Abstract
Pituitary adenylate cyclase-activating polypeptide (PACAP) is an excitatory neuromodulatory peptide strongly implicated in nervous stress processing. Human polymorphism of the primary PACAP receptor (PAC1) is linked to psychiatric disorders, including posttraumatic stress disorder (PTSD). Prefrontal cortex PACAP signaling is associated with processing of traumatic stress and fear learning, suggesting a potential role in PTSD-related deficits. We used RNAscope to define the cellular location of PACAP and PAC1 in the infralimbic cortex (IL). Subsequent experiments used a pharmacological approach to assess the impact of IL PACAP infusion on behavioral and physiological stress response and fear memory. Adult male Sprague-Dawley rats were bilaterally microinjected with PACAP (1 ug) or vehicle into the IL, 30 minutes prior to forced swim test (FST). Blood was sampled at 15, 30, 60, and 120 minutes for analysis of hypothalamic pituitary adrenal (HPA) axis reactivity. Five days after, animals were tested in a 3-day passive avoidance paradigm with subsequent testing of fear retention two weeks later. We observed that PACAP is highly expressed in putative pyramidal neurons (identified by VGlut1 expression), while PAC1 is enriched in interneurons (identified by GAD). Pretreatment with PACAP increased active coping style in the FST, despite higher levels of ACTH and corticosterone. The treatment was also sufficient to cause an increase in anxiety-like behavior in a dark/light crossover test and enhanced retention of passive avoidance. Our data suggest that IL PACAP plays a role in driving stress responses and in processing of fear memories, likely mediated by inhibition of cortical drive.
Introduction
Pituitary adenylate cyclase-activating polypeptide (PACAP) is an excitatory peptide neuromodulator that is heavily implicated in central nervous system stress processing (Hammack and May, Citation2015). Although widely expressed in the brain, PACAP is concentrated in stress-associated nuclei such as the hypothalamus, amygdala, bed nucleus of the stria terminalis (BST) and prefrontal cortex (PFC) (Cauvin et al., Citation1991). Recently, PACAP has received increasing attention for its role in central nervous system regulation of stress and anxiety (Hammack and May, Citation2015; Iemolo et al., Citation2016; Kirry et al., Citation2018; Lezak et al., Citation2014). A comprehensive behavioral test battery conducted in PACAP knockout (KO) mice revealed a unique phenotype characterized by increased novelty seeking and reduced anxiety-like behaviors (Hattori et al., Citation2012). Moreover, PACAP is directly implicated in hypothalamic pituitary adrenal (HPA) axis activation, as PACAP KO mice lack typical corticosterone elevation following various stressors (Lehmann et al., Citation2013; Morita et al., Citation2006).
The translational significance of PACAP appears to be considerable as polymorphisms of its receptor, PAC1, are associated with psychiatric disorders in humans, including post-traumatic stress disorder (Almli et al., Citation2013; Jovanovic et al., Citation2013; Ressler et al., Citation2011; Uddin et al., Citation2013; Wang et al., Citation2013). Genetic studies have identified a PAC1 polymorphism conferring risk for PTSD in women (Ressler et al., Citation2011). Moreover, circulating PACAP levels are positively correlated with PTSD symptoms, again in women (Ressler et al., Citation2011). One of the core symptoms of PTSD is inappropriate processing of fear memories, manifested as fear generalization and impaired extinction of conditioned fear processes, linked to impaired activation of the ventromedial prefrontal cortex. Studies in rodents reveal that central infusions of PACAP disrupt acquisition of fear conditioning, whereas prefrontal cortex infusion of a PAC1 antagonist disrupts cue learning in a trace conditioning paradigm (Kirry et al., Citation2018). These studies suggest that PACAP is involved in prefrontal processing of traumatic stress and fear learning, suggesting a potential role in PTSD-related deficits in emotional memory. Prior mapping studies indicate that both PACAP and PAC1 receptors are localized to the rodent ventromedial prefrontal cortex, including its infralimbic and prelimbic subdivisions (Hannibal, Citation2002; Lein et al., Citation2007), indicating the potential for PACAP action in circuitry regulating emotional memory. However, the cell types expressing PACAP and PAC1 are not known.
These studies were designed to delineate potential mechanisms of prefrontal PACAP signaling and assess the role of prefrontal cortical PACAP in control of behavioral and physiological responses to stressors. Our data indicate that ventromedial (infralimbic cortex (IL)) PFC infusions of PACAP enhance HPA axis stress responses and identify immediate and long-lasting impact of PACAP infusions on coping behavior and avoidance learning, effects that may be mediated via cortical interneurons.
Methods and materials
Animals
Male Sprague Dawley rats purchased from Envigo and housed in translucent polycarbonate shoebox cages with corncob bedding and food and water available ad libitum. Animals were standardly housed in pairs for the anatomical study of PACAP and PAC1 localization, and single-housed in the cannulation study to minimize the loss of cannula head caps. The colony room was temperature and humidity-controlled and maintained on a 12-h light-dark cycle (07:00 h lights on, 19:00 h lights off). Experiments were performed from 9:00 AM to 12:00 PM
All experiments complied with the National Institutes of Health Guidelines for the Care and Use of Animals and approved by the University of Cincinnati Institutional Animal Care and Use Committee.
RNAscope and immunohistochemistry
Rats (n = 6) were killed by rapid decapitation and brains were removed rapidly and frozen in cold isopentane (-45 °C). For the RNAscope procedure, flash frozen (-80 °C) rat brains were warmed to −20 °C for 1 h and sectioned at 18μm on a Microm cryostat. Brain slices were then mounted on RNase free (Fisher Superfrost Plus) slides and fixed (without air drying) in 4% paraformaldehyde/0.1M PBS for 20 min. The slides with sections were then rinsed with 0.1 M PBS and placed through an ascending series of ethanol, allowing the sections to dry after 100% ethanol. The slides were then permeabilized with the RNAscope Pretreatment Kit (Advanced Cell Diagnostics-Fluorescent Multiplex Reagent Kit-Cat # 320850) to unmask the target RNA. Sections were then hybridized to the target RNA probes complementary to PACAP (Rn- Adcyap 1-Cat # 400651), PAC1 (Rn- Adcyap1r1-Cat # 466981) and vesicular glutamate transporter 1 (VGLut1) (Rn- Slc17a7- Cat # 317001), a marker for glutamatergic cortical neurons. The sections were then processed for amplification using the RNAscope detection reagent (Advanced Cell Diagnostics-Fluorescent Multiplex Reagent Kit-Cat # 320850). The fluorescent markers used were as follows: Alexa-488 for PACAP and VGLut1, and Cy5 for PAC1 receptor. All steps were performed in accordance with the manufacturer Advanced Cell Diagnostics specifications.
