Abstract
Neurovascular coupling ensures rapid and precise delivery of O2 and nutrients to active brain regions. Chronic stress is known to disturb neurovascular signaling with grave effects on brain integrity. We hypothesized that stress-induced neurovascular disturbances depend on stress susceptibility. Wistar male rats were exposed to 8 weeks of chronic mild stress. Stressed rats with anhedonia-like behavior and with preserved hedonic state were identified from voluntary sucrose consumption. In brain slices from nonstressed, anhedonic, and hedonic rats, neurons and astrocytes showed similar intracellular Ca2+ responses to neuronal excitation. Parenchymal arterioles in brain slices from nonstressed, anhedonic, and hedonic rats showed vasodilation in response to neuronal excitation. This vasodilation was dependent on inward rectifying K+ channel (Kir2) activation. In hedonic rats, this vasodilation was transient and followed by vasoconstriction insensitive to Kir2 channel inhibition with 100 µM BaCl2. Isolated arteries from hedonic rats showed increased contractility. Elevation of bath K+ relaxed isolated middle cerebral arteries in a concentration-dependent and Kir2-dependent manner. The vasorelaxation to 20–24 mM K+ was reduced in arteries from hedonic rats. The expression of voltage-gated K+ channels, Kv7.4, was reduced in the cerebral arteries from hedonic rats, whereas the expression of arterial inward-rectifying K+ channels, Kir2.1 was similar to that of nonstressed and anhedonic rats. We propose that preserved hedonic state is associated with increased arterial contractility caused by reduced hyperpolarizing contribution of Kv7.4 channels leading to biphasic cerebrovascular responses to neuronal excitation. These findings reveal a novel potential coping mechanism associated with altered neurovascular signaling.
Introduction
Chronic stress is among the most important risk factors for a variety of psychiatric disorders including depression (de Kloet et al., Citation2005). Some subjects exposed to chronic stress are, nevertheless, able to cope with stressful conditions (Bergstrom et al., Citation2008). However, the mechanism behind the ability to cope with stress is poorly understood. It has been proposed that disturbance in the communication between neuronal tissue and the vasculature, i.e. neurovascular signaling, plays a central role in the pathogenesis of psychiatric manifestations of stress (Longden et al., Citation2014). Neurovascular signaling ensures rapid and precise delivery of O2 and nutrients in addition to the removal of waste products from active brain regions (Longden et al., Citation2016). Neurovascular signaling is believed to be mediated through the release of vasoactive substances, e.g. K+ ions, from active neuronal tissue. The elevation of K+ in the perivascular space activates vascular inward-rectifying K+ channels (Kir2) leading to smooth muscle cell hyperpolarization, vasodilation, and thus increased blood flow to active brain regions (Longden et al., Citation2016). On the other hand, the elevation of interstitial K+ has an overall depolarizing action that counterbalances Kir2-mediated hyperpolarization (Jackson, Citation2018). Therefore, interstitial K+ elevation has a narrow window where arteries dilate prior to vasoconstriction induced by further K+ elevation (Girouard et al., Citation2010). This K+-induced vasoconstriction depends on voltage-gated K+ channels (Jackson, Citation2018). Under physiological conditions, interstitial K+ does not raise to the concentrations that might constrict vascular wall as this will have devastating consequences for active neuronal tissue.
Neurovascular signaling has been shown to be modified upon psychological stress (Han et al., Citation2019; Longden et al., Citation2014). However, these previous studies did not question whether neurovascular changes were associated with variable stress susceptibility. Stress susceptibility can be assessed in a rodent model of chronic mild stress (CMS) based on their preference for sucrose consumption (Willner, Citation2017). In this CMS model, rodents are exposed to unpredictable stressors and develop symptoms similar to depression (Strekalova et al., Citation2022; Wiborg, Citation2013; Willner, Citation2017), such as impaired physical activity and inability to experience pleasure from normally pleasurable stimuli (anhedonia). Hence, rats exhibiting anhedonia-like behavior show a reduction in the preference for consumption of palatable sucrose solution over water. The model is considered to be realistic and with a high validity (Willner, Citation2017). In the CMS model, anhedonia-like symptoms are detected in approximately 30% of stressed rats, while another 30% of rats show less than 10% reduction in the preference of sucrose intake, i.e., preserve their hedonic state (Wiborg, Citation2013). Rats with preserved hedonic state are normally characterized with behavior, biochemical parameters, histological, and molecular changes in the brain that are more similar with the non-stressed rats than with rats showing anhedonia-like symptoms (Strekalova et al., Citation2022; Wiborg, Citation2013; Willner, Citation2017).
In the present study, we hypothesized that chronic mild stress affects neurovascular signaling differently in subjects developing anhedonia-like behavior compared with subjects that preserve their hedonic state upon CMS exposure. We used the conventional CMS protocol with unpredictable stressors and assessed rats' hedonic state using sucrose preference test (Wiborg, Citation2013). We tested the hypothesis by comparing neurovascular signaling in brain slices from hedonic and anhedonic stressed rats and from nonstressed controls. Cerebrovascular function and expression of K+ channels important for neurovascular signaling were assessed in isolated middle cerebral arteries.
