Abstract
The importance of social interactions has been reported in a variety of animal species. In human and rodent models, social isolation is known to alter social behaviors and change anxiety or depression levels. During the coronavirus pandemic, although people could communicate with each other through other sensory cues, social touch was mostly prohibited under different levels of physical distancing policies. These social restrictions inspired us to explore the necessity of physical contact, which has rarely been investigated in previous studies on mouse social interactions. We first conducted a long-term observation to show that pair-housed mice in a standard laboratory cage spent nearly half the day in direct physical contact with each other. Furthermore, we designed a split-housing condition to demonstrate that even with free access to visual, auditory, and olfactory social signals, the lack of social touch significantly increased anxiety-like behaviors and changed social behaviors. There were correspondingly higher levels of the pro-inflammatory cytokine interleukin-6 in the hippocampus in mice with no access to physical contact. Our study demonstrated the necessity of social touch for the maintenance of mental health in mice and could have important implications for human social interactions.
Introduction
Social animals, including humans, require constant social interactions to maintain physical and mental health. During the COVID-19 pandemic, most countries have, by necessity, issued orders involving physical distancing, social quarantines, or stay-at-home rules to prevent the spread of the coronavirus. These restrictions not only affected daily life but also caused potential health concerns. Research has suggested the influence of social isolation on mental illness, including anxiety and depression, along with an increase in suicide risk (Cacioppo et al., Citation2015; Robb et al., Citation2020; Trout, Citation1980). Numerous studies have also indicated that social disengagement is closely linked to physiological problems such as cognitive decline, disability and mortality (White et al., Citation2015). After the emergence of the pandemic, these issues imposed further complexity and burdens on the health system.
The impact of social isolation has also been investigated in rodent models, although the results from different studies were not always consistent. Regarding anxiety and depression, to correspond with human findings, reports have usually focused on the deleterious impacts of social isolation, i.e. increased anxiety-like and depression-like behaviors (Mumtaz et al., Citation2018). However, although less emphasized, no effect of social isolation has also been found in several studies (Bibancos et al., Citation2007; Hohlbaum et al., Citation2020; Pietropaolo et al., Citation2008; Rodgers & Cole, Citation1993). In fact, some studies have reported that social isolation results in an anti-anxiety phenotype, compared with social stress associated with group housing conditions (Lopez & Laber, Citation2015; Silva et al., Citation2011; Singewald et al., Citation2009). The impacts of social isolation on the stress hormone corticosterone have also been variable in rodent research (Ieraci et al., Citation2016; Kamal et al., Citation2014; Mumtaz et al., Citation2018). These inconsistent results indicated that the effects of social isolation in mice are very complicated, and the causes underlying these inconsistencies remain to be studied.
Multiple social signals are exchanged during social interactions. Although some social modalities, such as olfactory and auditory cues, have been extensively studied and suggested to be important in alleviating isolation syndrome (Pais et al., Citation2019; Pietropaolo et al., Citation2008), the specific influences of social touch on mouse health and physiology have rarely been addressed. In fact, during the COVID-19 pandemic, people could still exchange visual or auditory signals through virtual meetings. However, physical contact, such as hugging or handshaking, was strictly prohibited. These social restrictions inspired us to ask whether social touch may play a crucial role in maintaining mental health.
To address this issue, in this study, we evaluated the importance of physical touch by establishing a split-housing environment in which mice could freely exchange visual, olfactory, and auditory cues but could not physically contact each other. Male mice placed in split-housing conditions showed increased anxiety-like behaviors and altered social behavior compared with mice in standard pair- or single-housing. Concurrently, we observed elevated interleukin-6 (IL-6) in mice from the split-housing conditions. Our study demonstrated a critical role of physical contact in maintaining normal mouse behavioral performance. This information highlights the importance of social touch for health and may have potential implications for human interactions.
Results
Male mice spent half the day in social touch
To examine the proportion of social touch time in the daily interactions of mice, C57BL/6J males were housed in pairs, and all interactions were video recorded for 24 h. The videos were analyzed based on two parameters, the activity of each mouse (moving or no moving) and physical contact between two mice (contact or no contact) (). As expected, mice engaged in different activities and behavioral patterns between the light and dark periods. In the dark, mice mostly displayed exploratory behaviors without direct contact (both moving–no contact) (H(5) = 13.3, p = 0.0207; ). In the light period, the activity levels of the mice decreased, and physical contact increased (no movement–contact) (H(5) = 15.94, p = 0.007; ). Importantly, in both the light and dark periods, there was a very minimal amount of time during which both mice were inactive and were not in physical contact (no movement–no contact), suggesting that mice mostly huddled together during their resting time. In summary, our 24-h observation showed that mice spent half the day in physical contact with each other (), implying a strong motivation for social touch in their daily interactions.
Figure 1. Mice spent half a day physically contacting each other. (A) A raster plot showing the physical interactions of pair-housed mice over 24 h. (B) The total time for specific interactions between two mice in the dark phase (Kruskal–Wallis followed by Dunn’s test, p values were determined by comparison to both moving-no contact). (C) The total time for specific interactions between two mice in the light phase (Kruskal–Wallis followed by Dunn’s test, p values were determined by comparison to no moving-contact). (D) The percentage of time for specific interactions between two mice in 24 hours. *p < 0.05, **p < 0.01, ***p < 0.001; n = 3 pairs; mean ± S.E.M.