Upon completion of the RNA scope procedure, the slides with the sections were processed for glutamic acid decarboxylase (GAD-67) or glial fibrilliary acid protein (GFAP) immunohistochemistry, to test co-expression of PACAP and PAC1 RNA in GABAergic neurons or astrocytes respectively. Brain sections on slides were rinsed 6 × 5 min in 0.1 M PBS and incubated in blocking solution (4% normal goat serum (NGS), 0.4% TritonX-100, 0.2% bovine serum albumin (BSA) in 0.1 M PBS, 1 h at room temperature (RT). Following the blocking step, the sections were incubated with GAD 67 antibody (Millipore Cat # MAB 5406) OR GFAP antibody (DAKO Cat # Z0334) diluted in blocking solution overnight at RT. The next day, sections were rinsed (3 × 5 min) in 0.1 M PBS at RT, followed by incubation with secondary antibody goat anti-mouse CY3 conjugated IgG (GAD 67) diluted 1:500 0.1 M PBS- 45 min or secondary antibody goat anti rabbit CY3 conjugated IgG (GFAP) diluted 1:500 in 0.1 M PBS- 45 min, rinsed 4 × 5 min in 0.1 M PBS, incubated with DAPI (ThermoFisher Cat #62248) diluted 1:1000 in 0.1 M PBS for 10 min, rinsed 4 × 5 min in 0.1 M PBS, coverslipped and viewed and images collected with the Nikon C2 confocal microscope. RNAscope reveals positive signal as discrete puncta. Using Nikon Elements Analysis object count software, the number of puncta/cell (per fluorescent channel), was determined as an estimate of relative expression level/cell type. For each animal, total number of puncta from 3 cells were counted bilaterally over 3–5 different fields across a given region. Cell and puncta counts were performed blinded to experimental grouping and used for further statistical analysis.
Surgical procedures
A second cohort of rats was used for behavioral assessments following local infusions of PACAP in the IL. Rats (n = 30) were anesthetized with isoflurane and treated with a prophylactic dose (2 mg/kg) of meloxicam (Loxicom®; Norbrook, Overland Park, KS). A stereotaxic instrument (David Kopf Instruments, Tujunga, CA) was used to guide placement of double barreled 26-gauge guide cannulas (Plastics One Inc., Roanoke, VA) into the left and right infralimbic cortices [from bregma: +3.0 mm anterior-posterior, ± 0.6 mm mediolateral, −4.6 dorsoventral] under aseptic conditions. Guide cannulas were fixed with dental cement (Stoelting Company) to 3 anchoring screws on the skull. Each cannula was fitted with a dummy cannula that extended 0.2 mm beyond the tip of the guide. Rats were treated with meloxicam for 2 days following surgery and allowed to recover for 2 weeks prior to behavioral studies. The locations of the cannula track were histologically verified at the end of experiments.
Drug injections
The PAC1 receptor agonist PACAP-38 was obtained from BACHEM (Torrence, CA) and dissolved in sterile saline on the day of injection (neutral pH was confirmed). Following surgery, cannulated rats were acclimated for 2 weeks to gentle handling and dummy cannula manipulation. On the day of behavioral studies, rats received a bilateral injection of 1 µg of PACAP-38 or vehicle (sterile saline, 0.5 µl/side) via a 33-gauge infusion cannula connected by polyethylene tubing to a 10 µl Hamilton micro-syringe (Hamilton Company) 30 min prior to a forced swim test (FST). This dose of PACAP was chosen because it has been shown previously to be behaviorally active upon intraparenchymal infusion in rats (Lezak et al., Citation2014; Telegdy and Kokavszky, Citation2000). The intra-IL infusion was delivered using a syringe pump (Harvard Apparatus) at a rate of 0.16 µl/min and the injectors were left in place for 60 s after injection to allow for diffusion away from the cannula. The total process of manipulation of the animal was kept at a minimum (5–6 min) and each animal was handled consistently to avoid introducing significant disturbances that could confound the effects. Following injection, rats were returned to their home cage until behavioral studies commenced.
Forced Swim Test/HPA axis testing
This experiment tested the hypothesis that intra-IL pretreatment with PACAP would modify coping strategy and enhance physiological reactivity to forced swim. Rats received a pretreatment of either PACAP or vehicle 30 min prior to the onset of the FST. Behaviors were recorded and scored. Rats underwent a modified version of the forced swim test that consisted of a single 10 min session of swimming in a transparent, cylindrical container filled with ambient temperature water (25 ± 2 °C). Using a time-sampling technique, behavior was sampled every 5 s and recorded and coded as ‘climbing’, ‘swimming’, ‘diving’ or ‘immobile’ using 12 coded observations per minute (Slattery and Cryan, Citation2012). Climbing is defined as quick movements of the forelimbs that break the surface of the water; swimming is defined as paddling movement of fore- and hind limbs; diving as whole-body movement toward the bottom of the swim tank; immobility is defined as floating with the absence of movement except for those required to keep the nose above water (Yankelevitch-Yahav et al., Citation2015). Blood was sampled by tail bleed 15, 30, 60, and 120 min from the commencement of FST for corticosterone and ACTH analysis.
Corticosterone and ACTH radioimmunoassay
250 µl of blood was obtained by tail nick, collected within 2 min from the initial handling of the rat under light manual restraint. Subsequent samples were obtained by gently removing the clot to reinitiate bleeding. All samples were collected into tubes containing EDTA. Plasma was separated by centrifugation in 4 °C at 6000 rpm for 15 min and stored at −20 °C until assay preparation. Plasma corticosterone concentrations were determined using 125I radioimmunoassay kits (MP Biomedicals). Plasma ACTH concentrations were determined by radioimmunoassay using a specific antiserum generously donated by Dr. William Engeland (University of Minnesota, Minneapolis) at a dilution of 1:120,000, with 125I ACTH (GE Healthcare) as a labeled tracer following protocol from (Ulrich-Lai et al., Citation2006). All samples were run in duplicate within the same assay.
