Abstract
Childhood cancer survivors have a high risk for premature cardiovascular diseases, mainly due to cardiotoxic cancer treatments such as doxorubicin (DOX). Psychosocial stress is a significant cardiovascular risk factor and an enormous burden in childhood cancer survivors. Although observational studies suggest that psychosocial stress is associated with cardiovascular complications in cancer survivors, there is no translationally relevant animal model to study this interaction. We established a “two-hit” model in which juvenile mice were administered DOX (4 mg/kg/week for 3 weeks), paired to a validated model of chronic subordination stress (CSS) 5 weeks later upon reaching adulthood. Blood pressure, heart rate, and activity were monitored by radio-telemetry. At the end of CSS experiment, cardiac function was assessed by echocardiography. Cardiac fibrosis and inflammation were assessed by histopathologic analysis. Gene expressions of inflammatory and fibrotic markers were determined by PCR. Juvenile exposure to DOX followed by adult-onset CSS caused cardiac fibrosis and inflammation as evident by histopathologic findings and upregulated gene expression of multiple inflammatory and fibrotic markers. Intriguingly, juvenile exposure to DOX blunted CSS-induced hypertension but not CSS-induced tachycardia. There were no significant differences in cardiac function parameters among all groups, but juvenile exposure to DOX abrogated the hypertrophic response to CSS. In conclusion, we established a translationally relevant mouse model of juvenile DOX-induced cardiotoxicity that predisposes to adult-onset stress-induced adverse cardiac remodeling. Psychosocial stress should be taken into consideration in cardiovascular risk stratification of DOX-treated childhood cancer survivors.
Introduction
The 5-year survival rate of children diagnosed with cancer has increased from less than 60% in the 1970s to more than 80% in 2010 (Lipshultz, Diamond, et al., Citation2014; Lipshultz, Sambatakos, et al., Citation2014). Unfortunately, young childhood cancer survivors suffer from several long-term adverse health complications (Ariffin et al., Citation2017; Ness et al., Citation2015; Blaauwbroek et al., Citation2007). In particular, childhood cancer survivors have a considerably increased risk for premature cardiovascular diseases (Faber et al., Citation2018), with an estimated 15 times higher risk of heart failure than their siblings who did not have cancer (Oeffinger et al., Citation2006). Nearly 50% of pediatric cancer patients receive anthracyclines such as doxorubicin (DOX), despite its known cardiotoxic effects (van Dalen et al., Citation2011). Although it has been widely accepted that the risk of anthracycline-induced cardiotoxicity increases with a higher anthracycline cumulative dose (Nysom et al., Citation1998), recent studies have demonstrated that latent cardiotoxicity occurs in children who receive low doses of anthracyclines (Leger et al., Citation2015; Vandecruys et al., Citation2012; Yeh & Vejpongsa, Citation2015). This latent cardiotoxicity predisposes anthracycline-treated survivors to other cardiovascular risk factors later in life in a “two-hit” manner (Armstrong et al., Citation2013). Psychosocial stress, i.e. stress derived from interrelation of social interactions and individual thought and behavior, is a significant cardiovascular risk factor (Rozanski et al., Citation1999; Ford et al., Citation1998; Kivimaki et al., Citation2006; Kamphuis et al., Citation2006) and an enormous burden in up to 63% of childhood cancer survivors (Brinkman et al., Citation2016; Prasad et al., Citation2015; Johannsdottir et al., Citation2017; Tremolada et al., Citation2016; Kuba et al., Citation2019). Although observational studies demonstrate that psychosocial stress is associated with higher rates of cardiovascular complications in cancer survivors (Ehrhardt et al., Citation2018; Schoormans et al., Citation2016; Vuotto et al., Citation2017; Schoormans et al., Citation2017), there is a paucity in translationally relevant animal models that study the intriguing interaction between juvenile exposure to anthracyclines and adult-onset psychosocial stress.
We have recently demonstrated that exposure to low doses of DOX (4 mg/kg/week for 3 weeks) produced no overt cardiac dysfunction in young mice, yet was sufficient to cause modest cardiac atrophy and to induce molecular markers of cardiotoxicity such as atrial natriuretic peptide (ANP) (Matsumura et al., Citation2018; Maayah et al., Citation2021; Abdelgawad et al., Citation2021). Intriguingly, exposure of young mice to these low doses of DOX aggravated the detrimental cardiovascular effects of adult-onset angiotensin II (ANGII)-induced hypertension resulting in overt cardiac dysfunction and severe cardiac fibrosis (Matsumura et al., Citation2018; Maayah et al., Citation2021; Agostinucci et al., Citation2022). We have also developed and validated a chronic subordination stress (CSS) model in which experimental male mice are housed with aggressive males and exposed to daily defeat episodes and chronic sensory housing, allowing a continuous threat of aggression while limiting physical injuries (Bartolomucci et al., Citation2001). Subordinate mice manifest several hallmarks of chronic stress (Razzoli et al., Citation2014, Citation2015; Bartolomucci et al., Citation2004), signifying the validity of the CSS model to recapitulate human psychosocial stress. Intriguingly, we and others have shown that CSS alone caused modest structural and functional cardiovascular effects in experimental animals (Costoli et al., Citation2004; Simas et al., Citation2018; Agrimi et al., Citation2019) and exacerbated the cardiovascular complications when combined with other cardiovascular risk factors such as obesity (Simas et al., Citation2018; Agrimi et al., Citation2019). Therefore, the objective of the current work is to determine how juvenile exposure to DOX may aggravate the cardiovascular response to adult-onset psychosocial stress in a clinically relevant mouse model.
Materials and methods
Animals
Male C57BL/6N mice were purchased from Charles River Laboratories at 4 weeks of age and housed in groups of 4 with free access to food and water during the study period, under 12:12 h light: dark cycle at 22 ± 2 °C. After a one-week acclimation period, mice were randomized to receive either 4 mg/kg/week DOX or equivalent volume of sterile saline by intraperitoneal injection for 3 weeks. CD1 male mice that served in the CSS model as aggressive residents were purchased from Charles River Laboratories and individually housed in static cages (257 mm × 483 mm × 152 mm) to be used for the CSS protocol and provided with food, water, and bedding under the same general environmental conditions as described above. At the end of the experiment, animals were humanely euthanized by decapitation under isoflurane anesthesia. Hearts were harvested, rinsed in phosphate-buffered saline, flash frozen in liquid nitrogen, and stored at −80 °C. All experimental procedures were approved by the Institutional Animal Care and Use Committee (IACUC) of the University of Minnesota (Protocol ID: 1807-36187 A). The number of experimental subjects used for each experiment is indicated in the figure legends.