Methods
All animal experiments conformed to guidelines from the European Communities Council Directive (86/609/EEC) for the Protection of Animals used for Experimental and other Scientific Purposes; and were conducted with permission from the Animal Experiments Inspectorate of the Danish Ministry of Environment and Food.
Experimental design
CMS protocol and sucrose preference test
Male Wistar rats, 6 weeks old, were purchased from Taconic, Denmark, and housed individually in 12-h light/dark cycle. Food and water were available ad libitum. All rats were singly housed. The standard conditions were changed only in the course of the stress regime. Upon arrival to the animal facility, all rats were adapted and trained to consume a palatable sucrose solution prior CMS protocol was initiated (Bouzinova et al., Citation2014). During this period, the sucrose test was made twice a week during the first three weeks and once a week during the last two weeks. Rats were food and water deprived 14 h prior the test and then exposed to a bottle with sucrose solution (1.5%) for 1 h (Wiborg, Citation2013). Five weeks later, when rats were 11 weeks old, the CMS protocol was initiated. During the CMS period, the sucrose consumption test was performed once a week.
Rats were randomly sub-divided into a group exposed to CMS and a nonstressed control group. The rats from nonstressed group were housed in animal facility room different from the one, where the CMS protocol was applied. The experimental CMS protocol was applied and continued for 8 weeks, as described previously (Matchkov et al., Citation2015). The stress protocol consisted of 12-h periods of intermittent illumination, stroboscopic light, grouping, food or water deprivation, 45° box tilting, soiled cage, and periods of no stress. Grouping was done by housing rats in pairs with different partners alternately being a resident or intruder. All procedures are well established and validated by the research group (Wiborg, Citation2013).
During the first four weeks of the stress protocol, some rats (approximately 20%) did not change their sucrose consumption more than 10% and were considered hedonic, while the rats with a reduction in sucrose intake exceeding 30% (approximately 30% of rats exposed to CMS) were characterized as the group with anhedonia-like behavior. Rats with 10–30% reduction in sucrose intake were excluded from the study. In total, 14 nonstressed, 11 hedonic rats, and 16 rats with anhedonia-like behavior were distributed between in situ brain slice studies and the experiments for ex vivo assessment of middle cerebral artery function.
Brain slice experiments
At the end of the 8-week CMS paradigm, rats were euthanized by decapitation under pentobarbital anesthesia (0.75 mg/kg, i.p.; Euthasol vet., Virbac, Denmark). The brain was quickly moved into ice-cold artificial cerebrospinal fluid (aCSF) aerated with 95% O2 and 5% CO2. The cerebral cortex was cut into 160 µm slices using vibratome (1200 s, Leica Biosystems, Germany) and incubated for 90 min in 5.33 mg/L Calcium Green AM (ThermoFisher Scientific, MA, USA) diluted into aCSF. The slices were continuously superfused with aerated aCSF at 37 °C and examined by simultaneous contrast and fluorescence microscopy (Zeiss LSM 5 Pascal, Germany) as described previously (Staehr et al., Citation2020).
Slices were pretreated with thromboxane A2 analog, U46619 (10 nM) for 30 min prior to the experiment in order to achieve sufficient vascular tone. Neurons were stimulated with electric field stimulation (32 Hz, 14 V, 0.3 ms pulses, stimulation period 3 s) using two platinum electrodes. A baseline period before stimulation (15 s) was used for calculation of diameter changes, i.e. [diameter] /[mean baseline diameter].
Ex vivo studies on isolated middle cerebral arteries
Middle cerebral arteries were cleaned of connective tissue and mounted in a wire myograph (Danish Myo Technology A/S, Denmark) for isometric force measurements (Staehr et al., Citation2020). Active force (mN) was recorded with a PowerLab 4/25, Chart7 acquisition system (ADInstruments Ltd., New Zealand) and converted to wall tension by dividing the force with two times the arterial segment length. Middle cerebral arteries were constricted with a thromboxane A2 receptor agonist, U46619. The U46619-preconstricted arteries were challenged with an increased bath concentration of K+ ions that substituted Na+ ions in the physiological salt solution (PSS).
Western blot
Lysate of middle cerebral arteries was centrifuged at 10,000g to collect the supernatant that was adjusted 10:3:3 with 1 M/L DTT and 2× Tris-glycine SDS sample buffer (Thermo Fisher Scientific, Denmark). The expression of Kir2.1 channel protein was semi-quantified as described previously (Staehr et al., Citation2020). The membrane above 25 kDa was incubated with primary antibody against Kir2.1 (1:200; sc-18708; Santa Cruz Biotechnology, TX, USA), and the lower part was incubated with antibody against thioredoxin-2 (1:10,000; ab185544; Abcam, UK) overnight at 5 °C. The next day, the membranes were incubated with horseradish peroxidase (HRP)-conjugated secondary antibody (1:5000; Dako, Denmark) for 1 h. Bound antibody was detected by an enhanced chemiluminescence (ECL) kit (Sigma Aldrich, Denmark). Detected protein was semi-quantified (ImageJ, NIH, MD, USA) relative to thioredoxin-2 in the same probe. The Kir2.1 expression in nonstressed controls was taken as 100%.
