Abstract
Recent studies have revealed the role of L-citrulline (L-CIT) in thermoregulation, but very little is known about the mechanisms involved. In this study, nitric oxide synthase inhibition and endotoxin-induced fever were used to investigate the effects of L-CIT on body temperature and inflammatory responses. In experiment 1, NW-nitro-L-arginine methyl ester (L-NAME, 150 mg/kg BW), was i. p. injected into chicks fed with basal (CON) or L-CIT diets for 14 days. In experiment 2, Lipopolysaccharide (LPS, 2 mg/kg BW) was i. p. injected following 21d feeding with CON or L-CIT diets. In experiment 3, chickens were injected with either L-NAME, LPS, or L-NAME + LPS following 26 days feeding with CON or L-CIT diets. The rectal (RT), ear (ET), and core body temperature (CBT) of chickens were examined. Results showed that L-NAME effectively decreased the RT, ET, CBT, and plasma NO concentration. In contrast, LPS increased NO levels and initiated hyperthermia by increasing RT, ET, CBT, and PGE2 levels. L-CIT diet reduced the mean CBT in experiment 1 and diminished the NO level, PGE2 level, and mean RT in experiment 3. Co-administration of L-CIT + LPS upregulated IL-6 expression, whereas, LPS injection alone induced IL-10, IL-1β, and TLR4 gene expressions. Therefore, this study reveals that L-CIT-induced hypothermia was related to NO inhibition and a decrease in PGE2 concentration. Conversely, LPS induced hyperthermia was associated with an increase in both NO and PGE2 concentrations.
Introduction
The inflammatory response is an integral part of the immune system in living organisms (Kumar et al., Citation2011). Nitric oxide (NO) is an important factor in the initiation of inflammatory responses, as such, it can act either as an anti-inflammatory or pro-inflammatory mediator (Gamal El-Din et al., Citation2020). Lipopolysaccharide (LPS), a component of the gram-negative bacteria cell wall, is a potent immunomodulator that binds to Toll-like receptor 4 (TLR4) and activates nuclear factor kappa B (NF-kB) transcription, stimulating the downstream release of pro-inflammatory cytokines (Slawinska et al., Citation2021), free radicals, and nitrogen reactive species (Marais et al., Citation2011; Slawinska et al., Citation2016). The peripheral or central release of endogenous cytokines including interleukin-1b (IL-1β), interleukin-6 (IL-6), and tumor necrosis factor-alpha (TNF-α) stimulates LPS-induced fever (Soszynski, Citation2001). These pyrogenic cytokines are mobilized to the preoptic region of the anterior hypothalamus for the synthesis and release of prostaglandin E2 (PGE2), which is largely responsible for the upward readjustment of the thermoregulatory set point (Soszynski, Citation2001), fever induction, and the elevation of core body temperature (Dias et al., Citation2005; Gamal El-Din et al., Citation2020). As such, NO is directly involved in thermoregulation in birds and mammals (Coleone et al., Citation2009; Nakano et al., Citation2001), since it can act in the periphery and in the central nervous system to elicit thermoregulatory effects (Nakano et al., Citation2001). However, the bioavailability of NO is largely dependent on its synthesis by the different nitric oxide synthases (NOS), that is, endothelial (eNOS), neuronal (nNOS), and inducible (iNOS) isoforms (Calabro et al., Citation2018). Nω-nitro-L-Arginine methyl ester (L-NAME), is an unspecific NOS inhibitor, as such, it can competitively inhibit both constitutive and inducible NOS isoforms (Bowen et al., Citation2007). Studies in chickens have demonstrated the inhibitory role of L-NAME against NO synthesis under various conditions (Gray et al., Citation2005; Khan et al., Citation2007; Wang et al., Citation2014; Wideman et al., Citation2005).
Recently, it has been revealed that nutritional supplementation with L-citrulline (L-CIT) can lower body temperature in chickens (Chowdhury et al., Citation2015, Citation2017; Uyanga et al., Citation2021). L-CIT is a non-essential amino acid and a key metabolite of the urea cycle. In mammals and chickens, L-CIT has been ascertained to enhance L-arginine synthesis (i.e. the endogenous substrate necessary for NO production), and consequently promote peripheral NO levels (Schwedhelm et al., Citation2008; Uyanga et al., Citation2020). L-CIT is considered as a heat stress biomarker in chicks since prolonged heat stress reduces plasma L-CIT in the brain and liver embryo, while its supplementation provides thermotolerance in young chicks (Chowdhury, Citation2019; Chowdhury et al., Citation2020). Although the role of NO in thermoregulation and inflammatory response has been well established, very little is known about L-CIT, especially in avian species. Therefore, this study was designed to investigate whether L-CIT could influence the body temperature of broilers during LPS-induced fever and/or NOS inhibition. Specifically, the effects of dietary L-CIT, L-NAME, and LPS administered alone or in combination were examined on the body temperature, nitric oxide production, and cytokine expression of broilers.
Methods
Experimental animals
All experimental procedures were conducted in compliance with the “Guidelines for Experimental Animals” of the Ministry of Science and Technology (Beijing, P. R. China), and the study was approved by the Ethics Committee of Shandong Agricultural University, China.
Experimental designs
Experiment 1: Effect of dietary L-CIT on the thermal physiology of broilers during NOS inhibition
A total of 64, 817 hybrid broiler and layer chickens were used for this study. Chicks were brooded for the first 3 d at 35 °C, and the room temperature was gradually reduced to 30 °C at 7d old, where chicks were managed throughout the experiment (). Chicks were fed dietary treatments of basal diet (CON) or 1% L-Cit supplemented diet (L-CIT). L-Cit was purchased from Shandong Fosun Biotechnology Co., Ltd., China, and the dosage was adapted according to previous studies (Uyanga et al., Citation2020, Citation2021). At 10 d old, chicks were implanted with an ibutton device for core body temperature recording as previously reported (Uyanga et al., Citation2021). After 14 d feeding, chicks were grouped into a 2 × 2 factorial arrangement comprising diet (CON vs L-CIT) and injection (Saline vs L-NAME). NW-nitro-L-arginine methyl ester (L-NAME; Alladin) was dissolved in 0.9% pyrogen-free saline at a final concentration of 150 mg/kg BW and used for intraperitoneal (i.p) injection. The saline-treated group received sham injections of 0.9% saline solution proportionate to their body weight. L-NAME was freshly prepared before the injections. To avoid any effects of circadian variation, all injections were initiated between 08:30 and 09:00 h. The experiment lasted 5 h post-injection. Feed intake was measured hourly, RT was measured at 30 mins followed by hourly measurements; CBT was recorded at 30 mins intervals and the ET was taken at the 5 h timepoint.
Figure 1. Summary of study design for experiments 1 to 3.