Chronic subordination stress (CSS)
Experimental animals were exposed to CSS 5 weeks after the last dose of DOX or saline had been administered. The protocol used is a modified version of our standard CSS procedure (Razzoli et al., Citation2014; Bartolomucci et al., Citation2004; Razzoli et al., Citation2020; Bartolomucci et al., Citation2010). Saline- and DOX-exposed mice entered a one-week baseline period before being exposed to daily (between 8 and 10 AM) social defeat exposure by a CD1 mouse for a maximum of 10 min and continuous sensory contact (allowing auditory, olfactory, and visual stimuli but preventing physical interaction) for 14 d (). According to our standard protocol, each experimental mouse was only paired with one CD1 for the entire duration of the study, thus enabling the establishment of a true and stable social hierarchy between the two subjects. During the social interaction, offensive behaviors of the animals were manually recorded and social status was determined by display of upright postures, flight behavior, and squeaking vocalization as previously described (Bartolomucci et al., Citation2010; Dadomo et al., Citation2011). To prevent injuries, mice were continuously monitored and were separated if fights escalated. Mice in the CSS group were housed in large colony boxes (257 mm× 483 mm× 152 mmH; 1241 cm2 of floor space) divided lengthwise in two equally sized compartments (∼620 cm2 each) by a divider consisting of a white plexiglass frame and wire mesh netting; controls were individually housed in standard housing cages (186 mm ×298 mm ×128 mmH; 554 cm2 of floor space) and received daily gentle handling at the same time as the social defeat procedure.
Figure 1. Effect of juvenile exposure to DOX, adult-onset CSS, and their combination on body composition. (A) Experimental design of the two-hit model of latent DOX-induced cardiotoxicity using CSS as a second hit. Male 5-week-old C57BL/6N mice were administered DOX (4 mg/kg/week) or saline for 3 weeks and allowed to recover for 5 weeks prior to exposure to 14 d of CSS. Effect of juvenile exposure to DOX one day prior to CSS on (B) body weight (n = 27–29 per group), (C) Fat mass (n = 12 per group), and (D) fat free mass (n = 12 per group). (E) Average number of attacks received during the daily social defeat interactions in CSS (n = 14). Comparisons between the two groups in (B–E) were performed using unpaired student t-test. (F) Daily body weight gain during CSS where a significant stress × time interaction is found (F(4,208)=5.915, p<.001) and asterisks denote significant binary differences within each time point among groups following Tukey’s HSD post hoc test. (#p=.054301, within saline groups, control vs. CSS; ***p<.001, within DOX groups, control vs. CSS; comparisons at day 14), (G) Total amount of food consumed in the course of CSS (n = 13–15 per group). (H) Delta fat free mass (n = 6 per group), and (I) Delta fat mass (n = 6 per group) following CSS. Values are represented as means ± SEM. Statistical analysis was performed by two-way ANOVA with Tukey’s HSD post-hoc tests as appropriate (*p<.05, **p<.01, ***p<.001, ****p<.0001). Detailed statistical analysis is provided in Supplementary Table 2.
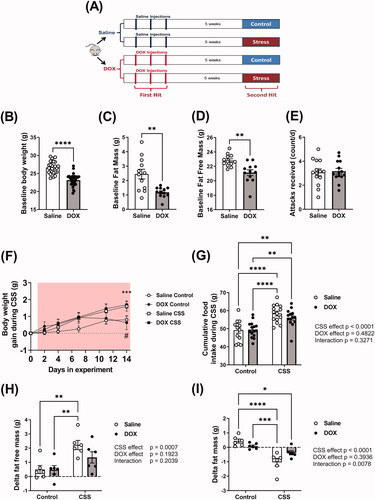
Radio-telemetry for hemodynamic monitoring
A cohort of experimental mice underwent surgery for the implantation of a pressure catheter in the femoral artery attached to a telemetry device (PA-C10; Data Sciences International, Saint Paul, MN) 1 week prior to CSS exposure. Briefly, mice were prepared for surgery under aseptic conditions, and anesthetized with 2–3% isoflurane. The body of the transmitter was implanted in the flank through a small incision. Another incision was made in the groin to expose the femoral artery. The transmitter was inserted in a small nick made in the femoral artery and advanced to the abdominal aorta. A suture was placed around the femoral artery and the catheter to hold it in place. Subsequently, the body of the transmitter was tunneled to the flank and the incisions were closed with 5–0 silk sutures. Following the surgery, mice were individually housed during a 7-d recovery period. Blood pressure, heart rate, and activity were then continuously monitored following the recovery period for the duration of the experiment.
Metabolic study and body composition analysis
Food intake and body weight were measured regularly throughout the experiment. Energy efficiency was calculated from the ratio between the body weight gain and the food consumed. A separate cohort of mice was used for the analysis of body composition. Body composition parameters including fat and fat free mass were measured with EchoMRI 3-in-1 (EchoMRI LLC, Houston, TX) one day before (baseline) and on day 12 of CSS exposure in a subset of mice.
Echocardiography
Cardiac function was assessed by trans-thoracic echocardiography 1 day following the end of the CSS experiment in a subset of mice. Echocardiography was performed using the Vevo 2100 system (VisualSonics, Inc., Toronto, Ontario, Canada) equipped with an MS400 transducer. Anesthesia was induced with 3% isoflurane in oxygen and maintained at 1–2% during the procedure. Mice were secured in a supine position on a heated physiologic monitoring stage. Parasternal short axis images of the left ventricle (LV) were obtained in M-Mode at the level of the papillary muscles. Endocardial and epicardial borders were manually traced over 3–4 cardiac cycles and parameters of cardiac function and morphometry were calculated using VisualSonics cardiac measurement package of the Vevo 2100. Pulsed Doppler images of mitral valve flow were acquired in the apical 4-chamber view and peak velocities of the early (E) and atrial (A) peaks were measured to calculate E/A ratio.