The voltage-gated potassium channel subunit Kv7.4 was semi-quantified by loading 15 mg of middle cerebral artery lysate to 4–20% precast polyacrylamide stain-free gels (CriterionTM TGX Stain-freeTM precast gel, BioRad, CA, USA). The proteins were electrotransferred to nitrocellulose membranes that were blocked in 5% bovine serum albumin (BSA) with 0.5% v/v Tween 20. The membrane was incubated overnight at 5 °C with Kv7.4 antibody (monoclonal, 1:1000 in 3% BSA; S43-6, Thermo Fisher Scientific Inc., MA, USA). After washing, the membranes were incubated with HRP-conjugated secondary antibody. Bound antibody was detected by an ECL kit and normalized using ImageJ software as a ratio to total protein load measured for the same probe.
Solutions
PSS contained (in mM) NaCl 115.8, KCl 2.82, KH2PO4 1.18, MgSO4 1.2, NaHCO3 25, CaCl2 1.6, EDTA 0.03, glucose 5.5, gassed with 5% CO2 in air and adjusted to pH 7.4. aCSF contained (in mM) NaCl 125, KCl 3, NaHCO3 25.9, NaH2PO4 1.25, MgCl2 1, CaCl2 2, L-ascorbic acid 0.4, glucose 4, gassed with 95% O2 and 5% CO2 and adjusted to pH 7.4. Lysis solution contained (in mM) Tris-HCl 10, sucrose 250, EDTA 1, EGTA 1, Triton X-100 2%, pH 7.4; and one tablet protease inhibitor per 10 mL.
Statistical analyses
GraphPad Prism software (v.7.03) was used for graphing and statistical analysis. Data are summarized as the mean value ± standard error of mean (SEM) of the sample group. Contractile concentration–response curves were fitted to experimental data using four-parameter, nonlinear regression curve fitting. From these curves, pD2 (–log to the concentration required to produce a half-maximal response) and maximal response were derived and compared using an extra sum-of-squares F test. Ex vivo K+ induced relaxation curves were drawn with centered third order polynomial function. Significant differences between means of other values were determined by either one-way or two-way ANOVA, where appropriate, followed by correction for multiple comparison as indicated. A probability (p) level of <.05 was considered significant.
Results
Stressed rats with unchanged hedonic state exhibited biphasic neurovascular responses
After 8 weeks of exposure to CMS, the hedonic state of rats was identified based on sucrose consumption test, and hedonic and anhedonic groups were selected for further experiments (). We compared these two groups to matching nonstressed rats. Rats were weighed every week prior and during CMS protocol (). There was a significant increase in weight over time for all the three experimental groups (F(3.049, 115.9) = 217.2, p<.001); however, this was not significantly different between the groups (F(2,38) = 1.290, p=.287).
Figure 1. Based on the sucrose preference test, the stressed hedonic (n = 11) and anhedonic rats (n = 16) were identified and compared to nonstressed rats (n = 14) (A). Anhedonia-like behavior was defined as >30% reduction in sucrose consumption compared with baseline (horizontal dotted black line). A significant differences between groups (F(2, 38) = 41.24, p<.0001) and over time (F(4.88, 183.60) = 3.43, p=.006) was seen. The hedonic group did not differ in sucrose index from the non-stressed group, whereas the group with anhedonia-like behavior had a significantly reduced sucrose index after CMS. There was no significant difference in weight changes over time between the groups (B). *p<.01 and ***p<.001 for the anhedonia-like group vs. nonstressed controls; †p < .05, ††p<.01, and †††p<.001 for the anhedonia-like group vs. the hedonic group (ANOVA followed by Tukey correction for multiple comparison).
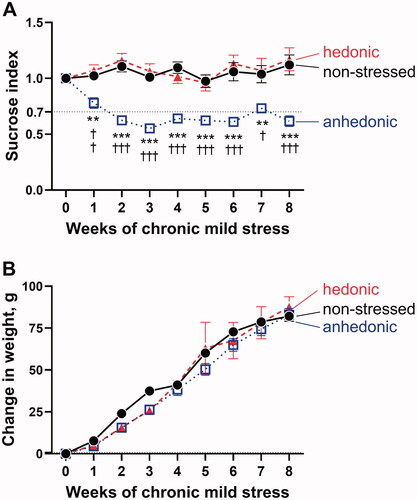
In the brain slices, we assessed intracellular Ca2+ in neuronal tissue and astrocytes, and neurovascular responses of parenchymal arterioles in brain slices (). The arteriole baseline diameter was similar (F(2, 15)=0.421, p=.664) in all the three groups after pre-constriction with 10 nM U46619 to achieve a basal tone of approximately 80% of fully relaxed diameter (). The relaxed diameter was also similar among all the three experimental groups (F(2, 15)=0.751, p=.489) as it was evident after the exposure of brain slices to a Ca2+-free aCSF solution ().