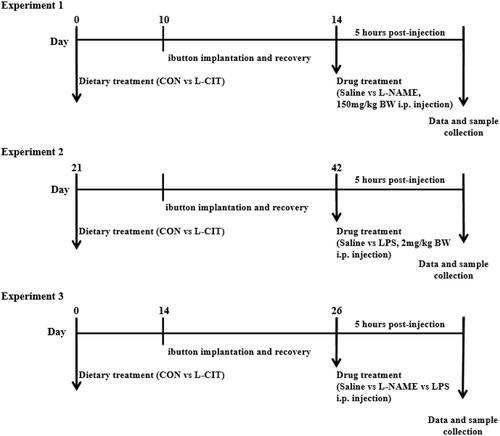
Experiment 2: Effect of dietary L-CIT on the thermal physiology of broilers during LPS induced fever
At 21 d of age, 96 chickens of the 817 hybrid broiler and layer strain with similar body weights were fed CON or L-CIT supplemented diets for 3 weeks (). The birds were implanted with ibutton thermosensors for the measurement of core body temperature and routinely managed at 24 °C. At 42 d of age, birds were grouped into a 2 × 2 factorial arrangement comprising diet (CON vs L-CIT) and injection (Saline vs LPS). Lipopolysaccharide (LPS; Sigma Aldrich) was dissolved in pyrogen-free 0.9% sodium chloride and kept on ice. Chicks were injected intraperitoneally at a dose of 2 mg/kg BW to initiate endotoxin fever. The saline-treated group received sham injections of 0.9% saline solution proportionate to their body weight, and the trial lasted for 5 h. During the LPS trial, data were recorded for RT at 30 mins intervals, and CBT at 10 mins intervals. Feed was withdrawn during the trial and all injections were initiated between 08:30 and 09:00 h to minimize the effect of circadian variation.
Experiment 3: Effect of dietary L-CIT on the thermal physiology of broilers during NOS inhibition and immune induce fever
A total of 48, day-old Arbor Acre chicks were randomized to similar body weight and fed with CON or L-CIT diets (). Chicks were implanted with an ibutton thermosensor at 14 d, and they were grouped into a 2 × 4 factorial arrangement comprising of diets (CON vs L-CIT) and injections (Saline, L-NAME, LPS, and L-NAME + LPS) at 26 d old. Injections were administered intraperitoneally (i.p) as either L-NAME (200 mg/kg BW), LPS (2 mg/kg BW), or L-NAME + LPS. To examine the effects of NOS inhibition on fever response to LPS, birds were first injected with L-NAME and then immediately injected with LPS (all injections were made separately into different sites) (Scammell et al., Citation1996; Soszynski, Citation2001). During the trial, birds were managed at 24 °C, and feed was withdrawn. The trial lasted for 5 h after injection. RT was measured within 60 min, ET was taken at end of the experiment and the CBT was recorded at 5 mins intervals. All injections were initiated between 08: 30 and 09:30 h.
Blood and tissue collection
After each experiment, blood was collected from the wing vein into heparinized tubes and centrifuged at 3000×g for 15 min at 4 °C to obtain the plasma, which was stored at −20°C. In experiment 2, the birds were exsanguinated, and the spleen was collected, frozen in liquid nitrogen, and later transferred to −80 °C for storage.
Core body temperature measurement
Core body temperature (CBT) was measured using a thermochron iButton logger (iButton, DS1922L, Maxim, CA). Data were obtained using the One wire Viewer software (Maxim Integrated Products Inc, CA).
Infrared thermography (IRT)
IRT technique was used to measure the surface (ear) temperature (ET) of broilers using an infrared thermal imager (TP 160 - Suzhou Shengguang Instrument Co. Ltd, China), which had a pixel pitch of 25 µm, a wavelength of 7.5 ∼ 14 µm, resolution of 160 × 120, and accuracy of ± 0.6 °C. Point temperature data displayed on real-time images were transferred to a computer for subsequent analysis and report generation. Data obtained from this setup has been reported as precise, reliable, and accurate (Yahav et al., Citation2005; Yahav & Giloh, Citation2012).
Rectal temperature measurement
Rectal temperature (RT) was measured using KRUUSE Vet thermometer (Cat No. 291110, China). The rectal probe was inserted at a depth of 2 − 3 cm through the cloaca into the rectum, and data was automatically generated from the device (Uyanga et al., Citation2020).
Determination of NO concentration
As previously reported, the plasma NO concentration was measured using a commercial kit (A012-1; Jiancheng Bioengineering Institute, Nanjing, Jiangsu, China) (Uyanga et al., Citation2020; Wang et al., Citation2018). Briefly, this reaction uses nitrate reductase to catalyze NAD(P)H reduction of nitrate to nitrite. The sum of nitrate and nitrite concentrations (NO2 − + NO3 −) in vivo is used to represent the total NO concentration (Bowen et al., Citation2007). The reaction absorbance was read at 550 nm using a microplate reader (Elx808, Bio-Tek, Winooski, VT).
Determination of nitric oxide synthase (NOS) activity
The total NOS (tNOS), and inducible NOS (iNOS) activities were measured according to manufacturer’s guidelines (A014-1; Jiancheng Bioengineering Institute, Nanjing, Jiangsu, China). The NOS assay is based on the enzymatic conversion of arginine and molecular oxygen to yield citrulline and NO. The optical density for tNOS and iNOS was measured with a 1 cm cuvette at 530 nm using a UV-2450 spectrophotometer (Beijing PGeneral, Beijing, China).
RNA extraction and quantitative real-time PCR analysis
The total RNA from the spleen was extracted using NcmZol reagent (NCM Biotech, China), following the manufacturer’s protocol. The nucleic acid spectrophotometer (Eppendorf, Germany) was used to determine the RNA concentration and purity at 260 nm and 280 nm (A260/280 = 1.80 − 2.00). Reverse transcription to complementary DNA (cDNA) was carried out using PrimeScriptTM reverse transcription reagent kit with gDNA eraser (Takara Bio Inc, Japan) according to manufacturer's protocol. A total of 20 µL reaction mix, constituting 2 µL of 5x diluted cDNA template, 0.8 µL of each forward and reverse primer, 10 µL of TB GreenTM Premix ExTaqTM (Takara Bio Inc, Japan), 0.4 µL of ROX reference Dye II and 6 µL DEPC water was used to perform Real-time PCR was on ABI QuantStudio 5 Real-Time PCR Instrument (Applied Biosystems, ThermoFisher Scientific, United States). Primers used for qRT-PCR were based on published target sequences (Table S1). Primers were normalized against the mRNA level of 18-S as an internal control and the CON + Saline group was used as calibrator. Thermal cycling was initiated with an initial denaturation stage of 30 s at 95 °C, followed by 40 cycles of 95 °C for 5 s and 60 °C for 30 s, and the dissociation stage. The relative mRNA expression of the target genes was analyzed using the 2−ΔΔCT method.