Histopathology
LV heart sections were collected, fixed in 10% neutral buffered formalin, and embedded in paraffin. Four-micron sections were stained with hematoxylin and eosin (HE) or Masson’s trichrome stain. Histopathologic evaluation was performed by a board-certified veterinary pathologist who was blinded to the experimental group. The severity of fibrosis on the Masson’s trichrome stained section was assessed for fibrosis as follows: 0, absent; 1, minimal inflammation or fibrosis; 2, mild inflammation or fibrosis; 3, moderate inflammation or fibrosis; and 4, marked inflammation or fibrosis.
Quantitative real-time PCR
Total RNA was extracted from 20 mg frozen ventricular tissue using 300 µL Trizol reagent (Life Technologies, Carlsbad, CA) according to manufacturer’s instructions. RNA concentrations were calculated by measuring absorbance at 260 nm using a NanoDrop 8000 spectrophotometer (Thermo Fisher Scientific, Wilmington, DE). First-strand cDNA was synthesized from 1.5 µg total RNA using the high-capacity cDNA reverse transcription kit (Applied Biosystems, Foster City, CA) according to manufacturer’s instructions. Specific mRNA expression was quantified by SYBR Green (Applied Biosystems) based real-time PCR performed on an ABI 7900HT instrument (Applied Biosystems) using 384-well optical reaction plates. Thermal cycling parameters were as follows: 95 °C for 10 min, followed by 40 PCR cycles of denaturation at 95 °C for 15 s, and annealing/extension at 60 °C for 1 min. Gene expression was determined using previously published primers for cyclooxygenase-2 (Cox-2), interleukin-6 (IL-6), collagen 1a1 (Col1a1), transforming growth factor-beta1 (TGF-beta1), and galectin-3. Primer sequences are listed in Supplementary Table 1. The mRNA expression levels were normalized to β-actin and are expressed relative to the saline-treated controls. Relative gene expression was determined by the ΔΔCT method, and primer specificity and purity of the final PCR product were verified by melting curve analysis.
Table 1. Echocardiography-derived morphometry parameters after CSS in male C57BL/6N mice pre-exposed to DOX as juveniles. Values are presented as mean ± standard of the mean (SEM).
Statistical analysis
Statistical significance was determined by one- or two-way ANOVA, one- or two-way ANOVA for repeated measures with Tukey’s HSD post-hoc test, or two-way mixed effects ANOVA with Sidaks post hoc test (Wilcox, Citation1987). Unpaired student t-test was used to compare between two groups when appropriate. Data are presented as mean ± SEM. Statistical analyses for histopathologic grading were performed using the non-parametric Kruskal–Wallis followed by Mann–Whitney U tests. A p value of <.05 was taken to indicate statistical significance. Statistica 13.5 (TIBCO Software Inc., Palo Alto, CA), R (Version 1.3.1093 © 2009-2020 RStudio, PBC), and GraphPad Prism (La Jolla, CA) were used for analyses. The specific statistical test used for each experiment is indicated in the figure legends.
Results
Effect of CSS on body weight, food intake, and body composition in saline- and DOX-exposed mice
Juvenile exposure to DOX caused a significant decline in body weight gain during the treatment weeks (Supplementary Figure 1(A)), resulting in a significantly lower body weight than that of saline-injected mice prior to the start of CSS (). The reduction in body weight in DOX-treated mice is attributed to a reduction in both fat mass () and fat free mass (). Five weeks after the last DOX or saline injection, mice were randomized to undergo the 14 d CSS protocol or to serve as controls as described in the Methods section. Juvenile exposure to DOX did not alter the number of attacks received by the CD1 during the aggressive interactions (). In the course of the 14 d of CSS, the body weight gain measured was significantly changed as a function of both time and stress, revealing that stress groups independently from DOX exposure, gained more weight than control mice groups ( and Supplementary Figure 1(B)). CSS had a significant impact on the amount of food ingested by stressed mice, where, independently from DOX exposure, they exhibited a clear hyperphagic response () becoming more pronounced as the CSS progressed and is strengthened by the normalization of amount of food ingested by body weight (Supplementary Figure 1(C)). CSS exposure modestly but significantly increased body weight gain (), an effect that was due to an increase in fat free mass () in presence of decreased fat mass () and % fat mass (Supplementary Figure 1(D)). These metabolic effects were significant in CSS-saline group only (Supplementary Table 2). Due to differences in baseline body weight caused by DOX treatment, % change in body weight was also analyzed. The overall effect mirrors the result with weight gain, although post hoc comparisons are not significant (Supplementary Figure 1(B)). In general, this result is consistent with previous reports on the same model and other strains of mice when mice were fed a standard diet (Sanghez et al., Citation2016; Razzoli et al., Citation2016; Sanghez et al., Citation2013).
Juvenile exposure to DOX blunted CSS-induced hypertension but not CSS-induced tachycardia
Juvenile exposure to DOX did not result in any significant difference in blood pressure measurements compared to saline-treated mice at baseline level (). However, exposure to CSS caused profound cardiovascular changes. In particular, mean arterial pressure (MAP) was found to be significantly elevated in saline-treated but not in DOX-treated mice exposed to CSS (; Supplementary Table 3). This result was reflected in changes in both systolic and diastolic pressure which were found significantly elevated in saline-treated but not in DOX-treated CSS-exposed mice (; Supplementary Table 3). Remarkably, unlike what was observed in MAP, CSS exposure caused significant tachycardia in both saline-treated and DOX-treated mice (; Supplementary Table 3). The same outcome was true for activity level, where CSS induced a similar level of hyperactivity in both saline- and DOX-treated mice compared to controls (; Supplementary Table 3). Finally, we analyzed MAP and heart rate during rest (defined as intervals with <2 activity counts per minute). Results demonstrate that MAP remained significantly elevated at rest in CSS-Saline treated, but not in CSS-DOX treated mice (Supplementary Figure 2(A)). Conversely, heart rate at rest is not increased by CSS (Supplementary Figure 2(B)).