Deprivation of social touch increased anxiety-like behaviors
Next, to investigate the necessity of social touch for mouse mental health, we housed two C57BL/6J male mice in a divided cage. Mice in this cage were separated by a transparent plate with holes, which allowed olfactory, visual, and auditory communications but minimized direct physical contact () (Video S1). After one month of this housing arrangement, we examined these split-housed mice for behaviors related to psychological symptoms and compared them with mice living in single-housed and standard pair-housed conditions ().
Figure 2. Three different housing conditions and the experimental schedule. (A) Male mice were housed in three different conditions: pair-, split-, and single-housing conditions. (B) Schematic diagram of the experimental schedule.
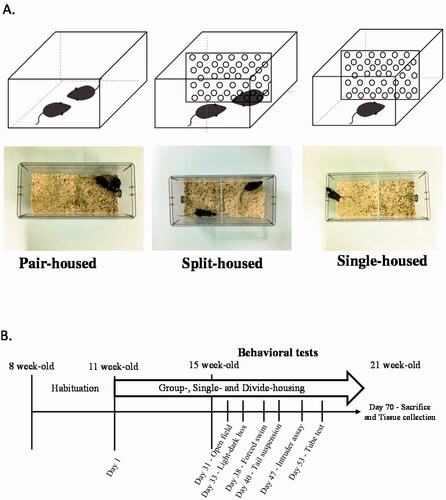
The levels of anxiety-like behaviors were evaluated by the open field test and the light-dark box test (Seibenhener & Wooten, Citation2015; Takao & Miyakawa, Citation2006) (). First, the open field test showed that there was a significant decrease in activity in single-housed mice but no difference between pair-housed and split-housed animals (W(2,23.2) = 7.028, p = 0.0041; ). Surprisingly, although there was no difference between pair- and single-housed animals, split-housed mice spent significantly less time in the center of the arena than pair-housed or single-housed mice (W(2,22.9) = 6.078, p = 0.0076; ). In the light-dark box test, split-housed mice also spent significantly less time in the light chamber than pair-housed or single-housed animals (W(2,20.36) = 18.79, p < 0.0001; ). The number of transitions between the two chambers was lower in the split-housed group than in the pair-housed group (W(2,24.28) = 14.03, p < 0.0001; ). Together, the two experiments indicated increased anxiety-like behaviors in split-housed mice.
Figure 3. Mice housed in divided cages showed increased anxiety-like behavior. (A) Representative traces of mice in the open field. (B) Travel distances in the open-field test. (C) Time spent in the inner zone in the open-field test. (D) Time spent in the light zone during the light-dark box test. (E) The number of transitions between the light and dark zones. Welch's ANOVA followed by Dunn’s test; *p < 0.05, **p < 0.01, ***p < 0.001; n = 12–14 per group; mean ± S.E.M.
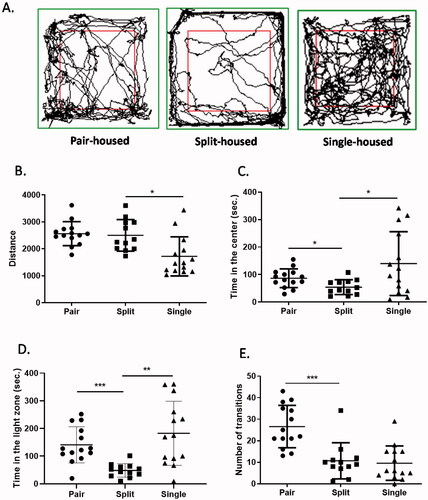
We also conducted the forced swim and tail suspension tests, both of which are antidepressant screening tests for measuring motor reactivity to stress (Can et al., Citation2012; Steru et al., Citation1985). In terms of latency to the first bout of immobility and total immobility time, we did not detect any difference among the three groups in either assay (forced swimming latency: W(2,20.43) = 1.506, p = 0.2454; forced swimming immobility: W(2,21.5) = 2.12, p = 0.1445; tail suspension latency: W(2,17.72) = 3.306, p = 0.0602; tail suspension immobility: W(2,19.69) = 1.15, p = 0.3369) (), suggesting that lack of physical touch raises the levels of anxiety-like behaviors but may not have an influence on anti-depression activity in mice.
Figure 4. Depression-like behavior was similar among mice housed in different conditions. (A) Latency to the first bout of immobility in the forced swim test. (B) The total immobility time in the forced swim test. (C) Latency to the first bout of immobility in the tail suspension test. (D) The total immobility time in the tail suspension test. Welch's ANOVA followed by Dunn’s test; n = 12–14 per group; mean ± S.E.M.