Statistical analysis
Data collected were analyzed using two-way ANOVA on Statistical Analysis Software (SAS version 8.1; SAS Institute Inc., Cary, NC, United States). Experiment 1 was a 2 × 2 factorial arrangement (Diet (CON vs L-CIT) and Injection (Saline vs L-NAME)). Similarly, experiment 2 was a 2 × 2 factorial arrangement (Diet (CON vs L-CIT) and Injection (Saline vs LPS)). Experiment 3 was a 2 × 4 factorial arrangement (Diet (CON vs L-CIT) and Injection (Saline, L-NAME, LPS, and L-NAME + LPS)). The RT and CBT data were analyzed using three-way repeated-measures ANOVA to evaluate the main effects of diet, injection, time, and their interactions. Differences were considered significant at p < .05. When the treatment effect(s) was significant, the means were separated using Duncan’s Multiple Range Test, and results were presented as mean ± SEM. Charts were designed using GraphPad Prism, version 8.0.2 (GraphPad Software Inc., La Jolla, CA, United States).
Results
Effects of dietary L-CIT and L-NAME on the thermal physiology of broilers
shows the IRT assessment of ET in the experimental broilers. L-NAME injection lowered the emissivity of the surface temperature than the Saline treatment as shown in the thermal images. Image quantification revealed no significant effect of diet (F[1,28] = 0.37; p = .549) or injection x diet interaction (F[1,28] = 0.12; p = .729) on the ET (). However, L-NAME lowered the ET than the Saline injection (F[1,28] = 21.22; p = <.0001). The RT was significantly influenced by injection (F[1,18] = 7.71; p = .013); time (F[6,108] = 3.57; p = .003), and time x diet interaction (F[6,108] = 2.39; p = .033). At the 60th, 120th, and 180th min, the CON + Saline group had elevated RT than other groups, but this effect was lost at the 240th and 300th min (). During the 5 h study, the administration of L-NAME significantly decreased the mean RT (F[1,28] = 13.16; p = .001) compared to the Saline injection (). The CBT was not affected by injection, and diet during the study, but it was significantly influenced by time (F[12, 72] = 6.46; p = <.0001) (). The trendline showed that CON + Saline group had higher CBT which persisted throughout the study. The mean CBT was influenced by injection x diet interaction (F[1,40] =18.47; p = .0001). The CON + Saline group had a higher mean CBT than the L-CIT + Saline and L-NAME treated groups fed either CON or L-CIT (). Also, L-NAME injection significantly lowered the mean CBT compared to Saline (F[1,40] =5.01; p = .031), while, L-CIT diet lowered the mean CBT than CON diet (F[1,40] =18.38; p = .0001). There was a significant effect of time (F[12,72] =6.46; p = <.0001) on cumulative feed intake at the 60th and 120th min post-injection ().
Figure 2. Effects of dietary L-citrulline and L-NAME on the thermal physiology of broilers (A) Infrared images of experimental animals (B) Ear temperature (C) Rectal temperature (D) Mean rectal temperature (E) Core body temperature (F) Mean core body temperature (G) Cumulative feed intake. Data are presented as mean ± SEM. *indicates significant difference at p < 0.05. a,bDifferent letters indicate significant difference at p < 0.05.
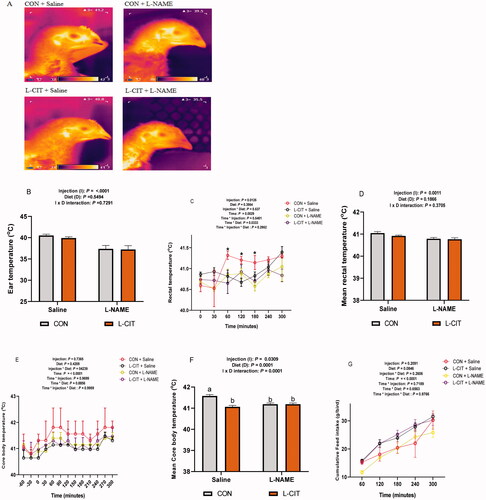
Effects of dietary L-CIT and LPS on the thermophysiology of broilers
The CBT data revealed an initial baseline of ∼41 °C before LPS administration (). There was a significant effect of injection (F[1,17] = 5.26; p = .0349); time (F[36,612] = 8.66; p = <.0001) and injection x time interaction (F[36,612] =17.25; p = <.0001) on the CBT of birds. It was observed that from 120th min, LPS injection increased the CBT, and this effect was sustained until the 300th min of the study. This corresponded with the mean CBT analysis which showed that there was no significant effect of diet (F[1,17] = 2.08; p = .168), and injection x diet interaction (F[1,17] = 0.23; p = .578) on the mean CBT. However, LPS injection increased the mean CBT higher than the Saline treatment (F[1,17] = 7.00; p = .017) ().
Figure 3. Effects of dietary L-citrulline and LPS on the thermal physiology of broilers (A) Core body temperature (B) Mean core body temperature (C) Rectal temperature (D) Mean rectal temperature (E) Infrared images of experimental animals (F) Ear temperature. Data are presented as mean ± SEM. *indicates significant difference at p < 0.05; **indicates significant difference at p < .001.
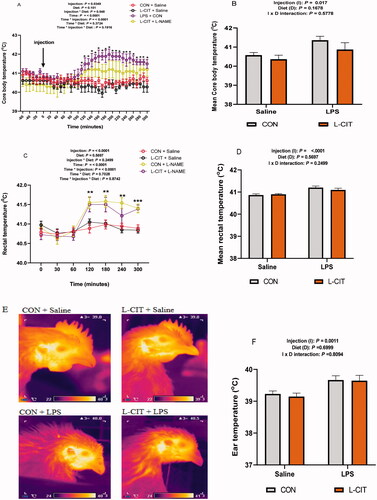
The RT was significantly influence by injection (F[1,28] = 20.74; p = <.0001); time (F[6,168] = 17.01; p = <.0001) and injection x time interaction (F[6,168] =8.04; p = <.0001). LPS significantly elevated RT from the 120th min to 300th min compared to Saline treated group (). Mean RT did not differ between CON and L-CIT diets (F[1,28] = 0.33; p = .5697), but was increased by LPS injection (F[1,28] = 20.74; p = < .0001) compared to the Saline group (). Also, the mean RT was not influenced by injection x diet interaction (F[1,28] = 1.38; p = .2499). In addition, IRT imaging revealed the ruffled feather condition in LPS treated chickens fed either CON or L-CIT diets (). Image quantification showed no differences in the ET between CON and L-CIT fed chickens (F[1,27] = 0.15; p = .6999). Also, there was no significant effect of injection x diet interaction (F[1,27] = 0.06; p = .8094). However, LPS induced higher ET (F[1,27] = 13.34; p = .001) compared to Saline injection ().