Figure 2. Assessment of neurovascular signaling in brain slices revealed biphasic neurovascular responses in stressed rats with unchanged hedonic state. Representative image of a Calcium Green AM-loaded brain slice from a nonstressed rat shows the neuronal tissue (black tilted arrow) and astrocytic endfood (white tilted arrow) located in a proximity of the adjacent parenchymal arteriole (horizotal arrow indicates arteriole lumen) (A). Brain slices were incubated with 10 nM U46619 for 30 min to pre-constrict parenchymal arterioles to the diameter of approximately 80% of maximally relaxed diameter. Arterioles of the three experimental groups were similar in diameter after pre-constriction with U46619 (B). The fully relaxed arteriole diameter measured in Ca2+-free artificial cerebral spinal fluid was also similar in all the three groups (C). Electric field stimulation (EFS, indicated with vertical dashed lines) of the neuronal tissue in brain slices was associated with vasodilation of parenchymal arterioles in nonstressed (n = 6), hedonic (n = 5) and anhedonic rats (n = 7) (D). In hedonic rats, the initial vasodilation was followed by vasoconstriction. Superfusion of the brain slices with 100 µM BaCl2 strongly diminished neurovascular responses in all groups, but the subsequent vasoconstriction was still observed in hedonic group (E). There was no difference in BaCl2-sensitive vasodilation among the three experimental groups (F). All the three groups showed similar increase in intracellular Ca2 in the neuronal tissue (G) and in astrocytic endfeet (H) in response to EFS. Two nonstressed brain slices and three slices from the anhedonia-like rats were excluded from Ca2+ astrocytic signaling analyses as no astrocytes were identified in the field of view. The data in (B), (C), and (F) were compared with one-way ANOVA; the data in (D), (E), (G), and (H) were compared with two-way ANOVA. **p < .01 and ***p<.001, respectively.
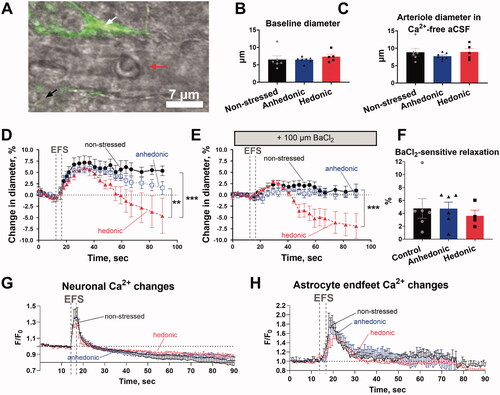
Electric field stimulation (EFS) was associated with similar vasodilation of parenchymal arterioles in all the three groups (). However, the initial vasodilation of arterioles in the brain slices from hedonic rats was followed by vasoconstriction, making their vascular response to EFS different from that of nonstressed rats and rats with anhedonia-like behavior (F(50,375) = 2.499, p<.001). The EFS-induced vasodilation was strongly diminished by 100 µM BaCl2 in all the three experimental groups (). This BaCl2-sensitive vasodilation was similar in all the three experimental groups (F(2, 13) = 0.229, p = .798) (). Importantly, delayed vasoconstriction in response to EFS in the brain slices from hedonic rats remained in the presence of BaCl2 () and was not different in the presence and absence of BaCl2 (F(25, 175) = 1.102, p = .345). Thus, the significant difference in EFS responses of hedonic rats in comparison with nonstressed rats and rats with anhedonia-like behavior remained in the presence of BaCl2 (F(50, 325) = 5.479, p < .001) ().
Neuronal excitation was associated with a similar (F(238, 1663) = 0.926, p = .773) increase in intracellular Ca2+ in the neuronal tissue in all the three experimental groups (). The neuronal Ca2+ response was followed by an increase in intracellular Ca2+ in astrocytic endfeet (), which was also similar in all the three groups (F(238, 1172) = 0.651, p = .999). Importantly, we have previously reported (Staehr et al., Citation2020) that incubation of brain slices with BaCl2 does not affect transient elevation of [Ca2+]i in neuronal tissue and astrocytic endfeet in response to EFS. This suggests that the effect of BaCl2 is of vascular origin.