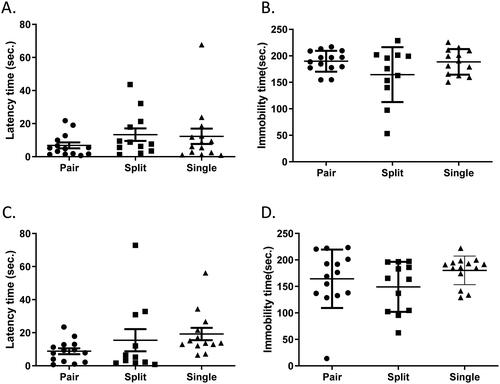
Physical isolation modulated social behaviors
A previous study using the tube test and the open-field test showed higher levels of anxiety-like behavior in dominant mice than in subordinate mice (Larrieu et al., Citation2017). We therefore applied the tube test to compare social dominance through pairwise interactions. Consistent with the previous finding, we found a higher win rate in split-housed mice than in single-housed mice (Split vs. Single: p = 0.0281) (). The difference between split- and pair-housed mice was also close to significance (Pair vs. Split: p = 0.0547), suggesting higher dominant social rank in split-housed mice. There was no difference between mice housed in paired- and single-housing conditions (Pair vs. Single: p = 0.2344).
Figure 5. Mice housed in divided cages showed changes in social behaviors. (A) Social dominance evaluated by pairwise comparisons in the tube test (Wilcoxon signed-rank test, n = 10–12 pairs). (B) The total duration of social interaction in the intruder assay. (C) The latency to physical interaction in the intruder assay. Welch's ANOVA followed by Dunn’s test; *p < 0.05, **p < 0.01; n = 12–14 per group; mean ± S.E.M.
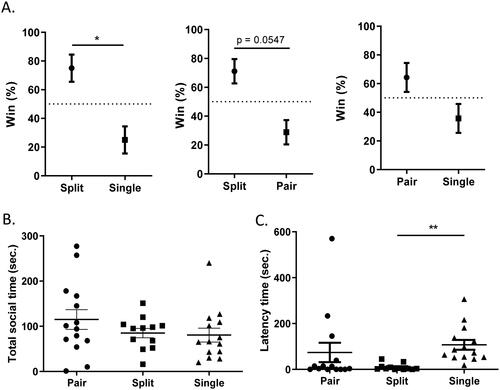
Social behaviors were also examined among these three groups using a standard intruder assay with Balb/c intruders. Although there was no detectable difference in total social time (W(2,23.49) = 0.8887, p = 0.2168; ), we observed that mice in the split-housed group showed a significantly shorter latency to interact with intruders than those in the single-housed group to interact with intruders (W(2,18.13) = 10.74, p = 0.0008; ), implying stronger social motivation after physical isolation.
Physical isolation increased IL-6 in the hippocampus
Neuroinflammation in the hippocampus has been suggested as one of the mechanisms causing anxiety (Calcia et al., Citation2016). To investigate potential inflammation in the hippocampus, mice were sacrificed after the behavioral assay (21 weeks old) for quantification of genes related to inflammation among these three groups (Figure S1). Our results indicated that there were higher expression levels of the cytokine IL-6 and Allograft inflammatory factor 1 (Iba-1) in split-housed mice than in pair-housed mice (IL-6: W(2,13.61) = 5.325, p = 0.0196; Iba-1: W(2,10.67) = 4.868, p = 0.0315) (). Since the relationship between cytokines, especially IL-6, and anxiety has been largely studied not only in the hippocampus but also in the serum (Hodes et al., Citation2016; Ting et al., Citation2020), we further applied a multiplex cytokine assay to measure serum cytokine levels and found a trend for higher IL-6 levels in split-housed mice than in single-housed mice (W(2,17.9) = 2.408, p = 0.1186; ). Unexpectedly, the analysis also showed lower levels of IL-17A in split-housed mice than in single-housed mice (IL-17A: W(2,17.98) = 3.284, p = 0.0609) ( and Table S2).
Figure 6. Expression of the cytokine IL-6 was increased in split-housed mice. (A) Expression levels of the cytokines IL-6 and Iba-1 in the hippocampus (n = 8–10 per group). (B) Serum concentrations of the cytokines IL-6 and IL-17A. (n = 10 per group). Welch's ANOVA followed by Dunn’s test; *p < 0.05; Mean ± S.E.M.
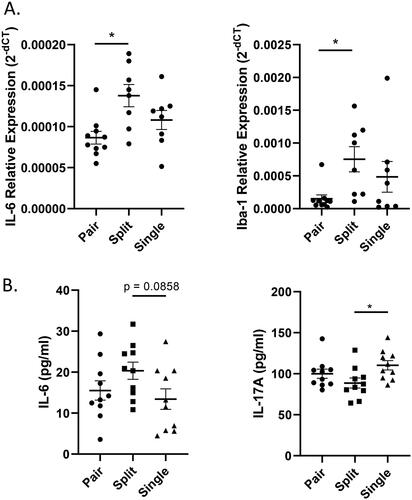
Discussion
Male mice are known to be territorial and do not share space with other males in their natural environment (Kappel et al., Citation2017). Our long-term observation under laboratory conditions, however, demonstrated that male mice with the option of having their own space actually preferred to stay close to each other most of the time. Although this result may not be surprising, these data present a new quantitative evidence to demonstrate a preference for social contact in mice and imply the important role of physical interaction in their daily lives.