Effects of dietary L-CIT and LPS on the gene expression of inflammatory mediators in the spleen
The splenic IL-6 expression was influenced by injection x diet interaction (F[1, 26] = 7.62; p = .010). L-CIT + LPS treatment upregulated IL-6 expression compared to the L-CIT + Saline and CON + LPS (). LPS significantly upregulated IL-10 mRNA expression in the spleen (F[1, 23] = 5.29; p = .031), higher than the Saline injection (). The IL-1β expression was upregulated by LPS (F[1, 26] = 14.51; p = .0008) compared to the Saline injection (). Similarly, TLR4 expression was also induced by LPS (F[1, 20] = 5.69; p = .027) compared to Saline injection (). The splenic expression of NFkB and TNFα were not affected by injection, diet or injection x diet interaction ().
Figure 4. Transcriptional expression of inflammatory cytokines and mediators following treatment with dietary L-CIT and LPS in the spleen of broilers (A) IL-6 (B) IL-10 (C) IL-1β (D) TLR4 (E) NFκB (F) TNFα. Data are presented as mean ± SEM. a,bDifferent letters indicate significant difference at p < .05.
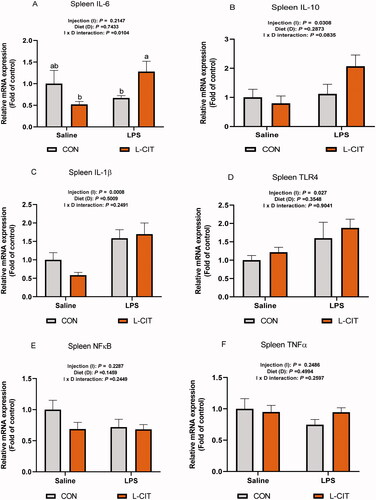
Effects of dietary L-CIT, L-NAME, and LPS treatment on the plasma NO concentration
From experiment 1, the plasma NO did not differ between the CON and L-CIT fed groups (F[1, 21] = 2.52; p = .129), and there was no significant effect of injection x diet (F[1, 21] = 0.05; p = .826). However, L-NAME significantly lowered the plasma NO concentration (F[1, 21] = 15.69; p = .0009), compared to the Saline injected group (). From experiment 2, shows that LPS injection significantly elevated the plasma NO compared to the Saline group (F[1, 28] = 53.71; p = <.0001). However, there was no significant effect of diet (F[1, 28] = 0.99; p = .328) or injection x diet interaction (F[1, 28] = 1.24; p = .275) on the plasma NO concentration.
Figure 5. Peripheral nitric oxide concentration following (A) Dietary L-citrulline and L-NAME treatment (experiment 1) (B) Dietary L-citrulline and LPS treatment (experiment 2). Data are presented as mean ± SEM.
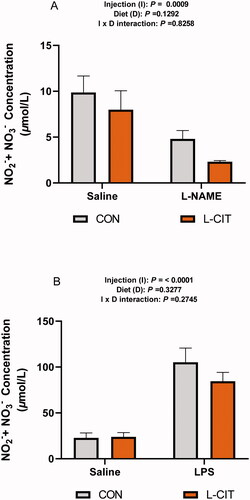
Effects of co-treatment with L-CIT, L-NAME, and LPS on the thermal physiology of broilers
There was a significant effect of injection (F[3,40] = 4.29; p = .010); diet (F[1,40] = 9.89; p = .003); time (F[5,40] = 16.31; p = <.0001) and time x diet interaction (F[5,200] = 2.43; p = .037) on the RT of chickens (). The RT was elevated by CON diet + LPS injection compared to the other treatments, and this effect was sustained from the 30th to the 300th min of study. Mean RT had a similar trend, showing that L-CIT diet significantly lowered the mean RT (F[7, 40] = 4.37; p = .001) compared to CON diet (). In addition, LPS injection increased the mean RT than L-NAME and co-treatment with L-NAME and LPS (F[7, 40] = 4.37; p = .001).
Figure 6. Effects of dietary L-citrulline, L-NAME, and LPS administration on the thermal physiology of broilers (A) Rectal temperature (B) Mean rectal temperature (C) Infrared images of experimental animals (D) Ear temperature (E) Core body temperature (F) Mean core body temperature. Data are presented as mean ± SEM. *indicates significant difference at p < .05.
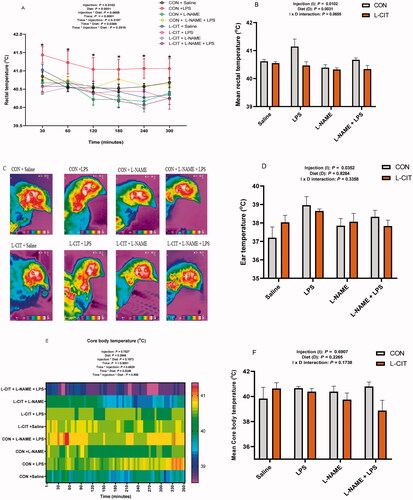
IRT imaging was captured () and the CON + LPS chickens displayed a wider heated surface area (red and yellow areas) than other groups. Image quantification revealed that LPS significantly elevated ET (F[3,38] = 3.17; p = .0352) than the Saline and L-NAME injection (). There was no significant effect of diet (F[1,38] = 0.05; p = .8284) and injection x diet interaction (F[3,38] = 1.16; p = .3358) on ET of broilers. The CBT data was influenced by time (F[72, 864] = 2.36; p = <.0001); and time x diet interaction (F[72, 864] = 1.34; p = .035). Heat mapping showed that CON + LPS, and CON + L-NAME + LPS groups tended to display higher thermal gradients, whereas, the CON + Saline, L-CIT + L-NAME, and L-CIT + LPS + L-NAME groups showed lowered thermal intensity (). Nonetheless, the mean CBT was not influenced by injection (F[3,13] = 0.50; p = .691); diet (F[1,13] = 1.61; p = .227) or injection x diet (F[3,13] = 1.94; p = .174) interaction ().
Effects of co-treatment with L-CIT, L-NAME, and LPS on NO production
Similar to the findings from , LPS administration significantly induced plasma NO concentration (F[1, 35] = 5.25; p = .041) compared to the Saline and L-NAME treated groups (). However, the plasma tNOS and iNOS activity did not differ among treatments (). Compared with the CON group, L-CIT (p < .05) decreased plasma PGE2 (F[1, 34] = 5.74, p = .022). Furthermore, LPS injection increased plasma PGE2 higher than Saline or the co-administration of L-NAME + LPS (F[3, 34] = 3.34; p = .031).