Figure 2. Juvenile exposure to DOX prevented CSS-induced hypertension. Male 5-week-old C57BL/6N mice were administered DOX (4 mg/kg/week) or saline for 3 weeks and allowed to recover for 5 weeks prior to exposure to CSS. Average daily values and average values at baseline and at the end of CSS of (A and B) Mean arterial blood pressure (MAP), (C and D) systolic, and (E and F) diastolic blood pressure recorded by radio telemetry prior to and over the course of CSS (n = 4–8 per group); shaded area on the graphs represents time during CSS protocol. Values are represented as means ± SEM. Statistical analysis was conducted by repeated measures ANOVA followed by Tukey’s HSD post-hoc tests (**p<.01, ***p<.001). Detailed statistical analysis is provided in Supplementary Table 3.
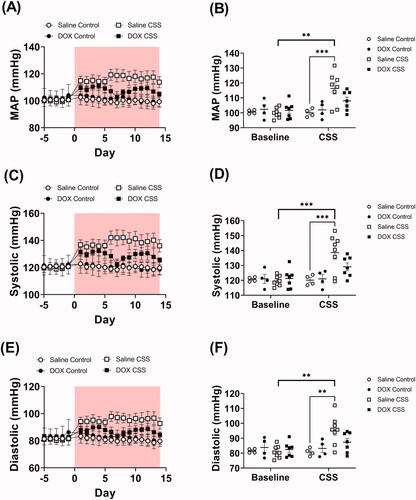
Figure 3. Adult CSS induces tachycardia and increases activity level. Male 5-week-old C57BL/6N mice were administered DOX (4 mg/kg/week) or saline for 3 weeks and allowed to recover for 5 weeks prior to exposure to CSS. Heart rate and locomotor activity data were recorded by radio telemetry. Time course and average values at baseline and at the end of CSS of (A and B) heart rate and (C and D) locomotor activity prior to and during CSS (n = 4–8 per group); shaded area on the graphs represents time during CSS protocol. Values are represented as means ± SEM. Statistical analyses were conducted by repeated measures ANOVA followed by Tukey’s HSD post-hoc tests(**p<.01, ***p<.001). Detailed statistical analysis is provided in Supplementary Table 3.
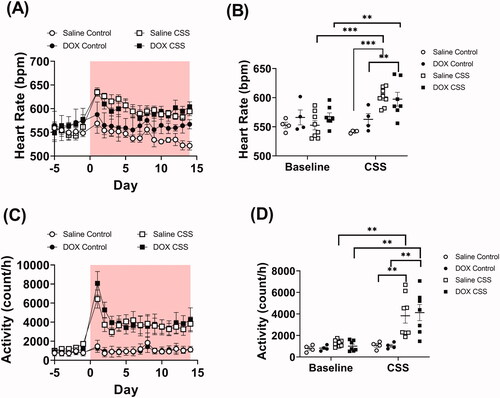
Juvenile exposure to DOX abrogated the hypertrophic response to CSS
Trans-thoracic echocardiography was utilized to determine the effects of juvenile exposure to DOX, adult-onset CSS, and the combination thereof on cardiac function and morphometry. Representative echocardiographic images obtained in M-Mode from each group are displayed in . There were no statistically significant differences in cardiac function parameters among all these groups, as evidenced by no change in ejection fraction, fractional shortening, E/A ratio or cardiac output (; Supplementary Table 4). Nevertheless, 2 weeks of CSS caused cardiac hypertrophy in C57BL/6N mice as evidenced by a significant increase in heart weight to tibia length (HW/TL) (; Supplementary Table 4). Echocardiography-derived morphometric measurements showed a statistically significant effect of DOX exposure to reduce LV mass and a statistically significant effect of CSS to increase LV mass (). CSS-induced increase in LV mass was associated with a significant effect to increase LV posterior wall thickness (). Intriguingly, juvenile exposure to DOX significantly reduced HW/TL and LV mass, thus abrogating CSS-induced cardiac hypertrophy (; ).
Figure 4. Juvenile exposure to DOX abrogated CSS-induced hypertrophy without changes in cardiac function. Male 5-week-old C57BL/6N mice were administered DOX (4 mg/kg/week) or saline for 3 weeks and allowed to recover for 5 weeks prior to exposure to CSS. Cardiac function was assessed by trans-thoracic echocardiography (n = 7–9 per group) at the end of CSS. (A) Representative M-Mode images from parasternal short axis view of the heart, (B) ejection fraction, (C) fractional shortening, (D) E/A ratio, and (E) cardiac output. (F) Heart weight to tibia length ratio (HW/TL) (n = 13–15 per group). Values are represented as means ± SEM. Statistical significance of pairwise comparisons was determined by two-way ANOVA with Tukey’s HSD post-hoc tests (**p<.01, ***p<.001, ****p<.0001). Detailed statistical analysis is provided in Supplementary Table 4.
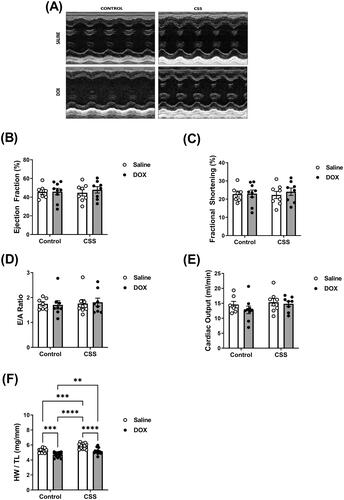
Juvenile exposure to DOX exacerbated CSS-induced myocardial inflammation and fibrosis
Histopathology analysis using HE and Masson’s trichrome stains revealed inflammation and cardiac fibrosis that were more severe and significantly increased in the DOX/CSS group as compared to the saline control (). Although a few mice in the DOX/control and saline/CSS groups also showed signs of cardiac fibrosis, neither DOX nor CSS alone was sufficient to cause a statistically significant effect on cardiac fibrosis (). Consistent with the histopathologic findings, gene expressions of multiple key inflammatory markers and fibrotic markers such as Cox-2 () and IL-6 () were significantly induced in DOX-treated mice following CSS. The IL-6/Cox-2 signaling pathway has been shown to be a key mediator of cardiac inflammation and fibrosis via different mechanisms (Chou et al., Citation2018; Liao et al., Citation2020). Similarly, Col1a1 (), TGF- β1 (), and galectin-3 () were significantly upregulated in DOX-treated mice following CSS (Supplementary Table 5). Col1a1, TGF- β1, and galectin-3 are markers as well as mediators of myocardial fibrosis as reported by several studies (Shen et al., Citation2018). Intriguingly, neither juvenile exposure to DOX nor adult-onset CSS alone resulted in significant upregulation of any of these markers ().