Cerebral arteries from hedonic rats showed increased contractility and biphasic response to elevated concentration of K+ in the bath solution
Isolated middle cerebral arteries from hedonic rats showed stronger contractile responses to increasing concentrations of U46619 than arteries from nonstressed rats (F(3, 162) = 6.986, p = .0002) (). U46619 also constricted the arteries from anhedonia-like rats stronger than arteries from nonstressed rats (F(3, 198) = 3.819, p = .011). Pre-constricted middle cerebral arteries were relaxed by elevation of bath K+ concentration and this relaxation was similar between all the three experimental groups at 6–18 mM K+ concentrations (F(12, 120) = 0.813, p = .637) (). However, at concentrations above 18 mM of bath K+, the relaxation of arteries from hedonic rats declined while the relaxation of arteries from nonstressed and anhedonia-like rats sustained. At 20 mM of bath K+, there was a significant difference between responses of arteries from hedonic and anhedonic rats (q = 4.045, 95% CI [–78.60, –1.10], p = .0442). At 24 mM of bath K+, there was a difference between responses of arteries from hedonic rats and from both nonstressed (q = 3.978, 95% CI [–49.20, −0.88], p = .0423) and anhedonic rats (q = 4.783, 95% CI [–54.89, –6.89], p = .0121) (). BaCl2 (100 µM) abolished K+-induced relaxation in all groups (F(12,114) = 0.986, p = .467) (). At 24 mM of bath K+ and in the presence of BaCl2, arteries from hedonic rats had significantly higher wall tension in comparison with arteries from nonstressed (q = 3.388, 95% CI [–27.38, –0.19], p = .0460) and anhedonia-like rats (q = 3.405, 95% CI [–23.91, –0.23], p = .0446).
Figure 3. Middle cerebral arteries from stressed rats with unchanged hedonic state exhibited increased arterial contractility. Middle cerebral arteries from hedonic (n = 6) and anhedonic (n = 9) rats showed increased constriction to U46619 compared with nonstressed control rats (n = 8) (A). Middle cerebral arteries pre-constricted with 10 nM U46619 from nonstressed (n = 6), hedonic (n = 9), and anhedonic groups (n = 6) relaxed in response to the elevation of bath K+ concentration up to 18 mM (B). At 20 mM and 24 mM of bath K+, the arteries from hedonic rats showed a decline in vasorelaxation, whereas arteries from anhedonic and nonstressed rats remained relaxed. The K+-induced relaxation was inhibited by 100 µM BaCl2; but at 24 mM bath K+, arteries from hedonic rats constricted (C). The concentration–response curves in (A) were compared with an extra sum-of-squares F test; the responses in (B) and (C) were analyzed with two-way ANOVA followed by Tukey correction for multiple comparison. *p < .05 and **p< .01 for nonstressed vs. hedonic rats; †p < .05 for anhedonia-like vs. nonstressed rats; +p < .05 for anhedonia-like vs. hedonic rats.
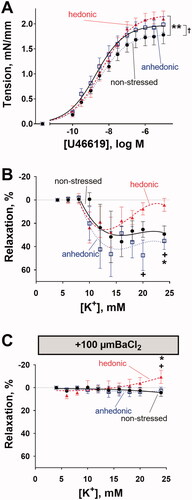
Reduced expression of Kv7.4 in cerebral arteries from hedonic rats
We analyzed the expression of Kir2.1 and K+ voltage-gated channel protein Kv7.4 channels in the middle cerebral artery. No difference in the expression of Kir2.1 channels was found between the groups (F(1.715, 10.29) = 0.984, p = .394) ()). However, the expression of Kv7.4 was significantly decreased in the arteries from hedonic rats in comparison with arteries from nonstressed rats (q = 2.554, 95% CI [1.62, 56.450], p = .0375) ()). No significant difference between hedonic and anhedonic groups was achieved (q = 1.433, 95% CI [–48.37, 12.32], sp = .2911).
Figure 4. Cerebral arteries from stressed rats with unchanged hedonic state had reduced expression of Kv7.4 channels but unchanged Kir2.1 expression. Lysate of middle cerebral arteries was blotted for the Kir2.1 channel expression with thioredoxin as a loading control. Representative blots of Kir2.1 and thioredoxin from the same lanes (A); the vertical white space between the bands indicates that irrelevant lanes from the same film are not shown. No difference in relative Kir2.1 expression was seen between middle cerebral arteries from nonstressed (n = 6), hedonic (n = 4), and anhedonic rats (n = 5) (B). Representative blots for Kv7.4 protein detection and total protein load as identified with stain-free gel loading control. The expression of Kv7.4 protein was reduced in arteries from hedonic rats (n = 7) in comparison with nonstressed rats (n = 9). *p < .05 for nonstressed vs. resilient rats (one-way ANOVA followed by Dunnett correction for multiple comparison).
Cerebral arteries from stressed rats with unchanged hedonic state had reduced expression of Kv7.4 channels but unchanged Kir2.1 expression.