Figure 7. Effects of dietary L-citrulline, L-NAME, and LPS treatment on nitric oxide production (A) Plasma nitric oxide concentration (B) Total nitric oxide synthase (C) Inducible nitric oxide synthase (D) Prostaglandin E2 concentration.
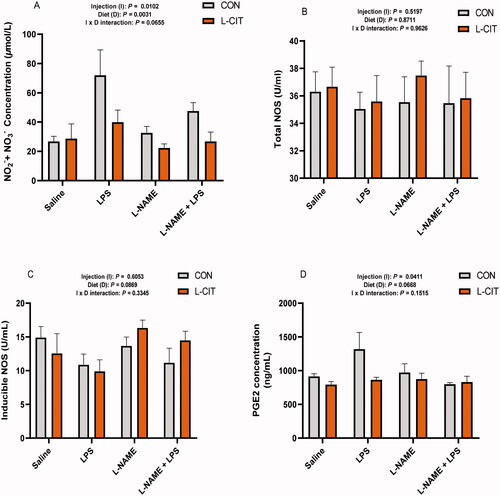
Discussion
In the present study, endotoxin-induced fever (LPS injection) and NOS inhibition (L-NAME) were applied to examine the mechanisms of L-CIT-induced hypothermia and to further elucidate the involvement of NO. The surface temperature (ear-region) was detected and thermal differences were quantified to understand the magnitude of stress-induced thermal responses among individual birds (Tabh et al., Citation2021). The results revealed significant variabilities in the ear, rectal, and core body temperatures attributed to the dietary treatments and/or injections administered. Few studies had reported that there was no significant influence of L-CIT on RT in KUB chicks, laying hens, and pigs (Erwan et al., Citation2020; Kvidera et al., Citation2016; Liu et al., Citation2019; Uyanga et al., Citation2020), which corresponded to our findings from experiment 2. Contrarily, it was also observed that L-CIT could lower the mean CBT (experiment 1), as well as the RT and mean RT (experiment 3) of broilers. These findings corroborate with the reports on L-CIT-induced hypothermia (Chowdhury et al., Citation2015, Citation2017; Uyanga et al., Citation2021), however, the disparity in findings may be attributed to differences in species, age of birds, ambient temperature, as well as the route of L-CIT administration.
L-NAME treatment decreases plasma NO due to the inhibition of endogenous NO production and NOS synthesis (Peeri et al., Citation2013; Sonoda et al., Citation2017). A previous report by Chowdhury et al. (Citation2017) had shown that oral L-CIT (15 mmol/10 ml/kg BW) lowered RT of birds, but L-NAME caused greater suppression of RT and reduced the plasma NO concentration. Our findings corroborate with this report regarding L-NAME suppression of body temperature (RT, ET, and CBT), as well as plasma NO. In addition, L-NAME interacted with L-CIT to lower the mean CBT as seen in experiment 1. This suggests that the L-CIT and L-NAME could synergize to suppress body temperature in birds.
In contrast, LPS is an exogenous pyrogen that induces febrile responses in mammals and birds. However, the patterns of CBT changes in response to LPS are variable due to differences in injection routes, dosage, and ambient temperature (Dias et al., Citation2005; Grabbe et al., Citation2020). In experiment 2, LPS administration elevated CBT at 2 h post-injection and this effect lasted throughout the study. A similar increment was observed in the mean CBT, RT, mean RT, and ET of broilers. This rise in body temperature can be attributed to immune-induced fever since LPS can stimulate prostaglandin E2 release, which allows for the upregulation and adjustment of the central set-point of body temperature in the hypothalamus (Nakamura et al., Citation2018). Interestingly, this febrile response was not attenuated by dietary L-CIT. Additionally, the ruffled feather condition was observed in LPS treated birds, which corroborates with earlier reports that LPS infected chickens exhibit increased cloacal temperature and physical symptoms such as drowsiness, depression, ruffled feathers, anorexia, lethargy, and slight diarrhea (Cheng et al., Citation2017; Tachibana et al., Citation2017). In chickens, LPS stimulates iNOS activity and expression, leading to an increase in NO production in avian macrophages (Chapman et al., Citation2005; Nikami et al., Citation2008). Broilers injected with LPS had increased iNOS activation in circulating monocytes and macrophages, resulting in a significant increment of total plasma NO that peaked at 5 h post-injection (Bowen et al., Citation2007). These reports corroborate our findings that LPS administration could effectively increase NO levels whether in the presence or absence of L-CIT.
Investigation into the influence of L-CIT and LPS on the gene expression of inflammatory cytokines in the spleen revealed that co-administration of L-CIT and LPS invoked a pro-inflammatory response by upregulating IL-6 expression. LPS administration further increased splenic IL-10, IL-1β, and TLR4 mRNA expression. Pro-inflammatory cytokines, particularly IL-1β and IL-6 are well known to mediate immune functions in the periphery and also function in fever initiation (Marais et al., Citation2011; Nakamura et al., Citation2018). Hence, the increased expression of these cytokines could be related to LPS induced febrile response. Alongside this, TLR4 acts as a signaling receptor for LPS, and once activated, it stimulates the synthesis and release of critical pro-inflammatory cytokines (Chen et al., Citation2018; Llewellyn & Foey, Citation2017). Previous reports have shown that L-CIT can establish an anti-inflammatory profile by increasing IL-10 but it lowered IL-1β and IL-12 production (Romero et al., Citation2013). This contradicts our findings since dietary L-CIT could not counteract LPS induced inflammation.
To further understand the mechanism of L-CIT actions, L-NAME and LPS were co-administered to broilers fed with CON or L-CIT diets in experiment 3. Similar to our findings from experiment 2, LPS elevated the RT of broilers but the administration of L-NAME or L-NAME + LPS decreased the RT. The hypothermic effect of the L-CIT diet was evident on the RT and mean RT in experiment 3, in contrast, CON + LPS interacted to elevate the RT of broilers. These findings corroborate previous studies that examined the co-administration of L-NAME and LPS on body temperature. In 5d old chicks, LPS (2 µg/kg i.m) induced fever at 3 to 5 h post-injection, whereas, L-NAME (50 mg/kg i.m) attenuated LPS-fever and facilitated CBT reduction (Dantonio et al., Citation2016). The L-NAME attenuation of LPS fever was attributed to thermoeffector activity, rather than alterations in the immune-to-brain signaling (Dantonio et al., Citation2016). In mammalian studies, i.p. L-NAME (50 mg/kg) attenuated LPS-induced fever in rats due to minimal blood outflow from the sympathetic nervous system to the brown adipose tissue, consequently inhibiting brown fat thermogenesis (Soszynski, Citation2001). Also, L-NAME injection to conscious, unrestrained rats, induced dose-dependent hypothermia while LPS produced a dose-dependent febrile response, but L-NAME along with LPS resulted in the attenuation of LPS induced fever, causing marked hypothermia (Scammell et al., Citation1996). Therefore, the present study successfully demonstrated that L-NAME induced hypothermia; LPS induced febrile response, and validated the efficacy of L-NAME to attenuate LPS febrile response when co-administered in broilers. In line with these findings, there was a possible association with NO production. Notably, LPS increment in body temperature was related to its induction of NO production, whereas, L-NAME induced hypothermia was linked with its reduction of NO production. Interestingly, experiment 3 also revealed the possible association between L-CIT’s reduction of RT and its attenuation of NO and PGE2 production. Typically, LPS acts to increase the plasma and serum PGE2 concentration in chickens (Fraifeld et al., Citation1995; Zhang et al., Citation2011). High dose LPS administration (100 µg/kg im) increased brain PGE2 concentration, while L-NAME pretreatment did not influence LPS induced PGE2 levels in both the plasma and brain of chicks (Dantonio et al., Citation2016). From experiment 3, LPS elevated plasma PGE2, whereas, L-CIT acted to diminish PGE2 concentration. Therefore, it is probable that the reduction in NO and PGE2 by L-CIT would likely function to reduce body temperature, thereby facilitating hypothermia induction (Tan & Knight, Citation2018).