Figure 5. Histopathologic evaluation of myocardial fibrosis and inflammation in hearts from male C57BL/6N mice. Male 5-week old C57BL/6N mice were administered DOX (4 mg/kg/week) or saline for 3 weeks and allowed to recover for 5 weeks prior to exposure to CSS. Representative images from (A) hematoxylin and eosin (HE) and (B) Masson’s trichrome stained heart sections; bar scale = 50 µM. Inflammatory cell infiltration in (A) and fibrotic areas in (B) are indicated with arrows. (C) Semi-quantification of fibrosis score derived from Masson’s trichrome stain (n = 6 per group). Statistical analysis was determined by non-parametric Kruskal–Wallis followed by a Mann–Whitney tests (*p< .05).
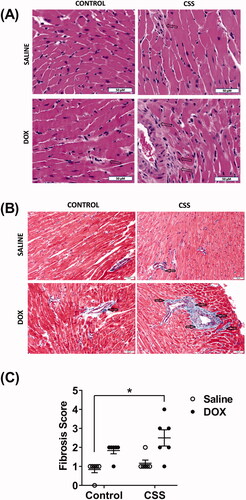
Figure 6. Inflammatory and fibrotic markers are upregulated following CSS in DOX-exposed mice. Male 5-week old C57BL/6N mice were administered DOX (4 mg/kg/week) or saline for 3 weeks and allowed to recover for 5 weeks prior to exposure to CSS. The mRNA expression of inflammatory markers (A) Cyclooxygenase-2 (Cox-2) and (B) Interleukin-6 (IL-6) and the fibrotic markers (C) Collagen 1a1 (Col1a1), (D) Transforming Growth Factor-beta-1 (TGF-beta1), and (E) Galectin-3 was determined by real-time PCR (n = 6 per group). Values are represented as means ± SEM. Statistical significance of pairwise comparisons was determined by two-way ANOVA with Tukey’s HSD post-hoc tests (*p<.05, **p<.01, ***p<.001, ****p<.0001). Detailed statistical analysis is provided in Supplementary Table 5.
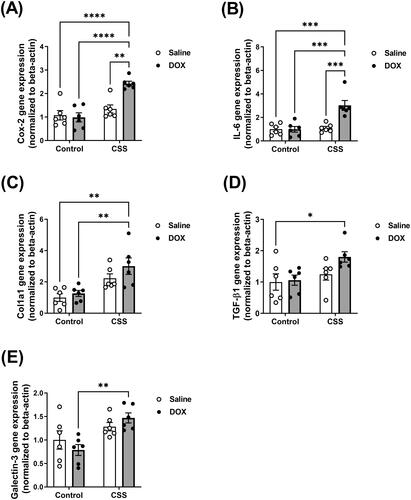
Discussion
It is increasingly recognized that suffering from cancer and undergoing cancer treatment has significant ramifications on patients’ lives (Schoormans et al., Citation2016; Schoormans, Citation2021) with up to 63% of childhood cancer survivors experiencing psychiatric diseases such as anxiety and depression (Brinkman et al., Citation2016; Prasad et al., Citation2015; Johannsdottir et al., Citation2017; Tremolada et al., Citation2016; Kuba et al., Citation2019). Furthermore, anthracycline-treated childhood cancer survivors are already at a higher risk for cardiovascular complications (van Dalen et al., Citation2011). In the general population, chronic psychosocial stress is associated with cardiovascular diseases, independent of other traditional risk factors (Rozanski et al., Citation1999). This led us to hypothesize that juvenile exposure to anthracyclines and adult-onset psychosocial stress could act synergistically to exacerbate cardiovascular complications (Blaes et al., Citation2021). We thus established a “two-hit” model in which young mice were administered DOX (Matsumura et al., Citation2018), paired to a validated model of CSS 5 weeks later upon reaching adulthood. To this end, we adopted our novel mouse model of juvenile DOX-induced latent cardiotoxicity (Matsumura et al., Citation2018), wherein DOX (4 mg/kg/week for 3 weeks) is administered to 5-week-old juvenile C57BL/6N mice (equivalent to ∼10–12 in human years (Dutta & Sengupta, Citation2016)). This dosage regimen is equivalent to a human dose of 40 mg/m2 (Reagan-Shaw et al., Citation2008), which is relevant to clinical doses used to treat hematologic malignancies in pediatric cancer patients (Kelly, Citation2012). Juvenile exposure to DOX caused modest cardiac atrophy, induced the gene expression of ANP a molecular marker of cardiotoxicity, and activated cardiac p38 MAPK (Matsumura et al., Citation2018). Echocardiographic measurements of cardiac function demonstrated that juvenile exposure to DOX had no effect on either systolic or diastolic function parameters (Matsumura et al., Citation2018; Maayah et al., Citation2021; Abdelgawad et al., Citation2021). Therefore, this approach models the clinical scenario of anthracycline-treated pediatric cancer patients who do not develop cardiac dysfunction immediately after completing their cancer treatment, but are left with “weaker” hearts predisposing them to cardiovascular risk factors later in life, in agreement with clinical studies (Leger et al., Citation2015; Tham et al., Citation2013; Çetin et al., Citation2018; Germanakis et al., Citation2006; Yazaki et al., Citation2018). Mice in the CSS model have been shown to develop sustained hypothalamic-pituitary-adrenal-axis activation, one of the hallmarks of chronic stress (Razzoli et al., Citation2014), anxiety and depression-like behavior (Razzoli et al., Citation2015; Bartolomucci et al., Citation2004), and, importantly, cardiovascular changes including tachycardia (Costoli et al., Citation2004; Bartolomucci et al., Citation2003; Carnevali et al., Citation2012). Furthermore, CSS exerts a positive energy balance in mice, caused by robust hyperphagia resulting in weight gain (Razzoli et al., Citation2015, Citation2016; Sanghez et al., Citation2013; Patterson et al., Citation2013). Weight gain in mice fed standard diet is almost entirely driven by increased fat free mass, while fat mass accrual has been consistently reported on mice fed a high fat diet (Razzoli et al., Citation2015, Citation2016; Sanghez et al., Citation2013). Notably, obesity resistance was also shown, if CSS is applied to morbidly diet-induced obese mice (Agrimi et al., Citation2019).