In most behavioral assays, we did not detect a significant difference between single- and pair-housed mice, partially due to higher variability in single-housed mice. These results, however, are not surprising since the influence of social isolation on anxiety- or depression-like behaviors of mice is still controversial in the literature. Although most reports preferred to emphasize the disadvantages of social isolation (Mumtaz et al., Citation2018), data showing no effect have been reported in several studies. The differing results can be found with the same testing assays, same genetic background, and similar ages or isolation times (Bibancos et al., Citation2007; Hohlbaum et al., Citation2020; Pietropaolo et al., Citation2008Ieraci et al., 2016; Rodgers & Cole, Citation1993; Voikar et al., Citation2005), suggesting that the inconsistencies are probably caused by subtle differences in the experimental design or a combination of multiple factors. Moreover, social isolation has been shown to lessen the stress from social hierarchy in rats and mice (Nyuyki et al., Citation2012; Singewald et al., Citation2009). The relationship between hierarchy and stress has also been inconsistent across different studies (Varholick et al., Citation2020). Although social defeat has been widely used as a model of stress on subordinate mice (Golden et al., Citation2011), some studies have also indicated higher anxiety in dominant animals (Larrieu et al., Citation2017). Given such a complex dynamic in mouse social structure, the impact of social isolation on behaviors and physiology probably cannot be easily generalized or addressed.
Although there was no phenotypic distinctions in single-housed mice, our experiments based on the open field test and the light-dark box test still detected increased anxiety-like behaviors in split-housed mice. Consistent with a previous study (Larrieu et al., Citation2017), split-housed mice with increased anxiety also showed higher social dominance as defined by the tube test. The most surprising finding was that, in contrast to previous reports (Pais et al., Citation2019; Pietropaolo et al., Citation2008), split-housing caused even higher levels of anxiety-like behaviors than single-housing. These results implied that sensing other social signals without direct physical interaction may produce an even more stressful environment than complete social isolation. For example, animals may release threat messages in visual, auditory or even olfactory forms, which can normally be alleviated by tactile interaction. Alternatively, since other sensory cues may stimulate strong motivation for social interaction, which is supported by the reduced social latency obtained with split-housed mice, the restriction of physical contact could potentially cause more undesirable pressure or stress. Although our data demonstrated that social touch is critical in regulating behavioral performance and maintaining normal health, the question of how social signals other than touch can cause harmful influences remains to be explored.
Neuroinflammation involving the cytokine IL-6 has been reported to induce anxiety in both mice and humans (Calcia et al., Citation2016; Ting et al., Citation2020). Our qPCR results showed higher levels of IL-6 in the mouse hippocampus under split-housing conditions. Although the differences were only close to significance between split- and single-housed mice in the serum, the overall trend supported our observations in the behavioral experiments. Surprisingly, the multiplex assay also detected reduced levels of IL-17A in the split-housed groups. IL-17A is generally believed to be positively correlated with anxiety levels (Alves de Lima et al., Citation2020; Vieira et al., Citation2010), which is contrary to our results. Our data from serum, therefore, only partially support the potential role of cytokines in the increased level of anxiety in the split-housed group of mice.
Although the physical isolation experiment suggested that deprivation of social touch could significantly increase anxiety-like behaviors in mice, a few limitations of our experimental designs should be noted. First, our divider in the split-housing condition may not completely block social touch. Although we did not observe any physical contact between the two mice during our handling, the hole (0.5 cm in diameter) on the divider still provides the possibility for limited interaction through paws or noses. Indeed, in several comparisons, split-housed mice looked more similar to pair-housed mice. We expect that finer design of the divider to completely prevent their interactions could probably strengthen the effects of physical isolation and further enhance the phenotypes. Second, although we separated each behavioral assay by at least 48-h intervals, potential carryover effects from one test to the next were still possible. Unfortunately, complete elimination of these effects will require one set of mice used for only one assay, which is not recommended by the 3 R policy (Replacement, Reduction and Refinement) in animal welfare guidelines. However, since mice in the three housing conditions were all under the same test procedures, we hope that any carryover effect on behavioral performance has been excluded.
Last, this study was conducted with male mice only. Due to higher aggression in male mice, most research related to social isolation has mainly focused on males for animal welfare reasons (Bartolomucci et al., Citation2005; Kappel et al., Citation2017). Studies from the female perspective are thus underrepresented in this field. In addition, it is generally believed that the complexity of the estrus cycle makes female behaviors more variable (Palanza et al., Citation2001; Walf & Frye, Citation2006). However, one systematic review of the existing literature suggested that variability was not significantly greater in females than males in behavioral and physiological traits (Prendergast et al., Citation2014). An extension of this research to female mice should therefore be conducted in future behavioral studies. Interestingly, one previous report compared female postoperative recovery under single housing, group housing and housing with a grid partitions (Van Loo et al., Citation2007), and suggested that mice experiencing physical separation (with a grid partition) showed the worst recovery, which is similar to what we found in this study. In future research, investigating the influence of physical isolation on female mouse behaviors would further enhance the translational significance of our current study.
Initially inspired by physical distancing policies during the pandemic, our study applied long-term observation and manipulations of housing conditions to show that physical touch plays a significant role in daily interactions and functions as a crucial environmental factor for the maintenance of normal behaviors in mice. Although the specific characteristics of social interactions are very different between mice and humans, this study reminds us of the important role of physical contact in our communication. After all, social touch is not merely about an emotional connection; it could also have a substantial impact on an individual’s mental health.