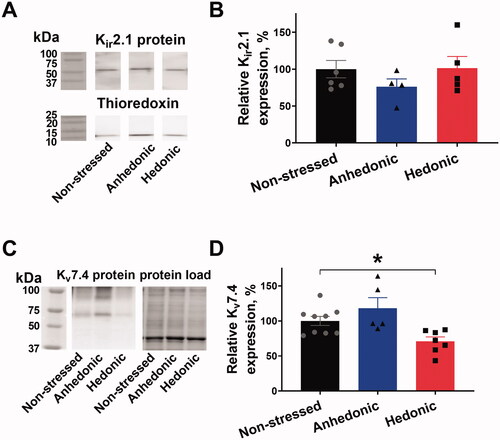
Discussion
In this study, we suggest that unchanged hedonic state of rats after exposure to the CMS protocol is associated with biphasic cerebrovascular responses to neuronal excitation caused by reduced expression of cerebrovascular Kv7.4 channels. We observed different individual responses to stress measured as a sucrose consumption of outbred rats, which is presumably caused by genetic variations. Similar to results of other reports (Bergstrom et al., Citation2008), approximately 20% of rats did not change their hedonic state. We found that neurovascular signaling was reduced in hedonic rats. While the hyperemic response was sustained one minute after neuronal excitation in brains from rats exhibiting anhedonia-like behavior and nonstressed rats, parenchymal arterioles of hedonic rats constricted after initial transient vasorelaxation. Since neuronal and astrocytic Ca2+ dynamics in response to neuronal excitation were similar, we suggest that the changes in neurovascular signaling are caused by different vascular properties. Our data suggest that reduced expression of Kv7.4 channels in cerebral arteries from hedonic rats may be responsible for altered neurovascular signaling.
Neurovascular coupling is essential for brain integrity (Longden et al., Citation2016). It has been shown to be modified by psychological stress in humans (Elbau et al., Citation2018) and suggested to be diminished upon stress in rodents (Han et al., Citation2019; Lee et al., Citation2015; Longden et al., Citation2014). The present study questions whether changes in neurovascular signaling are associated with variable stress susceptibility. In accordance with other reports, we found that vasodilation in response to neuronal activity was BaCl2-sensitive, suggesting the involvement of Kir2.1 channels (Longden et al., Citation2016). However, the amplitude of BaCl2-sensitive vasodilation and the expression of Kir2.1 channels were similar in all the three experimental groups, suggesting that this channel is not responsible for the observed difference in neurovascular signaling. This is in contrast to a previous study by Longden et al. (Citation2014) where chronic stress led to reduced cerebrovascular Kir2-dependent signaling. This previous study suggested that stress causes a glucocorticoid-mediated decrease in functional Kir2 channels and thereby suppresses neurovascular signaling (Longden et al., Citation2014). We have previously shown a similar corticosterone level after 8 weeks of CMS in nonstressed and stress-exposed groups, suggesting a functional habituation of the hypothalamic–pituitary–adrenal axis to incessant stress regardless of hedonic status (Christiansen et al., Citation2012). This argues against glucocorticoid-mediated changes in Kir2 channels in the present study. A different duration of stress exposure and different stress paradigms may therefore explain the discrepancy between our results and those of the previous study (Longden et al., Citation2014).
The voltage-gated K+ channel, Kv7.4 has previously been shown to be one of the most abundant K+ channels in rat middle cerebral artery (Zhong et al., Citation2010). The Kv7.4 channels were proposed to play an essential role in the control of cerebrovascular resistance and cerebral blood flow (Chadha et al., Citation2014). Activation of these channels hyperpolarizes cerebrovascular smooth cells, while inhibition of the channels depolarizes and constricts middle cerebral arteries (Zhong et al., Citation2010). We found a significantly reduced cerebrovascular expression of Kv7.4 channels and increased contractile properties of middle cerebral arteries from hedonic rats. Interestingly, this is not the first time an association between chronic stress and reduced expression of Kv7 channels has been observed. A recent study based on RNA sequencing and pharmacological intervention suggested that Kv7 membrane conductance and expression in the brain was reduced following chronic stress-induced brain injury (Ren et al., Citation2021).
It remains to be studied whether the association of reduced Kv7.4 channel expression and stress susceptibility is caused by an original intersubjective variability or is a result of stress-mediated expression changes. Circulating angiotensin II level that increases during stress (Yang et al., Citation1996) has recently been shown to increase the degradation of vascular Kv7.4 channels (Barrese et al., Citation2018). Whether angiotensin II modifies stress response through its regulation of vascular Kv7.4 channel expression remains, however, to be tested.
Suppression of neurovascular signaling, as we observed in hedonic rats, will diminish hyperemic responses in the brain (Longden et al., Citation2016). Reduced ability to match blood supply to neuronal metabolic demand might have detrimental impact on neuronal survival and brain integrity (Iadecola, Citation2017). Hence, hedonic rats exposed to CMS may suffer from neurological deterioration over time (Carrier et al., Citation2021). The acceleratory effects of stress, including cerebrovascular changes, have previously been shown to be associated with the progression of major depression, anxiety, posttraumatic stress disorder, stroke, dementia, and Alzheimer’s disease (Johansson et al., Citation2010; Rissman et al., Citation2012). This suggests that stress-induced neurovascular changes may be an important modulatory factor in these pathologies. The finding of an association between decreased expression of Kv7.4 channels and reduced neurovascular signaling in stressed subjects with unchanged hedonic state provides novel knowledge on the mechanisms underlying the ability to cope with stress and how stress susceptibility may be modified.
Acknowledgments
We thank Jane Holbaek Roenn for technical assistance with Western blot analysis.