Taken together, these findings demonstrate an association between NO generation and body temperature changes as influenced by diet. The finding that L-CIT could elicit pronounced effects on NO and body temperature as seen in experiment 3, as opposed to the lack of pronounced effects in experiments 1 and 2 suggests differential responses that may be attributed to strain effect. It is noteworthy that the huge genetic diversity in modern-day broiler strains allows most commercial broilers to be highly susceptible to environmental stressors and physiological alterations (He et al., Citation2018; Nawaz et al., Citation2021). Thus, we speculate that the 817 hybrid strains used in experiments 1 and 2 may be hardier than the conventional broiler strain (Arbor acre) which was used in experiment 3, leading to inconsistency in body temperature changes.
Conclusion
This study demonstrated that dietary L-CIT supplementation influenced broilers’ nitric oxide production and body temperature. LPS administration induced hyperthermia, inflammation and increased the peripheral NO and PGE2 production. Contrarily, L-NAME initiated hypothermia, inhibited NO production, and attenuated PGE2 synthesis. Feeding commercial broiler strain with L-CIT supplemented diet reduced the rectal temperature, and diminished the NO and PGE2 concentration similarly to L-NAME actions. Summarily, the effects of L-CIT on thermoregulation and NO regeneration in poultry still require further exploration using different routes of administration, doses, and examinations under acute and chronic stress conditions.
Author contributions
The study was designed by VAU and HL. VAU analyzed, interpreted, and presented the data. JZ, XW, HJ, OMO and HL contributed to the research design and result interpretation. All authors read, reviewed, and approved the final manuscript.
Ethics approval
The study was reviewed and approved by the Institutional Animal Care and Use Committee at the College of Animal Science of Shandong Agriculture University, and carried out following the “Guidelines for Experimental Animal” of Ministry of Science and Technology (Beijing, P. R. China).
Disclosure statement
No potential conflict of interest was reported by the authors.
Data availability statement
All datasets obtained and analyzed in this research are included in this article.
Additional information
Funding
Notes on contributors
Victoria Anthony Uyanga
Victoria Anthony Uyanga is a doctoral researcher in the field of Animal Nutrition and Physiology. Her research interest includes Stress physiology, amino acid metabolism, and immunomodulation.
Jingpeng Zhao
Jingpeng Zhao is a professor of Animal Science at Shandong Agricultural University.
Xiaojuan Wang
Xiaojuan Wang is an Assistant professor of Animal Science at Shandong Agricultural University.
Hongchao Jiao
Hongchao Jiao is a professor of Animal Science at Shandong Agricultural University.
Okanlawon M. Onagbesan
Okanlawon M. Onagbesan is a professor in the field of Animal Physiology. He specializes in endocrinology, reproductive physiology and environmental physiology.
Hai Lin
Hai Linis a professor Animal Nutrition and Feed Sciences at Shandong Agricultural University. He specializes in Stress physiology, Poultry nutrition and Nutritional biochemistry.
References
- Bowen, O. T., Erf, G. F., Chapman, M. E., & Wideman, R. F. Jr.(2007). Plasma nitric oxide concentrations in broilers after intravenous injections of lipopolysaccharide or microparticles. Poultry Science, 86(12), 2550–2554. https://doi.org/10.3382/ps.2007-00288
- Calabro, V., Litterio, M. C., Fraga, C. G., Galleano, M., & Piotrkowski, B. (2018). Effects of quercetin on heart nitric oxide metabolism in l-name treated rats. Archives of Biochemistry and Biophysics, 647, 47–53. https://doi.org/10.1016/j.abb.2018.03.041
- Chapman, M. E., Wang, W., Erf, G. F., & Wideman, R. F. Jr.(2005). Pulmonary hypertensive responses of broilers to bacterial lipopolysaccharide (LPS): Evaluation of LPS source and dose, and impact of pre-existing pulmonary hypertension and cellulose micro-particle selection. Poultry Science, 84(3), 432–441. https://doi.org/10.1093/ps/84.3.432
- Chen, Y., Zhang, H., Cheng, Y., Li, Y., Wen, C., & Zhou, Y. (2018). Dietary L-threonine supplementation attenuates lipopolysaccharide-induced inflammatory responses and intestinal barrier damage of broiler chickens at an early age. The British Journal of Nutrition, 119(11), 1254–1262. https://doi.org/10.1017/S0007114518000740
- Cheng, P., Wang, T., Li, W., Muhammad, I., Wang, H., Sun, X., Yang, Y., Li, J., Xiao, T., & Zhang, X. (2017). Baicalin alleviates lipopolysaccharide-induced liver inflammation in chicken by suppressing TLR4-mediated nf-κb pathway. Frontiers in Pharmacology, 8, 547. https://doi.org/10.3389/fphar.2017.00547
- Chowdhury, V. S. (2019). Heat stress biomarker amino acids and neuropeptide afford thermotolerance in chicks. The Journal of Poultry Science, 56(1), 1–11. https://doi.org/10.2141/jpsa.0180024
- Chowdhury, V. S., Han, G., Bahry, M. A., Tran, P. V., Do, P. H., Yang, H., & Furuse, M. (2017). L-citrulline acts as potential hypothermic agent to afford thermotolerance in chicks. Journal of Thermal Biology, 69, 163–170. https://doi.org/10.1016/j.jtherbio.2017.07.007
- Chowdhury, V. S., Han, G., Eltahan, H. M., Haraguchi, S., Gilbert, E. R., Cline, M. A., Cockrem, J. F., Bungo, T., & Furuse, M. (2020). Potential role of amino acids in the adaptation of chicks and market-age broilers to heat stress. Frontiers in Veterinary Science, 7, 610541. https://doi.org/10.3389/fvets.2020.610541