Methods
Mice
C57BL/6J and BALB/cByJ 8-week old male mice were purchased from the National Laboratory Animal Center in Taiwan. Mice were housed in a controlled animal room with a 12-h light/dark cycle (0700–1900 hr). With the exception of the long-term observations, all behavioral tests were conducted during the light period. All animal procedures were in compliance with institutional guidelines established and approved by the Institutional Animal Care and Use Committee of National Tsing Hua University.
Long-term observation
Two random mice were paired-housed in a standard cage and moved to the behavioral room for habituation. After 24 h, the cage was placed under a video camera for recording from above for another 24 h. The videos were analyzed manually using Behavioral Observation Research Interactive Software (BORIS) (Friard & Gamba, Citation2016). According to the mouse activity and physical contact, the behaviors were classified into six categories: both moving-contact, both moving-no contact, single moving-contact, single moving-no contact, no moving-contact, and no moving-no contact ().
Isolation housing conditions
After three weeks of habituation, mice were randomly allocated into three housing conditions: (a) pair-housed cage; (b) split-housed cage; and (c) single-housed cage (). Mice in split-housed cages were separated by a transparent plate (0.5 cm thick) to minimize physical contact. Multiple 0.5-cm diameter holes on the plates allowed for the free exchange of olfactory, visual, and auditory cues. After one month of housing under different conditions, the mice were tested in multiple behavioral assays in sequence (). There was at least a 48-h interval between each assay to minimize the carryover effects on behaviors. Mice were returned to their experimental housing conditions after each assay.
Open field test
The open field test was used to evaluate anxiety-like behavior (Seibenhener & Wooten, Citation2015). A mouse was placed into a clean 50 cm × 50 cm open field apparatus for 6 min with overhead video recording. Videos were evaluated using SMART VIDEO TRACKING Software (Panlab) to determine the total distance moved as a measure of activity and total time in the center of the box (25 cm × 25 cm) as a measure of the anxiety level.
Light-dark box assay
The light-dark box assay was used to evaluate anxiety-like behavior (Takao & Miyakawa, Citation2006). The light-dark box was made of opaque Plexiglas (50 cm × 25 cm white open chamber for the light box, 50 cm × 25 cm × 30 cm black closed chamber for the dark box). The chambers were connected by a 5.5 cm × 4.5 cm door in the middle of the wall separating the two chambers. Animals were placed in the middle of the light chamber and free to explore for 6 min. Videos were evaluated using SMART VIDEO TRACKING Software (Panlab) to determine the total time in the light box as a measure of the anxiety level.
Standard forced swimming test
The forced swimming test is an antidepressant screening test for measuring motor reactivity to the stress associated with forced swimming (Can et al., Citation2012). The container [11.5 cm (R)× 17.5 cm (H)] was filled with water such that the animals could neither step on the bottom nor escape from the top. When the mice were placed in the container, the behavior was recorded by a digital camera for 6 min. After the test, the mice were removed from the water and dried with an infrared lamp in their home cage. Videos were analyzed by SMART VIDEO TRACKING Software (Panlab) for the last 5 min to avoid unstable immobility behavior in the first minute. Floating was defined as immobility behavior or minimal movement for maintaining balance in the water. All mice were first time swimmers, and no mice were used for multiple forced swimming test.
Tail suspension test
The tail suspension test is an antidepressant screening test for measuring motor reactivity to the stress of the tail suspension (Steru et al., Citation1985). The end of the mouse tail was fastened by tape on a bar 45 cm above the desk, allowing the mice to freely struggle and wave their limbs. The behavior was recorded by a digital camera for 6 min and analyzed for the last 5 min by SMART VIDEO TRACKING Software (Panlab) to avoid unstable immobility behavior in the first minute. Immobility behavior was defined as any passive behavior including slight swaying. All mice were tested only once, and no mice were used for multiple tail suspension tests.
Tube test
The tube test, in which one mouse forces its opponent backward out of a tube, was used to evaluate mouse social dominance (Chou et al., Citation2021). A clear Plexiglas tube (3.75-cm diameter, 60-cm length) was used for the test. Two mice were simultaneously released at opposite ends of the tube and then ran toward the middle. Since the size of the tube was not large enough for mice to crossover or reverse their direction, one of the mice would eventually move backward. When the mouse retreated and set all four paws outside the tube, the test trial was over, and that mouse was considered the loser. The mouse still in the tube was identified as the winner. The win rates between two mice were based on four consecutive trials, with each mouse starting at an alternative end of the tube for each trial.
Intruder assay
The intruder assay was used to investigate mouse social interaction. Although this assay has mostly been used for the study of aggression (Kaur et al., Citation2014), we recorded and incorporated all forms of social behaviors, including aggression, allogrooming and general social investigation, as social interactions. BALB/cByJ males were used as intruders. To minimize intruder aggression, the olfaction of the intruders had been ablated by injections of 2,6-dichlorobenzonitrile (dichlorobenil, Sigma D57558) at a concentration of 50 mg/mL and a dose of 100 ug/g of body weight every other day until three injections were administered. In this assay, one intruder was introduced into the home cage of a resident male for 10 min. For split- and pair-housed groups, cagemates and dividers were removed before the assay. The behaviors were digitally recorded and analyzed to obtain the latency time and total time for social interactions.
