O., Bollag, W. B., & Rainey, W. E. (2012). Acute and chronic regulation of aldosterone production. Molecular and Cellular Endocrinology, 350(2), 151–162. https://doi.org/https://doi.org/10.1016/j.mce.2011.07.034
- Koolhaas, J. M., Bartolomucci, A., Buwalda, B., de Boer, S. F., Flügge, G., Korte, S. M., Meerlo, P., Murison, R., Olivier, B., Palanza, P., Richter-Levin, G., Sgoifo, A., Steimer, T., Stiedl, O., van Dijk, G., Wöhr, M., & Fuchs, E. (2011). Stress revisited: A critical evaluation of the stress concept. Neuroscience and Biobehavioral Reviews, 35(5), 1291–1301. https://doi.org/https://doi.org/10.1016/j.neubiorev.2011.02.003
- Lever, C., Burton, S., & O'Keefe, J. (2006). Rearing on hind legs, environmental novelty, and the hippocampal formation. Reviews in the Neurosciences, 17(1–2), 111–133. https://doi.org/https://doi.org/10.1515/revneuro.2006.17.1-2.111
- McEwen, B. S. (1998). Protective and damaging effects of stress mediators. The New England Journal of Medicine, 338(3), 171–179. https://doi.org/https://doi.org/10.1056/NEJM199801153380307
- Mikulecká, A., Druga, R., Stuchlik, A., Mares, P., & Kubova, H. (2019). Comorbidities of early-onset temporal epilepsy: Cognitive, social, emotional, and morphologic dimensions. Experimental Neurology, 320, 113005. https://doi.org/https://doi.org/10.1016/j.expneurol.2019.113005
- Mikulecká, A., Šubrt, M., Stuchlík, A., & Kubová, H. (2014). Consequences of early postnatal benzodiazepines exposure in rats. I. Cognitive-like behavior. Frontiers in Behavioral Neuroscience, 8, 101.
- Mocayar Marón, F. J., Ferder, L., Saraví, F. D., & Manucha, W. (2019). Hypertension linked to allostatic load: From psychosocial stress to inflammation and mitochondrial dysfunction. Stress (Amsterdam, Netherlands), 22(2), 169–181. https://doi.org/https://doi.org/10.1080/10253890.2018.1542683
- Moncek, F., Kvetnansky, R., & Jezova, D. (2001). Differential responses to stress stimuli of Lewis and Fischer rats at the pituitary and adrenocortical level. Endocrine Regulations, 35(1), 35–41.
- Morley, J. E., & Levine, A. S. (1982). Corticotrophin releasing factor, grooming and ingestive behavior. Life Sciences, 31(14), 1459–1464. https://doi.org/https://doi.org/10.1016/0024-3205(82)90007-8
- Nyhuis, T. J., Masini, C. V., Taufer, K. L., Day, H. E., & Campeau, S. (2016). Reversible inactivation of rostral nucleus raphe pallidus attenuates acute autonomic responses but not their habituation to repeated audiogenic stress in rats. Stress (Amsterdam, Netherlands), 19(2), 248–259. https://doi.org/https://doi.org/10.3109/10253890.2016.1160281
- Ong, S. L., Zhang, Y., Sutton, M., & Whitworth, J. A. (2009). Hemodynamics of dexamethasone-induced hypertension in the rat. Hypertension Research, 32(10), 889–894. https://doi.org/https://doi.org/10.1038/hr.2009.118
- Platel, A., & Porsolt, R. D. (1982). Habituation of exploratory activity in mice: A screening test for memory enhancing drugs. Psychopharmacology, 78(4), 346–352. https://doi.org/https://doi.org/10.1007/BF00433739
- Rabasa, C., Gagliano, H., Pastor-Ciurana, J., Fuentes, S., Belda, X., Nadal, R., & Armario, A. (2015). Adaptation of the hypothalamus-pituitary-adrenal axis to daily repeated stress does not follow the rules of habituation: A new perspective. Neuroscience and Biobehavioral Reviews, 56, 35–49. https://doi.org/https://doi.org/10.1016/j.neubiorev.2015.06.013
- Sapolsky, R. M., Romero, L. M., & Munck, A. U. (2000). How do glucocorticoids influence stress responses? Integrating permissive, suppressive, stimulatory, and preparative actions. Endocrine Reviews, 21(1), 55–89. https://doi.org/https://doi.org/10.1210/er.21.1.55
- Scheuer, D. A., & Bechtold, A. G. (2002). Glucocorticoids modulate baroreflex control of heart rate in conscious normotensive rats. American Journal of Physiology, Regulatory, Integrative and Comparative Physiology, 282(2), R475–R483. https://doi.org/https://doi.org/10.1152/ajpregu.00300.2001
- Scheuer, D. A., Bechtold, A. G., & Vernon, K. A. (2007). Chronic activation of dorsal hindbrain corticosteroid receptors augments the arterial pressure response to acute stress. Hypertension, 49(1), 127–133. https://doi.org/https://doi.org/10.1161/01.HYP.0000250088.15021.c2