Our lab was the first to report that CSS causes persistent increase in MAP (Razzoli et al., Citation2020). In this study, we replicated CSS-induced hypertension in saline-treated mice. Strikingly, juvenile exposure to DOX abrogated the hypertensive response to CSS. DOX has been shown to lower blood pressure and increase heart rate in an adult rat model of DOX-induced cardiotoxicity (Rabelo et al., Citation2001). In addition, DOX-treated rats were less responsive to increasing doses of the vasoconstrictive drug phenylephrine. Interestingly, these alterations occurred in absence of a significant change in cardiac output (Rabelo et al., Citation2001). In another model, although DOX treatment elevated blood pressure, the vasoconstrictive response to phenylephrine was similarly blunted, implying a reduced cardiovascular reactivity to catecholamines (de Wildt et al., Citation1985). These preclinical observations are supported by clinical evidence of cardiovascular autonomic dysfunction in anthracycline-treated cancer survivors (Teng et al., Citation2021). Intriguingly, in our study, while juvenile DOX blunted the CSS-induced hypertension, it did not blunt the CSS-induced tachycardia. These results suggest that the effects of DOX in this study are mediated by changes in the vascular system, rather than the heart. In contrast to our current finding, we previously reported that juvenile exposure to DOX aggravated adult-onset ANGII-induced hypertension (Matsumura et al., Citation2018), implying that the impact of juvenile DOX exposure on blood pressure depends on the adult-onset hypertensive stimulus. In support of this notion, a recent study has shown that DOX impairs the vascular smooth muscle contractile response to phenylephrine, but not to ANGII (Bosman et al., Citation2021), suggesting the CSS-induced hypertension is likely mediated by catecholamines not ANGII.
We previously demonstrated that low doses of DOX (4 mg/kg/week for 3 weeks) did not cause cardiac dysfunction in young mice (Maayah et al., Citation2021; Abdelgawad et al., Citation2021), yet this regimen was sufficient to exacerbate ANGII-induced cardiac dysfunction (Matsumura et al., Citation2018; Agostinucci et al., Citation2022). The deterioration of cardiac function in DOX/ANGII-treated mice was associated with a significant increase in blood pressure (Matsumura et al., Citation2018). In contrast to these findings, neither DOX treatment, CSS, nor their combination adversely affected the cardiac function in this study. The preserved cardiac function in all experimental groups may be attributed to the observation that juvenile DOX exposure blunted the hypertensive response to CSS in clear contrast to the aggravated increase in blood pressure in the DOX/ANGII group. Interestingly, we have shown that ANGII but not catecholamines worsened cardiac dysfunction and exacerbated myocardial fibrosis following juvenile exposure to DOX (Agostinucci et al., Citation2022). These findings suggest that DOX-induced predisposition to cardiovascular risk factors is highly dependent on the pathologic stimulus imposed by a specific risk factor. There is paucity of studies reporting the effect of CSS on echocardiography-derived cardiac function. In one study, CSS caused modest cardiac dysfunction in healthy male C57BL/6J mice, but more severe cardiac dysfunction was precipitated in obese mice (Agrimi et al., Citation2019). Similarly, CSS did not significantly change cardiac function in wild-type mice, but it caused severe deterioration in cardiac function in mice with arrhythmogenic cardiomyopathy (Agrimi et al., Citation2020).
Anthracycline-induced subclinical cardiotoxicity is commonly associated with cardiac atrophy and thinning of the LV walls in childhood cancer survivors (Lipshultz et al., Citation2002, Citation2012, Citation2013; Mawad et al., Citation2020). We previously demonstrated that juvenile exposure to DOX blunted the adaptive hypertrophic response to both ANGII and isoproterenol in adult mice (Matsumura et al., Citation2018; Agostinucci et al., Citation2022). In this study, we also demonstrate that juvenile exposure to DOX abrogates the hypertrophic response to psychosocial stress, suggesting that exposure to DOX at a young age alters a fundamental mechanism of myocardial growth that prevents the adaptive hypertrophic response to a variety of stimuli. Several mechanisms have been proposed to delineate how DOX interferes with cardiac growth as discussed in (Chen et al., Citation2022). Future research is warranted to investigate the exact mechanism that mediates DOX-induced blunting of the hypertrophic response to CSS. The effect of CSS on features of cardiac hypertrophy has been reported in a few studies only and results are somewhat inconsistent, including no hypertrophy in CD1 mice (Costoli et al., Citation2004) and the lack of echocardiography-derived indices of cardiac hypertrophy in non-obese C57BL/6J mice (Agrimi et al., Citation2019).
In this study, we demonstrate that neither juvenile exposure to DOX nor CSS caused significant cardiac fibrosis in naïve mice, but significant fibrosis was observed when the two hits were combined. The aggravated cardiac fibrosis in the DOX/CSS C57BL/6N mice was associated with a marked upregulation in multiple inflammatory and fibrotic markers including interleukin-6 and cyclooxygenase-2. Mechanistically, our data suggest that a pro-inflammatory state caused by the combined effect of DOX and CSS may have exacerbated cardiac fibrosis. CSS has been shown to cause modest but significant myocardial fibrosis in CD1 mice (Costoli et al., Citation2004), while other studies demonstrated that CSS was not sufficient to cause myocardial fibrosis in healthy mice, but it exacerbated myocardial fibrosis in obese mice (Agrimi et al., Citation2019) and in Dsg2mut/mut mice with arrhythmogenic cardiomyopathy (Agrimi et al., Citation2020). These discrepant results suggest that genetic background changes the individual predisposition to develop cardiac fibrosis in the presence of social stress exposure. The incidence and degree of cardiac fibrosis in anthracycline-treated childhood cancer survivors are also debated. Some studies report modest cardiac fibrosis in anthracycline-treated childhood cancer survivors (Mawad et al., Citation2020; Cheung et al., Citation2015), while other studies demonstrate lack of a statistically significant increase in cardiac fibrosis (Tong et al., Citation2019; Ylanen et al., Citation2013). The discrepancy in these clinical studies may have arisen from the divergent effects of different cardiovascular risk factors on the pathogenesis of latent anthracycline-induced cardiotoxicity in childhood cancer survivors. Therefore, more clinical research is needed to study the possible association between cardiac fibrosis and different cardiovascular risk factors, including psychosocial stress, in anthracycline-treated childhood cancer survivors.