Passive avoidance
As PACAP is thought to play a role in lasting emotional dysregulation seen in PTSD we subsequently tested the ability of IL PACAP administration in the context of a stressor to modulate emotional memory later in time. Five days after FST, rats were tested in a passive avoidance paradigm to investigate long-term effects of PACAP treatment on stress-enhanced fear learning (Blouin et al., Citation2016). The passive avoidance GEMINI Active and Passive Avoidance System (San Diego Instruments, USA) consisted of two equal size chambers (24 cm (W) × 20 cm (D) × 20 cm (H)) separated by a guillotine door. One side of the chamber was illuminated, while the other remained dark. On habituation day, the rat was placed into the illuminated chamber. After 30 s, the guillotine door was raised, and the rat was able to explore for 120 s. Latency to cross into the dark chamber and number of crosses between chambers was recorded. Training was initiated the next day (24 h later approx.) and consisted of a 30 s habituation to the illuminated chamber before the guillotine door was raised. Once the rat crossed to the dark chamber the guillotine door was closed and the rat received a 0.25 mA shock for 1 s. The rat remained in the dark chamber for 10 s after the shock. Testing occurred 24 h later and the parameters were identical to training except no shock was delivered and a maximum of 300 s was allotted for latency to cross to the dark chamber. Finally, a retention test identical to the original test day was given 2 weeks later.
Fos induction by IL PACAP infusion
Following a 3-week washout period, we performed a crossover design in which rats previously treated with vehicle received PACAP, and vice versa, 60 minutes prior to euthanasia. Rats were administered an overdose of sodium pentobarbital and subsequently transcardially perfused with 0.1 M phosphate buffer (PBS) followed by 4% paraformaldehyde in 0.1 M PBS pH 7.4. Brains were post-fixed in 4% paraformaldehyde at 4 °C for 24 h, then transferred to 30% sucrose in 0.1 MPBS at 4 °C until the moment of processing the tissue. Brains were sliced into serial 35 μm coronal sections using a freezing microtome (−20 °C). Sections (1/6) were collected into wells containing cryoprotectant solution (30% Sucrose, 1% Polyvinyl-pyrolidone (PVP-40), and 30% Ethylene glycol, in 0.1 M PBS). Immunohistochemistry: sections were washed 6 × 5 min in 0.01 M PBS at RT. Immediately after, sections were incubated with 1% Sodium borohydride in 0.1 MPBS for 30 min at RT. After rinsing 6 × 5 min 0.1 M PBS, they were incubated in 3% hydrogen peroxide in 0.1 M PBS for 20 min. Subsequently, brain slices were rinsed 6 × 5 min and 4 × 15 min in 0.1 M PBS and then incubated in blocking solution (4% normal goat serum (NGS), 0.4% TritonX-100, 0.2% bovine serum albumin (BSA) in 0.1 M PBS, 2 h, at RT. Sections were then incubated with Fos rabbit polyclonal antibody (1:1000, Santa Cruz, sc-52) in blocking solution, overnight at RT. The next day, sections were rinsed (3 × 5 min) in 0.1 M PBS at RT, followed by incubation with secondary antibody (biotinylated goat anti-rabbit, (1:400; Vector Laboratories, BA1000) in blocking solution at RT for 1 h. Sections were again rinsed (3 × 5 min) in 0.1 M PBS and then reacted with avidin-biotin horseradish peroxidase complex (1:800 in 0.1 M PBS; Vector Laboratories) for 1 h at RT. Sections were then rinsed (3 × 5 min) in 0.1 M PBS and then developed with a 8 min incubation in DAB-Nickel solution: 10 mg 3,3′-diaminobenzidine (DAB) tablet (Sigma, DF905), 0.5 ml of a 2% aqueous nickel sulfate solution, 20ul of 30% hydrogen peroxide in 50 ml of 0.1 M PBS. Sections were finally washed in PBS, mounted on Superfrost slides (Fisherbrand, Fisher), allowed to dry, dehydrated with xylene, and then coverslipped in DPX mounting medium (Sigma). Fos immunoreactive nuclei were quantified in the IL and in regions known to be downstream of the IL and/or to participate in stress-regulatory process. The regions of interest included the PVN (parvocellular PVN: pPVN, dorsal pPVN, lateral pPVN, ventral zone of the medial pPVN; magnocellular PVN mPVN: anterior mPVN), basolateral amygdala, and central amygdaloid nucleus (Paxinos and Watson, Citation2007). The number of Fos positive nuclei was counted with a semi-automated method using ImageJ software (National Institutes of Health, Bethesda, MD) with the guidance of a rat brain atlas. The mean value of 2–3 sections through a given region bilaterally (4–6 individual measurements) was calculated for each rat and used for subsequent statistical analysis. Cell counts were performed blind to experimental grouping. The correct placement of the cannulas was determined by images taken during the process of slicing and they were represented on a modified figure from the Paxinos & Watson, 1998 using PowerPoint, Microsoft 365.
Statistics
Student t-test (two-tailed) was used to compare behavioral measures in the forced swim test, RNAscope PACAP and PAC1 counts, the response latency in passive avoidance learning, and Fos expression between groups. The Welch’s correction was used in case of non-homogeneous variance (verified by F test). Where warranted (e.g. failure of Shapiro-Wilk’s test for normality of residuals), non-parametric analysis of the data was performed using a two-tailed Mann–Whitney U test. A two-way repeated measures ANOVA was used to compare CORT and ACTH response curves between PACAP-treated and vehicle-treated groups. In case of interaction of the factors, the Sidak post hoc test was used to correct for multiple comparisons. Data were analyzed using GraphPad Prism version 8.4.3 for Windows, GraphPad Software, San Diego, California USA, www.graphpad.com.