RNA extraction, cDNA synthesis, and quantitative real-time PCR
Mice at 21 weeks old (10 weeks in different housing conditions) were anesthetized with isoflurane (Panions & BF Biotech Inc.). Then, the mice were sacrificed by decapitation. The brain was rapidly removed, and the hippocampi were dissected for RNA extraction. Total RNA was extracted using the RNeasy Mini Kit (Qiagen), including a DNase (Qiagen) treatment. cDNA of mRNA was generated by ReverTra Ace Set RT cDNA Synthesis Mix (Purigo) using oligo dT as primer. The qPCRs were performed under a qTOWER³ real-time PCR system (Analytik Jena) using the KAPA SYBR FAST Universal qPCR Kit (KAPA Biosystems, MA) following the manufacture’s protocol. The relative expression was calculated using the ΔCT method and GAPDH was used as a normalization control. The primer sequences used for qPCR are shown in Table S1.
Measurement of serum cytokines
Mouse blood was collected into a blood collection tube (BD Vacutainer) from the jugular vein and then centrifuged at 1,000×g for 10 min. The plasma was stored at −80 °C until analyzed. The cytokine levels in serum were measured by a Bio-Plex Mouse cytokine Group I 23-plex assay kit (Bio-Rad Laboratories, Hercules, CA) according to the manufacturer’s instructions. The measured molecules included eotaxin, G-CSF, GM-CSF, IFN-γ, IL-1α, IL-1β, IL-2, IL-3, IL-4, IL-5, IL-6, IL-9, IL-10, IL-12 (p40), IL-12 (p70), IL-13, IL-17A, KC, MCP-1 (MCAF), MIP-1α, MIP-1β, RANTES and TNF-α. In brief, 50 µl of the serum sample was incubated with 50 µl of washed antibody-coupled beads in each well of a flat-bottom plate for 30 min at room temperature. After washing to remove unbound materials, the beads were incubated with 25 µl biotinylated detection antibodies for 30 min. After washing away the unbound biotinylated antibodies, the beads were incubated with 50 µl streptavidin-PE for 10 min at room temperature. The beads were then resuspended in 125 µl assay buffer and read on the Bio-Plex suspension array system. The data were analyzed using Bio-Plex Manager software version 6.0.
Statistics
All statistics were performed using GraphPad Prism 8.0 software. The long-term observations were based on three pairs of mice and examined by Kruskal–Wallis test followed by Dunnett's test. Other multiple comparisons were examined by Welch’s ANOVA followed by Dunnett's test. Pairwise comparisons in the tube test were tested by the Wilcoxon signed-rank test. For behavioral assays, there were 14 mice in the pair-housed group, 12 mice in the split-housed group, and 14 mice in the single-housed group. For gene expression in the hippocampus by qPCR, there were 10 mice in the pair-housed group, and 8 mice in the split-housed and single-housed groups. For the cytokine levels in serum by multiplex assay, there were 10 mice in each group. All data are represented as the mean ± standard error of the mean (S.E.M.).
Ethics approval and consent to participate
All animal procedures were in compliance with institutional guidelines established and approved by the Institutional Animal Care and Use Committee of National Tsing Hua University.
Author contributions
K-T Lin, and T-H Kuo designed the experiments; Y-K Ma, C-L Lee, Y-H Chu, C-H Chen, and Y-S Su performed the experiments; Y-K Ma, P-Y Zeng, Y-H Chu, and C-C Cheng analyzed the data; K-T Lin and T-H Kuo wrote the manuscript.
Supplemental Material
Download MP4 Video (55.5 MB)Acknowledgements
The authors thank the members of the Kuo and Lin labs for experimental help. We also thank National Yang Ming Chiao Tung University for the mouse facility.
Disclosure statement
The authors declare no competing financial interests.
Data availability statement
All data generated or analyzed during this study are included in this published article and its supplementary information files.
Additional information
Funding
References
- Alves de Lima, K., Rustenhoven, J., Da Mesquita, S., Wall, M., Salvador, A. F., Smirnov, I., Martelossi Cebinelli, G., Mamuladze, T., Baker, W., Papadopoulos, Z., Lopes, M. B., Cao, W. S., Xie, X. S., Herz, J., & Kipnis, J. (2020). Meningeal γδ T cells regulate anxiety-like behavior via IL-17a signaling in neurons. Nature Immunology, 21(11), 1421–1429. https://doi.org/10.1038/s41590-020-0776-4
- Bartolomucci, A., Palanza, P., Sacerdote, P., Panerai, A. E., Sgoifo, A., Dantzer, R., & Parmigiani, S. (2005). Social factors and individual vulnerability to chronic stress exposure. Neuroscience and Biobehavioral Reviews, 29(1), 67–81. https://doi.org/10.1016/j.neubiorev.2004.06.009
- Bibancos, T., Jardim, D. L., Aneas, I., & Chiavegatto, S. (2007). Social isolation and expression of serotonergic neurotransmission-related genes in several brain areas of male mice. Genes, Brain, and Behavior, 6(6), 529–539. https://doi.org/10.1111/j.1601-183X.2006.00280.x
- Cacioppo, J. T., Cacioppo, S., Capitanio, J. P., & Cole, S. W. (2015). The neuroendocrinology of social isolation. Annual Review of Psychology, 66, 733–767. https://doi.org/10.1146/annurev-psych-010814-015240
- Calcia, M. A., Bonsall, D. R., Bloomfield, P. S., Selvaraj, S., Barichello, T., & Howes, O. D. (2016). Stress and neuroinflammation: a systematic review of the effects of stress on microglia and the implications for mental illness. Psychopharmacology, 233(9), 1637–1650. https://doi.org/10.1007/s00213-016-4218-9
- Can, A., Dao, D. T., Arad, M., Terrillion, C. E., Piantadosi, S. C., & Gould, T. D. (2012). The mouse forced swim test. Journal of Visualized Experiments, 59, e3638.