Disclosure statement
No potential conflict of interest was reported by the author(s).
Additional information
Funding
Notes on contributors
Christian Staehr
Christian Staehr, M.D. recently graduated as a medical doctor from Aarhus University (2021). In his PhD, he studies brain blood flow and neurovascular coupling in health and disease. His research suggests that restoration of balanced neurovascular coupling may be a novel treatment target in numerous neurological disorders.
Elena V. Bouzinova
Elena Bouzinova has PhD in neuroscience from Lomonosov Moscow State University (1998) and PhD in membrane transport from Aarhus University (2007). She is studying the devastating effects of chronic stress and neuronal dysfunction behind it in various animal models.
Ove Wiborg
Ove Wiborg, PhD from Aarhus University (1989). He is an Associate Professor at Aalborg University and has his research interest in neurobiology of Major Depression, Memory Loss, Alzheimer's Disease, and Dementia.
Vladimir V. Matchkov
Vladimir Matchkov has his PhD from Lomonosov Moscow State University (1998) and DMSc from Aarhus University (2010). Since 2010, he is an Associate Professor at Aarhus University, Health. His research addresses the comorbidity of neuronal disorders and disturbances in cerebral perfusion, with a focus of dysbalanced neurovascular coupling signaling.
References
- Barrese, V., Stott, J. B., Figueiredo, H. B., Aubdool, A. A., Hobbs, A. J., Jepps, T. A., McNeish, A. J., & Greenwood, I. A. (2018). Angiotensin II promotes KV7.4 channels degradation through reduced interaction with HSP90 (Heat Shock Protein 90). Hypertension, 71(6), 227–1100. https://doi.org/10.1161/HYPERTENSIONAHA.118.11116
- Bergstrom, A., Jayatissa, M. N., Mork, A., & Wiborg, O. (2008). Stress sensitivity and resilience in the chronic mild stress rat model of depression; an in situ hybridization study. Brain Research, 1196, 41–52. https://doi.org/10.1016/j.brainres.2007.12.025
- Bouzinova, E. V., Norregaard, R., Boedtkjer, D. M., Razgovorova, I. A., Moeller, A. M., Kudryavtseva, O., Wiborg, O., Aalkjaer, C., & Matchkov, V. V. (2014). Association between endothelial dysfunction and depression-like symptoms in chronic mild stress model of depression. Psychosomatic Medicine, 76(4), 268–276. https://doi.org/10.1097/PSY.0000000000000062
- Carrier, M., Simoncicova, E., St-Pierre, M. K., McKee, C., & Tremblay, M. E. (2021). Psychological stress as a risk factor for accelerated cellular aging and cognitive decline: The involvement of Microglia-Neuron crosstalk. Frontiers in Molecular Neuroscience, 14, 749737. https://doi.org/10.3389/fnmol.2021.749737
- Chadha, P. S., Jepps, T. A., Carr, G., Stott, J. B., Zhu, H. L., Cole, W. C., & Greenwood, I. A. (2014). Contribution of kv7.4/kv7.5 heteromers to intrinsic and calcitonin gene-related peptide-induced cerebral reactivity. Arteriosclerosis, Thrombosis, and Vascular Biology, 34(4), 887–893. https://doi.org/10.1161/ATVBAHA.114.303405
- Christiansen, S., Bouzinova, E. V., Palme, R., & Wiborg, O. (2012). Circadian activity of the hypothalamic pituitary adrenal axis is differentially affected in the rat chronic mild stress model of depression. Stress, 15(6), 647–657. https://doi.org/10.3109/10253890.2011.654370
- de Kloet, E. R., Joels, M., & Holsboer, F. (2005). Stress and the brain: From adaptation to disease. Nature Reviews. Neuroscience, 6(6), 463–475. https://doi.org/10.1038/nrn1683
- Elbau, I. G., Brucklmeier, B., Uhr, M., Arloth, J., Czamara, D., Spoormaker, V. I., Czisch, M., Stephan, K. E., Binder, E. B., & Samann, P. G. (2018). The brain’s hemodynamic response function rapidly changes under acute psychosocial stress in association with genetic and endocrine stress response markers. Proceedings of the National Academy of Sciences of the United States of America, 115(43), E10206–E10215.