Results
Anatomy
Anatomical studies confirmed rich localization of both PACAP and PAC1 mRNAs in the IL (). Co-localization studies indicate differential localization of PACAP and its primary receptor, with peptide mRNA predominantly localized in glutamatergic (putatively pyramidal) neurons (U = 212, n1 = n2 = 28, median PACAP = 26 median PAC1 = 17.5, p = 0.003) () In contrast, PAC1 mRNA was highly enriched in in GAD-positive cells (presumptive interneurons) (U = 3, n1 = n2 = 11, median PACAP = 8 median PAC1 = 60, p < 0.0001) (). Quantification of RNAscope puncta also revealed a significantly higher PACAP/PAC1 expression ratio in vGlut1 neurons relative to GAD67 neurons, confirming differential localization (U = 19, n1 = 28, n2 = 11, median Vglut1 = 1.559 median GAD67 = 0.075, p < 0.0001) (). Neither PACAP nor PAC1 were detected in GFAP-positive cells in this region ().
Figure 1. Distribution of PACAP and PAC1 mRNA expression in infralimbic (IL) neurons using RNAscope. (A) Cellular labeling of PACAP (upper arrow) and PAC1 (lower arrows) is largely distinct, with occasional cells showing colocalization (upper arrow). (B) A large population of projection neurons expresses PACAP (arrows). (C) PAC1 is expressed in both vGluT1-positive (upper left arrow) and vGluT1 negative (lower left arrow) neurons. A sizable population of vGluT1 neurons are PAC1 negative (right arrows). (D) GABAergic (GAD67) interneurons can express mostly PACAP (upper left inset) or PAC1 (lower left inset) alone or coexpress PACAP and PAC1 (lower right arrow). (E). While astrocyte processes (GFAP) are in proximity to both PACAP (third from bottom) and PAC1 ( white upper arrows)-containing cells, there is no evidence for colocalization in GFAP-positive cell bodies (lower arrows). (F) Preferential expression of PACAP vs. PAC1 mRNA in glutamatergic (vGluT1 positive) neurons in the IL, assessed by particle counts in vGlutT1 mRNA expressing neurons as defined from underlying nuclear stain (DAPI). (G) Preferential expression of PAC1 vs. PACAP mRNA in GABAergic IL neurons (GAD67 immunopositive). Note undetectable expression of PACAP in the majority of GAD67 neurons. (H) PACAP/PAC1 expression ratio in vGlut1 neurons relative to GAD67 highlights differential expression patterns across cell populations Data as Mean ± SEM. **p < 0.005, ***p < 0.0001.
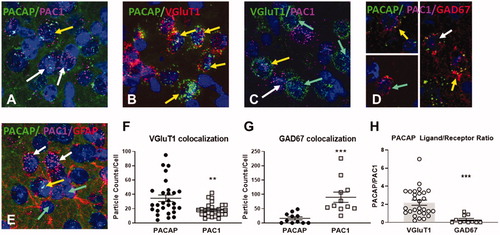
Anatomical verification of cannulas implantation
Only animals with both cannulas correctly hitting the IL () were included in the PACAP infusion study, resulting in final n’s of 12 for the PACAP group and 10 for the saline group.
Forced swim test/HPA axis response
Intra-IL administration of PACAP prior to testing in the FST caused a reorganization of coping behavior. While immobility time was not affected by acute PACAP, drug treated animals spent less time swimming (t(13.96)=2.409, p = 0.03) and more time diving (t(20)=2.692, p = 0.01) and performed more climbing in the initial minute of the test (PACAP × Time F(3,60)=3.303, p = 0.027; Time F(3,60)=24.67, p < 0.0001; Sidak’s test p < 0.05), overall consistent with an active behavioral coping response . The ACTH response to swim stress was enhanced following PACAP infusion (PACAP F(1,20)=13.55, p = 0.0015; time F(3,60)=86,84, p < 0.0001; PACAP × time F(3,60)=4.492, p = 0.0066), with elevations evident at 15 (p < 0.05) and 30 min (p < 0.05). Furthermore, the overall corticosterone response to the FST was not affected by PACAP, but there was a significant effect of time and a PACAP by time interaction (PACAP F(1,20)= 0.677, p = 0.4203; time F(3,60)=122.1, p < 0.0001; PACAP × time F(3,60)=4.867, p = 0.0043), with higher concentration of corticosterone relative to vehicle at the 15 min time point (p < 0.05) ().
Figure 3. Behavioral and HPA axis responses to forced swim test after PACAP or vehicle infusions into the IL (A–D). While overall immobility was not affected by PACAP (A), active behaviors such as swimming (B), climbing (C), and diving (D) were affected in PACAP treated animals. Blood samples were taken following completion of the FST for ACTH and CORT determinations. ACTH was significantly increased in PACAP-treated animals relative to controls at early post-FST time points, whereas corticosterone secretion was slightly increased only at the first post-stress time point and the overall corticosterone response was not affected. Data as Mean ± SEM. *p < 0.05.
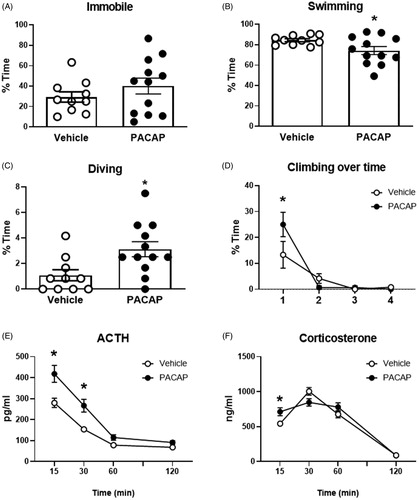
Passive Avoidance
To test possible lasting effects of PACAP on stress processing, we assessed the role of prior stress/PACAP exposure on passive avoidance, a test of emotional memory, 5 days after the acute stress session. We first assessed the latency to cross to the dark chamber during a free exploration habituation session, as a measurement of anxiety-like behavior. We observed that animals that were treated with PACAP during the previous acute stress (FST) 3 days prior decreased cross-over latency (t(18)=2.639, p = 0.0167), consistent with an enhanced anxiety-like phenotype. Although there were no differences between both groups during the training and test phase, 2 weeks later we observed a significant increase in latency to cross during the memory retention test in animals that had received IL PACAP infusion during FST (t(14.46)=2.261, p = 0.0397) (). These data suggest that exposure to drug prior to FST testing had a lasting effect on the response of the animal to a later aversive stimulus, consistent with synergistic actions of PACAP and stress on later behavioral performance.