In conclusion, we established, for the first time, a clinically relevant mouse model of juvenile DOX-induced latent cardiotoxicity that predisposes to adult-onset stress-induced adverse cardiac remodeling. Anthracyclines and psychosocial stress adversely affect multiple targets in the body; nevertheless, the cardiovascular system is particularly vulnerable to a detrimental synergy between these two pathologic stimuli. Accordingly, psychosocial stress should be taken into consideration in cardiovascular risk stratification of anthracycline-treated childhood cancer survivors.
Supplemental Material
Download MS Word (150.1 KB)Acknowledgments
Experiments using the NanoDrop 8000 and ABI 7900 HT were done with staff support at the University of Minnesota Genomics Center. Experiments using the Vevo 2100 echocardiography system were done with staff support at the University of Minnesota Imaging Center. Processing of heart tissues for histopathological analysis was performed with staff support at the Comparative Pathology Shared Resource, University of Minnesota Masonic Cancer Center. The stress and radiotelemetry experiments were conducted at the Physiology Core, University of Minnesota. We would like to thank Pilar Ariza for performing the surgeries, and Jan Pierre Pallais for help with the social stress experiments.
Disclosure statement
The authors declare that they do not have any financial or non-financial conflicts of interest concerning this manuscript.
Data availability statement
The data that support the findings of this study are available in the methods and/or supplementary material of this article.
Additional information
Funding
Notes on contributors
Marianne K. O. Grant
Marianne K.O. Grant, BA is a Senior Researcher in the Zordoky lab, University of Minnesota College of Pharmacy. Her current work focuses on cancer therapy-induced cardiovascular complications.
Maria Razzoli
Maria Razzoli, PhD is a Senior Scientist in the Bartolomucci lab and the behavioral/metabolic specialist of the Physiology Core of the University of Minnesota Medical School. Her current work focuses on rodent stress models and induced behavioral and physiological alterations, including stress accelerated aging phenotypes.
Ibrahim Y. Abdelgawad
Ibrahim Y. Abdelgawad, BSc is a PhD Candidate in the Zordoky lab, University of Minnesota College of Pharmacy. His current work focuses on cancer therapy-induced senescence.
Rachel Mansk
Rachel Mansk, BA is a Research Scientist in the Bartolomucci lab and a graduate student at the University of Minnesota School of Public Health. Rachel's research focuses on the social determinants of health.
Davis Seelig
Davis Seelig, DVM, PhD is Associate Professor, Department of Veterinary Clinical Sciences, University of Minnesota College of Veterinary Medicine and Director of the Comparative Pathology Shared Resource at the University of Minnesota Masonic Cancer Center. His research focuses on comparative and experimental pathology.
Alessandro Bartolomucci
Alessandro Bartolomucci, PhD is Professor, Ancel Keys Biomedical Scholar in Physiology and Metabolism, and Director of the Physiology Core at the Department of Integrative Biology and Physiology, University of Minnesota Medical School. His laboratory is primarily interested in metabolic physiology, stress physiology and social determinants of health and aging.
Beshay N. Zordoky
Beshay N. Zordoky, PhD is Associate Professor of Experimental and Clinical Pharmacology, University of Minnesota College of Pharmacy. His current research interests include cancer therapy-induced cardiovascular complications and therapy-induced senescence.
References
- Abdelgawad, I. Y., Grant, M. K. O., Popescu, F. E., Largaespada, D. A., & Zordoky, B. N. (2021). Doxorubicin paradoxically ameliorates tumor-induced inflammation in young mice. International Journal of Molecular Sciences, 22, 9023. https://doi.org/10.3390/ijms22169023
- Agostinucci, K., Grant, M. K. O., Seelig, D., Yucel, D., van Berlo, J., Bartolomucci, A., Dyck, J. R. B., & Zordoky, B. N. (2022). Divergent cardiac effects of angiotensin II and isoproterenol following juvenile exposure to doxorubicin. Frontiers in Cardiovascular Medicine, 9, 742193. https://doi.org/10.3389/fcvm.2022.742193
- Agrimi, J., Scalco, A., Agafonova, J., Williams Iii, L., Pansari, N., Keceli, G., Jun, S., Wang, N., Mastorci, F., Tichnell, C., Murray, B., James, C. A., Calkins, H., Zaglia, T., Paolocci, N., & Chelko, S. P. (2020). Psychosocial stress hastens disease progression and sudden death in mice with arrhythmogenic cardiomyopathy. Journal of Clinical Medicine, 9, 3804. https://doi.org/10.3390/jcm9123804
- Agrimi, J., Spalletti, C., Baroni, C., Keceli, G., Zhu, G., Caragnano, A., Matteucci, M., Chelko, S., Ramirez-Correa, G. A., Bedja, D., Casieri, V., Di Lascio, N., Scalco, A., Beltrami, A. P., Paolocci, N., Caleo, M., & Lionetti, V. (2019). Obese mice exposed to psychosocial stress display cardiac and hippocampal dysfunction associated with local brain-derived neurotrophic factor depletion. EBioMedicine, 47, 384–401. https://doi.org/10.1016/j.ebiom.2019.08.042
- Ariffin, H., Azanan, M. S., Abd Ghafar, S. S., Oh, L., Lau, K. H., Thirunavakarasu, T., Sedan, A., Ibrahim, K., Chan, A., Chin, T. F., Liew, F. F., Jeyamogan, S., Rosli, E. S., Baharudin, R., Yap, T. Y., Skinner, R., Lum, S. H., & Hainaut, P. (2017). Young adult survivors of childhood acute lymphoblastic leukemia show evidence of chronic inflammation and cellular aging. Cancer, 123(21), 4207–4214. https://doi.org/10.1002/cncr.30857