- Chou, Y.-J., Lu, Y.-H., Ma, Y.-K., Su, Y.-S., & Kuo, T.-H. (2021). The decisive role of subordination in social hierarchy in weanling mice and young children. iScience, 24(2), 102073. in press. https://doi.org/10.1016/j.isci.2021.102073
- Friard, O., & Gamba, M. (2016). BORIS: a free, versatile open-source event-logging software for video/audio coding and live observations. Methods in Ecology and Evolution, 7(11), 1325–1330. https://doi.org/10.1111/2041-210X.12584
- Golden, S. A., Covington, H. E., Berton, O., & Russo, S. J. (2011). A standardized protocol for repeated social defeat stress in mice. Nature Protocols, 6(8), 1183–1191. https://doi.org/10.1038/nprot.2011.361
- Hodes, G. E., Ménard, C., & Russo, S. J. (2016). Integrating Interleukin-6 into depression diagnosis and treatment. Neurobiology of Stress, 4, 15–22. https://doi.org/10.1016/j.ynstr.2016.03.003
- Hohlbaum, K., Frahm, S., Rex, A., Palme, R., Thone-Reineke, C., & Ullmann, K. (2020). Social enrichment by separated pair housing of male C57BL/6JRj mice. Scientific Reports, 10(1), 11165. https://doi.org/10.1038/s41598-020-67902-w
- Ieraci, A., Mallei, A., & Popoli, M. (2016). Social isolation stress induces anxious-depressive-like behavior and alterations of neuroplasticity-related genes in adult male mice. Neural Plasticity, 2016, 6212983. https://doi.org/10.1155/2016/6212983
- Kamal, A., Ramakers, G. M. J., Altinbilek, B., & Kas, M. J. H. (2014). Social isolation stress reduces hippocampal long-term potentiation: effect of animal strain and involvement of glucocorticoid receptors. Neuroscience, 256, 262–270. https://doi.org/10.1016/j.neuroscience.2013.10.016
- Kappel, S., Hawkins, P., & Mendl, M. T. (2017). To group or not to group? Good practice for housing male laboratory mice. Animals, 7(12), 88. https://doi.org/10.3390/ani7120088
- Kaur, A. W., Ackels, T., Kuo, T.-H., Cichy, A., Dey, S., Hays, C., Kateri, M., Logan, D. W., Marton, T. F., Spehr, M., & Stowers, L. (2014). Murine pheromone proteins constitute a context-dependent combinatorial code governing multiple social behaviors. Cell, 157(3), 676–688. https://doi.org/10.1016/j.cell.2014.02.025
- Larrieu, T., Cherix, A., Duque, A., Rodrigues, J., Lei, H., Gruetter, R., & Sandi, C. (2017). Hierarchical status predicts behavioral vulnerability and nucleus accumbens metabolic profile following chronic social defeat stress. Current Biology, 27(14), 2202–2210 e4. https://doi.org/10.1016/j.cub.2017.06.027
- Lopez, M. F., & Laber, K. (2015). Impact of social isolation and enriched environment during adolescence on voluntary ethanol intake and anxiety in C57BL/6J mice. Physiology & Behavior, 148, 151–156. https://doi.org/10.1016/j.physbeh.2014.11.012
- Mumtaz, F., Khan, M. I., Zubair, M., & Dehpour, A. R. (2018). Neurobiology and consequences of social isolation stress in animal model-A comprehensive review. Biomedicine & Pharmacotherapy = Biomedecine & Pharmacotherapie, 105, 1205–1222. https://doi.org/10.1016/j.biopha.2018.05.086
- Mumtaz, F., Khan, M. I., Zubair, M., & Dehpour, A. R. (2018). Neurobiology and consequences of social isolation stress in animal model-A comprehensive review. Biomedicine & Pharmacotherapy = Biomedecine & Pharmacotherapie, 105, 1205–1222. https://doi.org/10.1016/j.biopha.2018.05.086
- Nyuyki, K. D., Beiderbeck, D. I., Lukas, M., Neumann, I. D., & Reber, S. O. (2012). Chronic subordinate colony housing (CSC) as a model of chronic psychosocial stress in male rats. PLoS One, 7(12), e52371. https://doi.org/10.1371/journal.pone.0052371
- Pais, A. B., Pais, A. C., Elmisurati, G., Park, S. H., Miles, M. F., & Wolstenholme, J. T. (2019). A novel neighbor housing environment enhances social interaction and rescues cognitive deficits from social isolation in adolescence. Brain Sciences, 9(12), 336. https://doi.org/10.3390/brainsci9120336
- Palanza, P., Gioiosa, L., & Parmigiani, S. (2001). Social stress in mice: gender differences and effects of estrous cycle and social dominance. Physiology & Behavior, 73(3), 411–420. https://doi.org/10.1016/s0031-9384(01)00494-2
- Pietropaolo, S., Feldon, J., & Yee, B. K. (2008). Nonphysical contact between cagemates alleviates the social isolation syndrome in C57BL/6 male mice. Behavioral Neuroscience, 122(3), 505–515. https://doi.org/10.1037/0735-7044.122.3.505
- Prendergast, B. J., Onishi, K. G., & Zucker, I. (2014). Female mice liberated for inclusion in neuroscience and biomedical research. Neuroscience and Biobehavioral Reviews, 40, 1–5. https://doi.org/10.1016/j.neubiorev.2014.01.001
- Robb, C. E., de Jager, C. A., Ahmadi-Abhari, S., Giannakopoulou, P., Udeh-Momoh, C., McKeand, J., Price, G., Car, J., Majeed, A., Ward, H., & Middleton, L. (2020). Associations of social isolation with anxiety and depression during the early COVID-19 pandemic: a survey of older adults in London. Frontiers in Psychiatry, 11, 591120.