Figure 4. Passive avoidance behavior tested 5 days after FST exposure in PACAP and vehicle pretreated animals. (A) PACAP-treated rats were quicker to cross over to the dark chamber of the apparatus on the habituation trial. (B) Prior PACAP exposure did not affect cross-over latency on the training trial, nor during testing phase, but significantly enhanced retention of avoidance behavior when tested 2 weeks later (C), suggestive of enhanced memory of the aversive experience. Note that mechanical issues with the apparatus resulted in exclusion of data from three animals (2 vehicle, 1 PACAP). Data as Mean ± SEM. *p < 0.05.
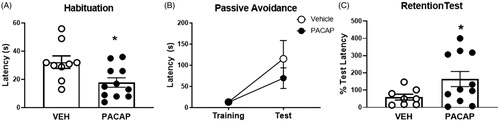
Fos expression
Fos protein is rapidly induced upon cellular stimulation and is used as a marker of recent neuronal activation. IL infusions of PACAP significantly increased the number of Fos-positive nuclei in the PVN (t(9)=2.297, p = 0.0472) commensurate with HPA axis activation. In contrast, PACAP infusion did not alter Fos expression in the IL, basolateral amygdala or central amygdaloid nucleus ().
Figure 5. Expression of Fos following acute infusion of PACAP into the IL of unstressed rats. PACAP infusion increased the number of PVN Fos immunoreactive neurons after IL infusion, but did not affect overall expression in the IL or downstream targeted in the central and lateral divisions of the central amygdaloid nucleus and the basolateral amygdala. Data as Mean ± SEM. *p < 0.05.
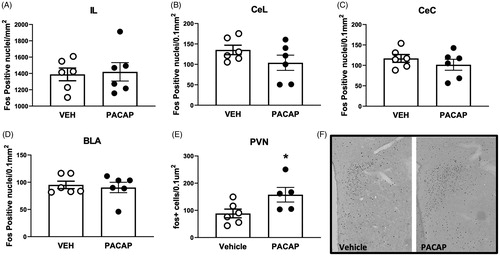
Discussion
The results of our study support an important role for prefrontal (IL) PACAP in regulating physiological and behavioral responses to stress. Single pretest IL infusion of PACAP had immediate effects on active coping (but not immobility) in the FST and was sufficient to increase anxiety-like behavior upon dark/light testing 5 days later and to enhance long-term retention of avoidance behavior. Enriched localization of PAC1 receptors to GABAergic neurons suggest that actions of PACAP may be mediated by inhibition of cortical projection neurons. Together, the data suggests that IL PACAP plays a role in driving stress responses and in long-term processing of fear memories, likely mediated by inhibition of cortical pyramidal neurons.
Our anatomical data suggest differential localization of PACAP and PAC1 producing neurons in the IL, with PACAP preferentially localized to glutamatergic cell populations and PAC1 to interneurons. Given that PAC1 is strongly linked to Gs activation (Liao et al., Citation2019; May et al., Citation2010; Spengler et al., Citation1993), these data suggest that exogenous PACAP will predominantly excite IL interneurons and thereby inhibit IL output. This hypothesis is consistent with the lack of observable increase in Fos activation in the IL following PACAP infusion, as interneuron activation would not be expected to result in pronounced enhancement of net cortical Fos expression (interneurons represent a small proportion (<20%) of cortical neurons). In addition, the marked increase in PVN Fos induction by acute PACAP infusion is consistent with trans-synaptic removal of IL inhibition, and is in keeping with the known role of the IL in HPA axis inhibition (Myers et al., Citation2017). Further studies to test this hypothesis require direct measures of neuronal activity, such as electrophysiological recordings of intrinsic excitability of GABAergic interneurons and inhibitory synaptic drive onto projection neurons in the IL following PACAP application.
The behavioral profile exhibited in the FST suggests that PACAP initiates an active coping response (Commons et al., Citation2017; Molendijk and de Kloet, 2019). While the interpretation of the FST as a test of depression is open to question, it affords the ability to assess changes in behavior in the stressful context of the test (Molendijk and de Kloet, 2019). Our data are of interest in this regard, as diving and increased climbing behavior may reflect a redirection of strategy from acclimation (floating) to escape or enhanced locomotion. Moreover, diving is a relatively rare behavioral observation in the FST (Bourke and Neigh, Citation2011; Slattery and Cryan, Citation2012), again suggesting that PACAP significantly modifies behavioral reactivity in this context. Increased active behavior is accompanied by enhanced ACTH release, which in combination with the active coping data suggests that IL PACAP enhances the negative impact of the swim procedure on the individual. Amplification of stress reactivity is supported by enhanced PVN activation following IL PACAP infusion.
The lasting effects of IL PACAP on passive avoidance latencies is further consistent with amplification of the significance of the FST experience to the organism (although it cannot be discarded that part of the effects were due to long-lasting effects of the drug itself). It is known that prior exposure to aversive stimuli can enhance emotional responses to subsequent stressors, a well-described phenomenon termed stress-enhanced fear learning (Blouin et al., Citation2016). The memory enhancing effects of discrete stress exposure contrast with impaired avoidance learning in rats receiving chronic stress exposure (restraint) (Radahmadi et al., Citation2012; Trnecková et al., Citation2005), suggesting that stressor intensity and chronicity may play different roles in processes regulating avoidance responding. Notably, enhanced avoidance can also be observed following glucocorticoid administration before or briefly after training enhance avoidance (Fornari et al., Citation2012; Roozendaal, Citation2002; Schwabe et al., Citation2012), suggesting that the observed increase in corticosteroid signaling may participate in memory-enhancing effects of PACAP following swim stress. In our study, we observed that PACAP evoked an exaggeration of remote but not 24-h avoidance retention in animals that were trained several days after being stressed, indicating that IL PACAP in the context of stress may participate in sensitizing the neuronal or endocrine signaling in response to subsequent stress and enhance the long-term consolidation of the inhibitory response in the passive avoidance task. The fact that the animals also showed increased anxiety-like behavior during the habituation session supports lingering sensitization of behavioral responses related to stress in the context of IL PACAP infusions.
Prior studies have addressed a role for PACAP and PAC1 receptors in fear conditioning paradigms. A recent study notes that infusions of a PAC1 receptor antagonist into the neighboring prelimbic cortex can impair cued conditioning in females (but not males), suggesting a role for endogenous PACAP in fear memory (Kirry et al., Citation2018). Though conducted in another prefrontal region, the data are consistent with our observations linking PACAP signaling to enhanced fear memory, which once again may relate to processes underlying latent effects seen in PTSD and PTSD models. Additional studies support complex effects of intracerebroventricular PACAP infusion on contextual fear responses: freezing was significantly blocked one day following PACAP and training, but gradually increased on subsequent days, exceeding that of controls by 7 days post acquisition (Meloni et al., Citation2016). The latter finding is further concordant with a role for central PACAP in incubation of stressful experiences.