- Chowdhury, V. S., Shigemura, A., Erwan, E., Ito, K., Bahry, M. A., Tran, P. V., & Furuse, M. (2015). Oral administration of l-citrulline, but not l-arginine or l-ornithine, acts as a hypothermic agent in chicks. The Journal of Poultry Science, 52(4), 331–335. https://doi.org/10.2141/jpsa.0150014
- Coleone, A. C., Torres, K. A., Carnio, E. C., Gargaglioni, L. H., Macari, M., Furlan, R. L., & Bicego, K. C. (2009). Role of brain nitric oxide in the thermoregulation of broiler chicks. Comparative Biochemistry and Physiology. Part A, Molecular & Integrative Physiology, 154(2), 204–210. https://doi.org/10.1016/j.cbpa.2009.06.005
- Dantonio, V., Batalhao, M. E., Fernandes, M. H., Komegae, E. N., Buqui, G. A., Lopes, N. P., Gargaglioni, L. H., Carnio, E. C., Steiner, A. A., & Bicego, K. C. (2016). Nitric oxide and fever: Immune-to-brain signaling vs. Thermogenesis in chicks. American Journal of Physiology. Regulatory, Integrative and Comparative Physiology, 310(10), R896–905. https://doi.org/10.1152/ajpregu.00453.2015
- Dias, M. B., Almeida, M. C., Carnio, E. C., & Branco, L. G. (2005). Role of nitric oxide in tolerance to lipopolysaccharide in mice. Journal of Applied Physiology, 98(4), 1322–1327. https://doi.org/10.1152/japplphysiol.01243.2004
- Erwan, E., Adelina, T., Koto, A., & Maslami, V. (2020). The potency of oral administration of l-citrulline as anti heat stress agent in KUB chickens. Journal of World’s Poultry Research, 10(1), 36–40. https://doi.org/10.36380/jwpr.2020.5
- Fraifeld, V., Blaicher-Kulick, R., Degen, A. A., & Kaplanski, J. (1995). Is hypothalamic prostaglandin e2 involved in avian fever? Life Sciences, 56(16), 1343–1346. https://doi.org/10.1016/0024-3205(95)00086-0
- Gamal El-Din, M. M., El-Gamal, M. I., Abdel-Maksoud, M. S., Lee, H., Choi, J., Kim, T. W., Shin, J. S., Lee, H. H., Kim, H. K., Lee, K. T., & Baek, D. (2020). Inhibitory effects of triarylpyrazole derivatives on LPS-induced nitric oxide and pge2 productions in murine raw 264.7 macrophages. Bioorganic & Medicinal Chemistry Letters, 30(4), 126884. https://doi.org/10.1016/j.bmcl.2019.126884
- Grabbe, N., Kaspers, B., Ott, D., Murgott, J., Gerstberger, R., & Roth, J. (2020). Neurons and astrocytes of the chicken hypothalamus directly respond to lipopolysaccharide and chicken interleukin-6. J Comp Physiol B, 190(1), 75–85. https://doi.org/10.1007/s00360-019-01249-1
- Gray, D. A., Maloney, S. K., & Kamerman, P. R. (2005). Lipopolysaccharide-induced fever in pekin ducks is mediated by prostaglandins and nitric oxide and modulated by adrenocortical hormones. American Journal of Physiology. Regulatory, Integrative and Comparative Physiology, 289(5), R1258–1264. https://doi.org/10.1152/ajpregu.00377.2005
- He, X. F., Lu, Z., Ma, B., Zhang, L., Li, J. L., Jiang, Y., Zhou, G. H., & Gao, F. (2018). Effects of chronic heat exposure on growth performance, intestinal epithelial histology, appetite-related hormones and genes expression in broilers. Journal of the Science of Food and Agriculture, 98(12), 4471–4478. https://doi.org/10.1002/jsfa.8971
- Khan, M. S., Tachibana, T., Hasebe, Y., Masuda, N., & Ueda, H. (2007). Peripheral or central administration of nitric oxide synthase inhibitor affects feeding behavior in chicks. Comparative Biochemistry and Physiology. Part A, Molecular & Integrative Physiology, 148(2), 458–462. https://doi.org/10.1016/j.cbpa.2007.06.006
- Kumar, S., Ciraci, C., Redmond, S. B., Chuammitri, P., Andreasen, C. B., Palić, D., & Lamont, S. J. (2011). Immune response gene expression in spleens of diverse chicken lines fed dietary immunomodulators. Poultry Science, 90(5), 1009–1013. https://doi.org/10.3382/ps.2010-01235
- Kvidera, S. K., Mayorga, E. J., Seibert, J. T., Ross, J. W., Rhoads, R. P., Horst, E. A., Al-Qaisi, M. A., & Baumgard, L. H. (2016). Effect of supplemental citrulline on thermal and production parameters during heat stress in growing pigs. Journal of Animal Science, 94(suppl_5), 477–477. https://doi.org/10.2527/jam2016-0995
- Liu, F., de Ruyter, E. M., Athorn, R. Z., Brewster, C. J., Henman, D. J., Morrison, R. S., Smits, R. J., Cottrell, J. J., & Dunshea, F. R. (2019). Effects of l-citrulline supplementation on heat stress physiology, lactation performance and subsequent reproductive performance of sows in summer. Journal of Animal Physiology and Animal Nutrition, 103(1), 251–257. https://doi.org/10.1111/jpn.13028
- Llewellyn, A., & Foey, A. (2017). Probiotic modulation of innate cell pathogen sensing and signaling events. Nutrients, 9(10), 1156. https://doi.org/10.3390/nu9101156
- Marais, M., Maloney, S. K., & Gray, D. A. (2011). Brain il-6- and pg-dependent actions of il-1β and lipopolysaccharide in avian fever. American Journal of Physiology. Regulatory, Integrative and Comparative Physiology, 301(3), R791–R800. https://doi.org/10.1152/ajpregu.00136.2011
- Nakamura, Y., Nakanishi, T., Shimada, H., Shimizu, J., Aotani, R., Maruyama, S., Higuchi, K., Okura, T., Deguchi, Y., & Tamai, I. (2018). Prostaglandin transporter oatp2a1/slco2a1 is essential for body temperature regulation during fever. The Journal of Neuroscience, 38(24), 5584–5595. https://doi.org/10.1523/JNEUROSCI.3276-17.2018
- Nakano, H., Lee, S.-D., Ray, A. D., Krasney, J. A., & Farkas, G. A. (2001). Role of nitric oxide in thermoregulation and hypoxic ventilatory response in obese zucker rats. American Journal of Respiratory and Critical Care Medicine, 164(3), 437–442. https://doi.org/10.1164/ajrccm.164.3.2010142