- Rodgers, R. J., & Cole, J. C. (1993). Influence of social isolation, gender, strain, and prior novelty on plus-maze behaviour in mice. Physiology & Behavior, 54(4), 729–736. https://doi.org/10.1016/0031-9384(93)90084-s
- Seibenhener, M. L., & Wooten, M. C. (2015). Use of the open field maze to measure locomotor and anxiety-like behavior in mice. Journal of Visualized Experiments, (96), e52434. https://doi.org/10.3791/52434
- Silva, C. F., Duarte, F. S., De Lima, T. C. M., & de Oliveira, C. L. (2011). Effects of social isolation and enriched environment on behavior of adult Swiss mice do not require hippocampal neurogenesis. Behavioural Brain Research, 225(1), 85–90. https://doi.org/10.1016/j.bbr.2011.07.007
- Singewald, G. M., Nguyen, N. K., Neumann, I. D., Singewald, N., & Reber, S. O. (2009). Effect of chronic psychosocial stress-induced by subordinate colony (CSC) housing on brain neuronal activity patterns in mice. Stress, 12(1), 58–69. https://doi.org/10.1080/10253890802042082
- Steru, L., Chermat, R., Thierry, B., & Simon, P. (1985). The tail suspension test: a new method for screening antidepressants in mice. Psychopharmacology, 85(3), 367–370. https://doi.org/10.1007/BF00428203
- Takao, K., & Miyakawa, T. (2006). Light/dark transition test for mice. Journal of Visualized Experiments, (1), e104.
- Ting, E. Y.-C., Yang, A. C., & Tsai, S.-J. (2020). Role of interleukin-6 in depressive disorder. International Journal of Molecular Sciences, 21(6), 2194. https://doi.org/10.3390/ijms21062194
- Trout, D. L. (1980). The role of social isolation in suicide. Suicide & Life-Threatening Behavior, 10(1), 10–23. https://doi.org/10.1111/j.1943-278x.1980.tb00693.x
- Van Loo, P. L. P., Kuin, N., Sommer, R., Avsaroglu, H., Pham, T., & Baumans, V. (2007). Impact of 'living apart together' on postoperative recovery of mice compared with social and individual housing. Laboratory Animals, 41(4), 441–455. https://doi.org/10.1258/002367707782314328
- Varholick, J. A., Bailoo, J. D., Jenkins, A., Voelkl, B., & Wurbel, H. (2020). A systematic review and meta-analysis of the relationship between social dominance status and common behavioral phenotypes in male laboratory mice. Frontiers in Behavioral Neuroscience, 14, 624036. https://doi.org/10.3389/fnbeh.2020.624036
- Vieira, M. M. M., Ferreira, T. B., Pacheco, P. A. F., Barros, P. O., Almeida, C. R. M., Araújo-Lima, C. F., Silva-Filho, R. G., Hygino, J., Andrade, R. M., Linhares, U. C., Andrade, A. F. B., & Bento, C. A. M. (2010). Enhanced Th17 phenotype in individuals with generalized anxiety disorder. Journal of Neuroimmunology, 229(1-2), 212–218. https://doi.org/10.1016/j.jneuroim.2010.07.018
- Voikar, V., Polus, A., Vasar, E., & Rauvala, H. (2005). Long-term individual housing in C57BL/6J and DBA/2 mice: assessment of behavioral consequences. Genes, Brain, and Behavior, 4(4), 240–252. https://doi.org/10.1111/j.1601-183X.2004.00106.x
- Walf, A. A., & Frye, C. A. A. (2006). A review and update of mechanisms of estrogen in the hippocampus and amygdala for anxiety and depression behavior. Neuropsychopharmacology : official Publication of the American College of Neuropsychopharmacology, 31(6), 1097–1111. https://doi.org/10.1038/sj.npp.1301067
- White, C. N., VanderDrift, L. E., & Heffernan, K. S. (2015). Social isolation, cognitive decline, and cardiovascular disease risk. Current Opinion in Psychology, 5, 18–23. https://doi.org/10.1016/j.copsyc.2015.03.005