Prior studies indicate that acute icv PACAP infusions may have effects on behavior in the context of a novel open field, increasing active behaviors including locomotion, rearing and grooming (Adamik and Telegdy, Citation2004). Thus, icv PACAP may promote active and exploratory behaviors, which may underscore our observation of increased active coping behavior (including an atypical diving response) in the FST. Indeed, it is plausible that PACAP is driving atypical behavioral responses that are encoded along with aversive information associated with stressors (swim and as well as shock experience, in the case of fear conditioning).
The organization of IL PACAP and PAC1 expression favors a role for PACAP in driving cortical interneurons. It is possible that endogenous PACAP may access PAC1 receptors via axons of neurons in the immediate vicinity of the IL or from corticocortical projections from other PFC regions, which are known to be extensively interconnected (Dembrow and Johnston, Citation2014; Zingg et al., Citation2014). This would suggest a putative role in feedback inhibition of pyramidal cell output, with PACAP released upon depolarization then activating PAC1-receptive interneurons. Conversely, PACAP is also localized in subcortical afferents innervating the IL such as the hypothalamus, amygdala and paraventricular thalamic nuclei (Hannibal, Citation2002), and may thus act to enforce extrinsic inhibition of the IL.
While PAC1 is the high-affinity PACAP receptor, PACAP (albeit at lower affinity) can also bind to the vasoactive intestinal polypeptide receptors VPAC1 and VPAC2 (Harmar et al., Citation2012; Ramos-Álvarez et al., Citation2015), which are also expressed in the IL (Joo et al., Citation2004). In particular, these receptors appear to be involved in PACAP signaling in astrocytes, which can influence subsequent cortical excitability (Kong et al., Citation2016; Zusev and Gozes, Citation2004). It is notable that we do not observe significant expression of PAC1 in IL astrocytes, suggesting that any actions on astrocytes would be mediated by actions at VPAC1 or VPAC2.
These experiments did not assess the role of IL PACAP in females. Elevated circulating PACAP and PAC1 gain-of-function SNPs are linked to PTSD in women, suggesting that the peptide may be particularly important in females (Mercer et al., Citation2016; Ressler et al., Citation2011). This is supported by evidence suggesting the existence of a functional SNP in a putative estrogen response element of the ADCYAP1R1 (PAC1) gene (Mercer et al., Citation2016), suggesting a possible mechanism for sex-specific PTSD susceptibility in individuals carrying the risk allele. There is also evidence to suggest that effects of prefrontal PAC1 antagonists on cued conditioning are seen in female but not male rats (Kirry et al., Citation2018). Thus, while strong behavioral effects were observed in males, it is possible that PACAP actions may be more pronounced in females, which will be a future direction for this research.
Overall, our data support a role for prefrontal PACAP in modulating behavioral responses to acute stress and potentiating emotional memory in an aversive context later in time. The latter finding supports the hypothesis that PACAP enhances the impact of an acute stress on later fear reactivity, a property that may be of relevance to stimulus generalization and/or extinction deficits seen in PTSD. Future work is required to determine the specific effects of PACAP at the local circuit level, and also to test the isolated persisting effects of PACAP in the absence of stress, and whether blockade of PACAP signaling may help ameliorate the lasting impact of stress exposure.
Acknowledgements
The authors thank the Herman lab staff for assistance in data collection and study conduct.
Disclosure statement
The authors declare that the research was conducted in the absence of any commercial or financial relationships that could be construed as a potential conflict of interest.
Additional information
Funding
References
- Adamik, A., & Telegdy, G. (2004). Involvement of different receptors in pituitary adenylate cyclase activating polypeptide induced open field activity in rats. Neuropeptides, 38(1), 16–20. https://doi.org/10.1016/j.npep.2003.11.001
- Almli, L. M., Mercer, K. B., Kerley, K., Feng, H., Bradley, B., Conneely, K. N., & Ressler, K. J. (2013). ADCYAP1R1 genotype associates with post-traumatic stress symptoms in highly traumatized African-American females. American Journal of Medical Genetics Part B: Neuropsychiatric Genetics, 162(3), 262–272. https://doi.org/10.1002/ajmg.b.32145
- Blouin, A. M., Sillivan, S. E., Joseph, N. F., & Miller, C. A. (2016). The potential of epigenetics in stress-enhanced fear learning models of PTSD. Learning & Memory, 23(10), 576–586. https://doi.org/10.1101/lm.040485.115
- Bourke, C. H., & Neigh, G. N. (2011). Behavioral effects of chronic adolescent stress are sustained and sexually dimorphic. Hormones and Behavior, 60(1), 112–120. https://doi.org/10.1016/j.yhbeh.2011.03.011
- Cauvin, A., Robberecht, P., De Neef, P., Gourlet, P., Vandermeers, A., Vandermeers-Piret, M. C., & Christophe, J. (1991). Properties and distribution of receptors for pituitary adenylate cyclase activating peptide (PACAP) in rat brain and spinal cord. Regulatory Peptides, 35(2), 161–173. https://doi.org/10.1016/0167-0115(91)90478-y
- Commons, K. G., Cholanians, A. B., Babb, J. A., & Ehlinger, D. G. (2017). The Rodent Forced Swim Test measures stress-coping strategy, not depression-like behavior. ACS Chemical Neuroscience, 8(5), 955–960. https://doi.org/10.1021/acschemneuro.7b00042
- Dembrow, N., & Johnston, D. (2014). Subcircuit-specific neuromodulation in the prefrontal cortex. Frontiers in Neural Circuits, 8, 54. https://doi.org/10.3389/fncir.2014.00054
- Fornari, R. V., Wichmann, R., Atucha, E., Desprez, T., Eggens-Meijer, E., & Roozendaal, B. (2012). Involvement of the insular cortex in regulating glucocorticoid effects on memory consolidation of inhibitory avoidance training. Frontiers in Behavioral Neuroscience, 6, 10. https://doi.org/10.3389/fnbeh.2012.00010
- Hammack, S. E., & May, V. (2015). Pituitary adenylate cyclase activating polypeptide in stress-related disorders: data convergence from animal and human studies. Biological Psychiatry, 78(3), 167–177. https://doi.org/10.1016/j.biopsych.2014.12.003
- Hannibal, J. (2002). Pituitary adenylate cyclase-activating peptide in the rat central nervous system: An immunohistochemical and in situ hybridization study. Journal of Comparative Neurology, 453(4), 389–417. https://doi.org/10.1002/cne.10418
- Harmar, A. J., Fahrenkrug, J., Gozes, I., Laburthe, M., May, V., Pisegna, J. R., Vaudry, D., Vaudry, H., Waschek, J. A., & Said, S. I. (2012). Pharmacology and functions of receptors for vasoactive intestinal peptide and pituitary adenylate cyclase-activating polypeptide: IUPHAR review 1. British Journal of Pharmacology, 166(1), 4–17. https://doi.org/10.1111/j.1476-5381.2012.01871.x
- Hattori, S., Takao, K., Tanda, K., Toyama, K., Shintani, N., Baba, A., Hashimoto, H., & Miyakawa, T. (2012). Comprehensive behavioral analysis of pituitary adenylate cyclase-activating polypeptide (PACAP) knockout mice. Frontiers in Behavioral Neuroscience, 6, 1–18.