- Nawaz, A. H., Amoah, K., Leng, Q. Y., Zheng, J. H., Zhang, W. L., & Zhang, L. (2021). Poultry response to heat stress: Its physiological, metabolic, and genetic implications on meat production and quality including strategies to improve broiler production in a warming world. Frontiers in Veterinary Science, 8, 699081. https://doi.org/10.3389/fvets.2021.699081
- Nikami, H., Mahmoud, M. E., Shimizu, Y., Shiina, T., Hirayama, H., Iwami, M., Dosoky, R. M., Ahmed, M. M., & Takewaki, T. (2008). Capsaicin pretreatment attenuates lps-induced hypothermia through trpv1-independent mechanisms in chicken. Life Sciences, 82(23–24), 1191–1195. https://doi.org/10.1016/j.lfs.2008.04.003
- Peeri, M., Habibian, M., Azarbayjani, M. A., & Hedayati, M. (2013). Protective effect of aerobic exercise against l-name-induced kidney damage in rats. Archives of Industrial Hygiene and Toxicology, 64(2), 229–235. https://doi.org/10.2478/10004-1254-64-2013-2260
- Romero, M. J., Yao, L., Sridhar, S., Bhatta, A., Dou, H., Ramesh, G., Brands, M. W., Pollock, D. M., Caldwell, R. B., Cederbaum, S. D., Head, C. A., Bagi, Z., Lucas, R., & Caldwell, R. W. (2013). L-citrulline protects from kidney damage in type 1 diabetic mice. Frontiers in Immunology, 4, 480. https://doi.org/10.3389/fimmu.2013.00480
- Scammell, T. E., Elmquist, J. K., & Saper, C. B. (1996). Inhibition of nitric oxide synthase produces hypothermia and depresses lipopolysaccharide fever. Am J Physiol, 271(2 Pt 2), R333–R338. https://doi.org/10.1152/ajpregu.1996.271.2.R333
- Schwedhelm, E., Maas, R., Freese, R., Jung, D., Lukacs, Z., Jambrecina, A., Spickler, W., Schulze, F., & Boger, R. H. (2008). Pharmacokinetic and pharmacodynamic properties of oral l-citrulline and l-arginine: Impact on nitric oxide metabolism. British Journal of Clinical Pharmacology, 65(1), 51–59. https://doi.org/10.1111/j.1365-2125.2007.02990.x
- Slawinska, A., Dunislawska, A., Plowiec, A., Gonçalves, J., & Siwek, M. (2021). Tlr-mediated cytokine gene expression in chicken peripheral blood mononuclear cells as a measure to characterize immunobiotics. Genes, 12(2), 195. https://doi.org/10.3390/genes12020195
- Slawinska, A., Hsieh, J. C., Schmidt, C. J., & Lamont, S. J. (2016). Heat stress and lipopolysaccharide stimulation of chicken macrophage-like cell line activates expression of distinct sets of genes. PLoS One, 11(10), e0164575. https://doi.org/10.1371/journal.pone.0164575
- Sonoda, K., Ohtake, K., Uchida, H., Ito, J., Uchida, M., Natsume, H., Tamada, H., & Kobayashi, J. (2017). Dietary nitrite supplementation attenuates cardiac remodeling in l-name-induced hypertensive rats. Nitric Oxide, 67, 1–9. https://doi.org/10.1016/j.niox.2017.04.009
- Soszynski, D. (2001). The inhibition of nitric oxide synthase suppresses LPS- and psychological stress-induced fever in rats. Physiology & Behavior, 72(1–2), 65–72. https://doi.org/10.1016/S0031-9384(00)00375-9
- Tabh, J. K. R., Burness, G., Wearing, O. H., Tattersall, G. J., & Mastromonaco, G. F. (2021). Infrared thermography as a technique to measure physiological stress in birds: Body region and image angle matter. Physiological Reports, 9(11), e14865. https://doi.org/10.14814/phy2.14865
- Tachibana, T., Nakai, Y., Makino, R., Khan, M. S. I., & Cline, M. A. (2017). Effect of central and peripheral injection of prostaglandin e2 and F2α on feeding and the crop-emptying rate in chicks. Prostaglandins Other Lipid Mediat, 130, 30–37. https://doi.org/10.1016/j.prostaglandins.2017.03.005
- Tan, C. L., & Knight, Z. A. (2018). Regulation of body temperature by the nervous system. Neuron, 98(1), 31–48. https://doi.org/10.1016/j.neuron.2018.02.022
- Uyanga, V. A., Jiao, H., Zhao, J., Wang, X., & Lin, H. (2020). Dietary l-citrulline supplementation modulates nitric oxide synthesis and anti-oxidant status of laying hens during summer season. Journal of Animal Science and Biotechnology, 11, 103. https://doi.org/10.1186/s40104-020-00507-5
- Uyanga, V. A., Wang, M., Tong, T., Zhao, J., Wang, X., Jiao, H., Onagbesan, O. M., & Lin, H. (2021). L-citrulline influences the body temperature, heat shock response and nitric oxide regeneration of broilers under thermoneutral and heat stress condition. Frontiers in Physiology, 12, 671691. https://doi.org/10.3389/fphys.2021.671691
- Wang, C., Hou, S. S., Huang, W., Xu, T. S., Rong, G. H., & Xie, M. (2014). Arginine affects appetite via nitric oxide in ducks. Poultry Science, 93(8), 2048–2053. https://doi.org/10.3382/ps.2013-03812
- Wang, R., Jiao, H., Zhao, J., Wang, X., & Lin, H. (2018). L-arginine enhances protein synthesis by phosphorylating mTOR (thr 2446) in a nitric oxide-dependent manner in c2c12 cells. Oxidative Medicine and Cellular Longevity, 2018, 7569127. https://doi.org/10.1155/2018/7569127
- Wideman, R. F., Erf, G. F., & Chapman, M. E. (2005). Nomega-nitro-l-arginine methyl ester (L-NAME) amplifies the pulmonary hypertensive response to microparticle injections in broilers. Poultry Science, 84(7), 1077–1091. https://doi.org/10.1093/ps/84.7.1077
- Yahav, S., & Giloh, M. (2012). Infrared thermography – Applications in poultry biological research. In Prakash, R. V. (Ed.). Infrared thermography InTech.
- Yahav, S., Shinder, D., Tanny, J., & Cohen, S. (2005). Sensible heat loss: The broiler’s paradox. World’s Poultry Science Journal, 61(3), 419–434. https://doi.org/10.1079/WPS200453
- Zhang, W. H., Jiang, Y., Zhu, Q. F., Gao, F., Dai, S. F., Chen, J., & Zhou, G. H. (2011). Sodium butyrate maintains growth performance by regulating the immune response in broiler chickens. British Poultry Science, 52(3), 292–301. https://doi.org/10.1080/00071668.2011.578121