- Sternberg, E. M., Glowa, J. R., Smith, M. A., Calogero, A. E., Listwak, S. J., Aksentijevich, S., Chrousos, G. P., Wilder, R. L., & Gold, P. W. (1992). Corticotropin releasing hormone related behavioral and neuroendocrine responses to stress in Lewis and Fischer rats. Brain Research, 570(1–2), 54–60. https://doi.org/https://doi.org/10.1016/0006-8993(92)90563-O
- Sternberg, E. M., Hill, J. M., Chrousos, G. P., Kamilaris, T., Listwak, S. J., Gold, P. W., & Wilder, R. L. (1989). Inflammatory mediator-induced hypothalamic-pituitary-adrenal axis activation is defective in streptococcal cell wall arthritis-susceptible Lewis rats. Proceedings of the National Academy of Sciences of the United States of America, 86(7), 2374–2378. https://doi.org/https://doi.org/10.1073/pnas.86.7.2374
- Sternberg, E. M., Young, W. S., III, Bernardini, R., Calogero, A. E., Chrousos, G. P., Gold, P. W., & Wilder, R. L. (1989). A central nervous system defect in biosynthesis of corticotropin-releasing hormone is associated with susceptibility to streptococcal cell wall-induced arthritis in Lewis rats. Proceedings of the National Academy of Sciences of the United States of America, 86(12), 4771–4775. https://doi.org/https://doi.org/10.1073/pnas.86.12.4771
- Tai, T. C., Claycomb, R., Her, S., Bloom, A. K., & Wong, D. L. (2002). Glucocorticoid responsiveness of the rat phenylethanolamine N-methyltransferase gene. Molecular Pharmacology, 61(6), 1385–1392. https://doi.org/https://doi.org/10.1124/mol.61.6.1385
- Tarcic, N., Ovadia, H., Weiss, D. W., & Weidenfeld, J. (1998). Restraint stress-induced thymic involution and cell apoptosis are dependent on endogenous glucocorticoids. Journal of Neuroimmunology, 82(1), 40–46. https://doi.org/https://doi.org/10.1016/S0165-5728(97)00186-0
- Uchida, S., Nishida, A., Hara, K., Kamemoto, T., Suetsugi, M., Fujimoto, M., Watanuki, T., Wakabayashi, Y., Otsuki, K., McEwen, B. S., & Watanabe, Y. (2008). Characterization of the vulnerability to repeated stress in Fischer 344 rats: Possible involvement of microRNA-mediated down-regulation of the glucocorticoid receptor. The European Journal of Neuroscience, 27(9), 2250–2261. https://doi.org/https://doi.org/10.1111/j.1460-9568.2008.06218.x
- Ullian, M. E. (1999). The role of corticosteriods in the regulation of vascular tone. Cardiovascular Research, 41(1), 55–64. https://doi.org/https://doi.org/10.1016/S0008-6363(98)00230-2
- Ulrich-Lai, Y. M., & Herman, J. P. (2009). Neural regulation of endocrine and autonomic stress responses. Nature Reviews. Neuroscience, 10(6), 397–409. https://doi.org/https://doi.org/10.1038/nrn2647
- Ulrich-Lai, Y. M., Figueiredo, H. F., Ostrander, M. M., Choi, D. C., Engeland, W. C., & Herman, J. P. (2006). Chronic stress induces adrenal hyperplasia and hypertrophy in a subregion-specific manner. American Journal of Physiology-Endocrinology and Metabolism, 291(5), E965–E973. https://doi.org/https://doi.org/10.1152/ajpendo.00070.2006
- van Erp, A. M., Kruk, M. R., Meelis, W., & Willekens-Bramer, D. C. (1994). Effect of environmental stressors on time course, variability and form of self-grooming in the rat: Handling, social contact, defeat, novelty, restraint and fur moistening. Behavioural Brain Research, 65(1), 47–55. https://doi.org/https://doi.org/10.1016/0166-4328(94)90072-8
- Vavřínová, A., Behuliak, M., Bencze, M., Vodička, M., Ergang, P., Vaněčková, I., & Zicha, J. (2019). Sympathectomy-induced blood pressure reduction in adult normotensive and hypertensive rats is counteracted by enhanced cardiovascular sensitivity to vasoconstrictors. Hypertension Research: Official Journal of the Japanese Society of Hypertension, 42(12), 1872–1882. https://doi.org/https://doi.org/10.1038/s41440-019-0319-2
- Yagil, Y., & Krakoff, L. R. (1988). The differential effect of aldosterone and dexamethasone on pressor responses in adrenalectomized rats. Hypertension (Dallas, Texas: 1979), 11(2), 174–178. https://doi.org/https://doi.org/10.1161/01.HYP.11.2.174