- Iemolo, A., Seiglie, M., Blasio, A., Cottone, P., & Sabino, V. (2016). Pituitary adenylate cyclase-activating polypeptide (PACAP) in the central nucleus of the amygdala induces anxiety via melanocortin receptors. Psychopharmacology, 233(17), 3269–3277. https://doi.org/10.1007/s00213-016-4366-y
- Joo, K. M., Chung, Y. H., Kim, M. K., Nam, R. H., Lee, B. L., Lee, K. H., & Cha, C. I. (2004). Distribution of vasoactive intestinal peptide and pituitary adenylate cyclase-activating polypeptide receptors (VPAC1, VPAC2, and PAC1 receptor) in the rat brain. The Journal of Comparative Neurology, 476(4), 388–413. https://doi.org/10.1002/cne.20231
- Jovanovic, T., Norrholm, S. D., Davis, J., Mercer, K. B., Almli, L., Nelson, A., Cross, D., Smith, A., Ressler, K. J., & Bradley, B. (2013). PAC1 receptor (ADCYAP1R1) genotype is associated with dark-enhanced startle in children. Molecular Psychiatry, 18(7), 742–743. https://doi.org/10.1038/mp.2012.98
- Kirry, A. J., Herbst, M. R., Poirier, S. E., Maskeri, M. M., Rothwell, A. C., Twining, R. C., & Gilmartin, M. R. (2018). Pituitary adenylate cyclase-activating polypeptide (PACAP) signaling in the prefrontal cortex modulates cued fear learning, but not spatial working memory, in female rats. Neuropharmacology, 133, 145–154. https://doi.org/10.1016/j.neuropharm.2018.01.010
- Kong, L., Albano, R., Madayag, A., Raddatz, N., Mantsch, J. R., Choi, S. J., Lobner, D., & Baker, D. A. (2016). Pituitary Adenylate cyclase-activating polypeptide orchestrates neuronal regulation of the astrocytic glutamate-releasing mechanism system xc (.). Journal of Neurochemistry, 137(3), 384–393. https://doi.org/10.1111/jnc.13566
- Lehmann, M. L., Mustafa, T., Eiden, A. M., Herkenham, M., & Eiden, L. E. (2013). PACAP-deficient mice show attenuated corticosterone secretion and fail to develop depressive behavior during chronic social defeat stress. Psychoneuroendocrinology, 38(5), 702–715. https://doi.org/10.1016/j.psyneuen.2012.09.006
- Lein, E. S., Hawrylycz, M. J., Ao, N., Ayres, M., Bensinger, A., Bernard, A., Boe, A. F., Boguski, M. S., Brockway, K. S., Byrnes, E. J., Chen, L., Chen, L., Chen, T.-M., Chin, M. C., Chong, J., Crook, B. E., Czaplinska, A., Dang, C. N., Datta, S., … Jones, A. R. (2007). Genome-wide atlas of gene expression in the adult mouse brain. Nature, 445(7124), 168–176. https://doi.org/10.1038/nature05453
- Lezak, K. R., Roelke, E., Harris, O. M., Choi, I., Edwards, S., Gick, N., Cocchiaro, G., Missig, G., Roman, C. W., Braas, K. M., Toufexis, D. J., May, V., & Hammack, S. E. (2014). Pituitary adenylate cyclase-activating polypeptide (PACAP) in the bed nucleus of the stria terminalis (BNST) increases corticosterone in male and female rats. Psychoneuroendocrinology, 45, 11–20. https://doi.org/10.1016/j.psyneuen.2014.03.007
- Liao, C., de Molliens, M. P., Schneebeli, S. T., Brewer, M., Song, G., Chatenet, D., Braas, K. M., May, V., & Li, J. (2019). Targeting the PAC1 receptor for neurological and metabolic disorders. Current Topics in Medicinal Chemistry, 19(16), 1399–1417. https://doi.org/10.2174/1568026619666190709092647
- May, V., Lutz, E., MacKenzie, C., Schutz, K. C., Dozark, K., & Braas, K. M. (2010). Pituitary adenylate cyclase-activating polypeptide (PACAP)/PAC1HOP1 receptor activation coordinates multiple neurotrophic signaling pathways: Akt activation through phosphatidylinositol 3-kinase gamma and vesicle endocytosis for neuronal survival. The Journal of Biological Chemistry, 285(13), 9749–9761. https://doi.org/10.1074/jbc.M109.043117
- Meloni, E. G., Venkataraman, A., Donahue, R. J., & Carlezon, W. A. (2016). Bi-directional effects of pituitary adenylate cyclase-activating polypeptide (PACAP) on fear-related behavior and c-Fos expression after fear conditioning in rats. Psychoneuroendocrinology, 64, 12–21. https://doi.org/10.1016/j.psyneuen.2015.11.003
- Mercer, K. B., Dias, B., Shafer, D., Maddox, S. A., Mulle, J. G., Hu, P., Walton, J., & Ressler, K. J. (2016). Functional evaluation of a PTSD-associated genetic variant: Estradiol regulation and ADCYAP1R1. Translational Psychiatry, 6(12), e978. https://doi.org/10.1038/tp.2016.241
- Molendijk, M. L., & de Kloet, E. R. (2019). Coping with the forced swim stressor: Current state-of-the-art. Behavioural Brain Research, 364, 1–10. https://doi.org/10.1016/j.bbr.2019.02.005
- Morita, Y., Yanagida, D., Shintani, N., Ogita, K., Nishiyama, N., Tsuchida, R., Hashimoto, H., & Baba, A. (2006). Lack of trimethyltin (TMT)-induced elevation of plasma corticosterone in PACAP-deficient mice. Annals of the New York Academy of Sciences, 1070, 450–456. https://doi.org/10.1196/annals.1317.060
- Myers, B., McKlveen, J. M., Morano, R., Ulrich-Lai, Y. M., Solomon, M. B., Wilson, S. P., & Herman, J. P. (2017). Vesicular glutamate transporter 1 knockdown in infralimbic prefrontal cortex augments neuroendocrine responses to chronic stress in male rats. Endocrinology, 158(10), 3579–3591. https://doi.org/10.1210/en.2017-00426
- Paxinos, G., & Watson, C. (1998). The rat brain in stereotaxic coordinates (Fourth Edition). Academic Press.