- Girouard, H., Bonev, A. D., Hannah, R. M., Meredith, A., Aldrich, R. W., & Nelson, M. T. (2010). Astrocytic endfoot Ca2+ and BK channels determine both arteriolar dilation and constriction. Proceedings of the National Academy of Sciences of the United States of America, 107(8), 3811–3816. https://doi.org/10.1073/pnas.0914722107
- Han, K., Min, J., Lee, M., Kang, B. M., Park, T., Hahn, J., Yei, J., Lee, J., Woo, J., Lee, C. J., Kim, S. G., & Suh, M. (2019). Neurovascular coupling under chronic stress is modified by altered GABAergic interneuron activity. The Journal of Neuroscience, 39(50), 10081–10095. https://doi.org/10.1523/JNEUROSCI.1357-19.2019
- Iadecola, C. (2017). The neurovascular unit coming of age: A journey through neurovascular coupling in health and disease. Neuron, 96(1), 17–42. https://doi.org/10.1016/j.neuron.2017.07.030
- Jackson, W. F. (2018). KV channels and the regulation of vascular smooth muscle tone. Microcirculation, 25(1), e12421. https://doi.org/10.1111/micc.12421
- Johansson, L., Guo, X., Waern, M., Ostling, S., Gustafson, D., Bengtsson, C., & Skoog, I. (2010). Midlife psychological stress and risk of dementia: A 35-year longitudinal population study. Brain, 133(Pt 8), 2217–2224. https://doi.org/10.1093/brain/awq116
- Lee, S., Kang, B. M., Shin, M. K., Min, J., Heo, C., Lee, Y., Baeg, E., & Suh, M. (2015). Chronic stress decreases cerebrovascular responses during rat hindlimb electrical stimulation. Frontiers in Neuroscience, 9, 462.
- Longden, T. A., Dabertrand, F., Hill-Eubanks, D. C., Hammack, S. E., & Nelson, M. T. (2014). Stress-induced glucocorticoid signaling remodels neurovascular coupling through impairment of cerebrovascular inwardly rectifying K + channel function. Proceedings of the National Academy of Sciences of the United States of America, 111(20), 7462–7467. https://doi.org/10.1073/pnas.1401811111
- Longden, T. A., Hill-Eubanks, D. C., & Nelson, M. T. (2016). Ion channel networks in the control of cerebral blood flow. Journal of Cerebral Blood Flow and Metabolism, 36(3), 492–512. https://doi.org/10.1177/0271678X15616138
- Matchkov, V. V., Kravtsova, V. V., Wiborg, O., Aalkjaer, C., & Bouzinova, E. V. (2015). Chronic selective serotonin reuptake inhibition modulates endothelial dysfunction and oxidative state in rat chronic mild stress model of depression. American Journal of Physiology, Regulatory, Integrative and Comparative Physiology, 309(8), R814–R823. https://doi.org/10.1152/ajpregu.00337.2014
- Ren, J., Guo, J., Zhu, S., Wang, Q., Gao, R., Zhao, C., Feng, C., Qin, C., He, Z., Qin, C., Wang, Z., & Zang, L. (2021). The role of potassium channels in chronic stress-induced brain injury. Biological & Pharmaceutical Bulletin, 44(2), 169–180. https://doi.org/10.1248/bpb.b20-00504
- Rissman, R. A., Staup, M. A., Lee, A. R., Justice, N. J., Rice, K. C., Vale, W., & Sawchenko, P. E. (2012). Corticotropin-releasing factor receptor-dependent effects of repeated stress on tau phosphorylation, solubility, and aggregation. Proceedings of the National Academy of Sciences of the United States of America, 109(16), 6277–6282. https://doi.org/10.1073/pnas.1203140109
- Staehr, C., Rajanathan, R., Postnov, D. D., Hangaard, L., Bouzinova, E. V., Lykke-Hartmann, K., Bach, F. W., Sandow, S. L., Aalkjaer, C., & Matchkov, V. V. (2020). Abnormal neurovascular coupling as a cause of excess cerebral vasodilation in familial migraine. Cardiovascular Research, 116(12), 2009–2020. https://doi.org/10.1093/cvr/cvz306
- Strekalova, T., Liu, Y., Kiselev, D., Khairuddin, S., Chiu, J. L. Y., Lam, J., Chan, Y. S., Pavlov, D., Proshin, A., Lesch, K. P., Anthony, D. C., & Lim, L. W. (2022). Chronic mild stress paradigm as a rat model of depression: Facts, artifacts, and future perspectives. Psychopharmacology, 239(3), 663–693. https://doi.org/10.1007/s00213-021-05982-w
- Wiborg, O. (2013). Chronic mild stress for modeling anhedonia. Cell and Tissue Research, 354(1), 155–169. https://doi.org/10.1007/s00441-013-1664-0
- Willner, P. (2017). Reliability of the chronic mild stress model of depression: A user survey. Neurobiology of Stress, 6, 68–77. https://doi.org/10.1016/j.ynstr.2016.08.001
- Willner, P. (2017). The chronic mild stress (CMS) model of depression: History, evaluation and usage. Neurobiology of Stress, 6, 78–93. https://doi.org/10.1016/j.ynstr.2016.08.002
- Yang, G., Wan, Y., & Zhu, Y. (1996). Angiotensin II – an important stress hormone. Biological Signals, 5(1), 1–8. https://doi.org/10.1159/000109168
- Zhong, X. Z., Harhun, M. I., Olesen, S. P., Ohya, S., Moffatt, J. D., Cole, W. C., & Greenwood, I. A. (2010). Participation of KCNQ (Kv7) potassium channels in myogenic control of cerebral arterial diameter. The Journal of Physiology, 588(Pt 17), 3277–3293. https://doi.org/10.1113/jphysiol.2010.192823