- Paxinos, G., & Watson, C. (2007). The rat brain in stereotaxic coordinates (Sixth Edition). Elsevier.
- Radahmadi, M., Alaei, H., Sharifi, M. R., & Hosseini, N. (2012). The effect of synchronized forced running with chronic stress on short, mid and long- term memory in rats. Asian Journal of Sports Medicine, 4(1), 54–62. https://doi.org/10.5812/asjsm.34532
- Ramos-Álvarez, I., Mantey, S. A., Nakamura, T., Nuche-Berenguer, B., Moreno, P., Moody, T. W., Maderdrut, J. L., Coy, D. H., & Jensen, R. T. (2015). A structure-function study of PACAP using conformationally restricted analogs: Identification of PAC1 receptor-selective PACAP agonists. Peptides, 66, 26–42. https://doi.org/10.1016/j.peptides.2015.01.009
- Ressler, K. J., Mercer, K. B., Bradley, B., Jovanovic, T., Mahan, A., Kerley, K., Norrholm, S. D., Kilaru, V., Smith, A. K., Myers, A. J., Ramirez, M., Engel, A., Hammack, S. E., Toufexis, D., Braas, K. M., Binder, E. B., & May, V. (2011). Post-traumatic stress disorder is associated with PACAP and the PAC1 receptor. Nature, 470(7335), 492–497. https://doi.org/10.1038/nature09856
- Roozendaal, B. (2002). Stress and memory: opposing effects of glucocorticoids on memory consolidation and memory retrieval. Neurobiology of Learning and Memory, 78(3), 578–595. https://doi.org/10.1006/nlme.2002.4080
- Schwabe, L., Joëls, M., Roozendaal, B., Wolf, O. T., & Oitzl, M. S. (2012). Stress effects on memory: An update and integration. Neuroscience & Biobehavioral Reviews.
- Slattery, D. A., & Cryan, J. F. (2012). Using the rat forced swim test to assess antidepressant-like activity in rodents. Nature Protocols, 7(6), 1009–1014. https://doi.org/10.1038/nprot.2012.044
- Spengler, D., Waeber, C., Pantaloni, C., Holsboer, F., Bockaert, J., Seeburg, P. H., & Journot, L. (1993). Differential signal transduction by five splice variants of the PACAP receptor. Nature, 365(6442), 170–175. https://doi.org/10.1038/365170a0
- Telegdy, G., & Kokavszky, K. (2000). The action of pituitary adenylate cyclase activating polypeptide (PACAP) on passive avoidance learning. The role of transmitters. Brain Research, 874(2), 194–199. https://doi.org/10.1016/s0006-8993(00)02579-8
- Trnecková, L., Hynie, S., Sída, P., Hlinák, Z., Krejcí, I., & Klenerová, V. (2005). Effects of stress and of amphetamine on passive avoidance conditioning in rats. General Physiology and Biophysics, 24(1), 129–142.
- Uddin, M., Chang, S. C., Zhang, C., Ressler, K., Mercer, K. B., Galea, S., Keyes, K. M., McLaughlin, K. A., Wildman, D. E., Aiello, A. E., & Koenen, K. C. (2013). ADCYAP1R1 genotype, posttraumatic stress disorder, and depression among women exposed to childhood maltreatment. Depression and Anxiety, 30(3), 251–258. https://doi.org/10.1002/da.22037
- Ulrich-Lai, Y. M., Arnhold, M. M., & Engeland, W. C. (2006). Adrenal splanchnic innervation contributes to the diurnal rhythm of plasma corticosterone in rats by modulating adrenal sensitivity to ACTH. American Journal of Physiology. Regulatory, Integrative and Comparative Physiology, 290(4), R1128–35. https://doi.org/10.1152/ajpregu.00042.2003
- Wang, L., Cao, C., Wang, R., Qing, Y., Zhang, J., & Zhang, X. Y. (2013). PAC1 receptor (ADCYAP1R1) genotype is associated with PTSD's emotional numbing symptoms in Chinese earthquake survivors. Journal of Affective Disorders, 150(1), 156–159. https://doi.org/10.1016/j.jad.2013.01.010
- Yankelevitch-Yahav, R., Franko, M., Huly, A., & Doron, R. (2015). The forced swim test as a model of depressive-like behavior. Journal of Visualized Experiments : JoVE, (97)
- Zingg, B., Hintiryan, H., Gou, L., Song, M. Y., Bay, M., Bienkowski, M. S., Foster, N. N., Yamashita, S., Bowman, I., Toga, A. W., & Dong, H. W. (2014). Neural networks of the mouse neocortex. Cell, 156(5), 1096–1111. https://doi.org/10.1016/j.cell.2014.02.023
- Zusev, M., & Gozes, I. (2004). Differential regulation of activity-dependent neuroprotective protein in rat astrocytes by VIP and PACAP. Regulatory Peptides, 123(1–3), 33–41. https://doi.org/10.1016/j.regpep.2004.05.021