- Armstrong, G. T., Oeffinger, K. C., Chen, Y., Kawashima, T., Yasui, Y., Leisenring, W., Stovall, M., Chow, E. J., Sklar, C. A., Mulrooney, D. A., Mertens, A. C., Border, W., Durand, J. B., Robison, L. L., & Meacham, L. R. (2013). Modifiable risk factors and major cardiac events among adult survivors of childhood cancer. Journal of Clinical Oncology, 31(29), 3673–3680. https://doi.org/10.1200/JCO.2013.49.3205
- Bartolomucci, A., Carola, V., Pascucci, T., Puglisi-Allegra, S., Cabib, S., Lesch, K. P., Parmigiani, S., Palanza, P., & Gross, C. (2010). Increased vulnerability to psychosocial stress in heterozygous serotonin transporter knockout mice. Disease Models & Mechanisms, 3(7–8), 459–470. https://doi.org/10.1242/dmm.004614
- Bartolomucci, A., Palanza, P., Costoli, T., Savani, E., Laviola, G., Parmigiani, S., & Sgoifo, A. (2003). Chronic psychosocial stress persistently alters autonomic function and physical activity in mice. Physiology & Behavior, 80(1), 57–67. https://doi.org/10.1016/S0031-9384(03)00209-9
- Bartolomucci, A., Palanza, P., Gaspani, L., Limiroli, E., Panerai, A. E., Ceresini, G., Poli, M. D., & Parmigiani, S. (2001). Social status in mice: Behavioral, endocrine and immune changes are context dependent. Physiology & Behavior, 73(3), 401–410. https://doi.org/10.1016/S0031-9384(01)00453-X
- Bartolomucci, A., Pederzani, T., Sacerdote, P., Panerai, A. E., Parmigiani, S., & Palanza, P. (2004). Behavioral and physiological characterization of male mice under chronic psychosocial stress. Psychoneuroendocrinology, 29(7), 899–910. https://doi.org/10.1016/j.psyneuen.2003.08.003
- Blaauwbroek, R., Stant, A. D., Groenier, K. H., Kamps, W. A., Meyboom, B., & Postma, A. (2007). Health-related quality of life and adverse late effects in adult (very) long-term childhood cancer survivors. European Journal of Cancer, 43(1), 122–130. https://doi.org/10.1016/j.ejca.2006.08.003
- Blaes, A., Konety, S., & Zordoky, B. (2021). Response to schoormans. Journal of the National Cancer Institute, 113(2), 214–215. https://doi.org/10.1093/jnci/djaa158
- Bosman, M., Kruger, D. N., Favere, K., Wesley, C. D., Neutel, C. H. G., Van Asbroeck, B., Diebels, O. R., Faes, B., Schenk, T. J., Martinet, W., De Meyer, G. R. Y., Van Craenenbroeck, E. M., & Guns, P. D. F. (2021). Doxorubicin impairs smooth muscle cell contraction: Novel insights in vascular toxicity. International Journal of Molecular Sciences, 22, 12812. https://doi.org/10.3390/ijms222312812
- Brinkman, T. M., Li, C., Vannatta, K., Marchak, J. G., Lai, J. S., Prasad, P. K., Kimberg, C., Vuotto, S., Di, C., Srivastava, D., Robison, L. L., Armstrong, G. T., & Krull, K. R. (2016). Behavioral, social, and emotional symptom comorbidities and profiles in adolescent survivors of childhood cancer: A report from the childhood cancer survivor study. Journal of Clinical Oncology, 34(28), 3417–3425. https://doi.org/10.1200/JCO.2016.66.4789
- Carnevali, L., Mastorci, F., Audero, E., Graiani, G., Rossi, S., Macchi, E., Callegari, S., Bartolomucci, A., Nalivaiko, E., Quaini, F., Gross, C., & Sgoifo, A. (2012). Stress-induced susceptibility to sudden cardiac death in mice with altered serotonin homeostasis. PLoS One, 7(7), e41184. https://doi.org/10.1371/journal.pone.0041184
- Çetin, S., Babaoğlu, K., Başar, E. Z., Deveci, M., & Çorapçıoğlu, F. (2018). Subclinical anthracycline-induced cardiotoxicity in long-term follow-up of asymptomatic childhood cancer survivors: Assessment by speckle tracking echocardiography. Echocardiography, 35(2), 234–240. https://doi.org/10.1111/echo.13743
- Chen, D. S., Yan, J., & Yang, P. Z. (2022). Cardiomyocyte atrophy, an underestimated contributor in doxorubicin-induced cardiotoxicity. Frontiers in Cardiovascular Medicine, 9, 812578. https://doi.org/10.3389/fcvm.2022.812578
- Cheung, Y. F., Lam, W. W., Ip, J. J., Cheuk, D. K., Cheng, F. W., Yang, J. Y., Yau, J. P., Ho, K. K., Li, C. K., Li, R. C., Yuen, H. L., Ling, A. S., Li, V. W., & Chan, G. C. (2015). Myocardial iron load and fibrosis in long term survivors of childhood leukemia. Pediatric Blood & Cancer, 62(4), 698–703. https://doi.org/10.1002/pbc.25369
- Chou, C. H., Hung, C. S., Liao, C. W., Wei, L. H., Chen, C. W., Shun, C. T., Wen, W. F., Wan, C. H., Wu, X. M., Chang, Y. Y., Wu, V. C., Wu, K. D., & Lin, Y. H. (2018). IL-6 trans-signalling contributes to aldosterone-induced cardiac fibrosis. Cardiovascular Research, 114(5), 690–702. https://doi.org/10.1093/cvr/cvy013
- Costoli, T., Bartolomucci, A., Graiani, G., Stilli, D., Laviola, G., & Sgoifo, A. (2004). Effects of chronic psychosocial stress on cardiac autonomic responsiveness and myocardial structure in mice. American Journal of Physiology Heart and Circulatory Physiology, 286(6), H2133–2140. https://doi.org/10.1152/ajpheart.00869.2003
- Dadomo, H., Sanghez, V., Di Cristo, L., Lori, A., Ceresini, G., Malinge, I., Parmigiani, S., Palanza, P., Sheardown, M., & Bartolomucci, A. (2011). Vulnerability to chronic subordination stress-induced depression-like disorders in adult 129SvEv male mice. Progress in Neuro-Psychopharmacology & Biological Psychiatry, 35(6), 1461–1471. https://doi.org/10.1016/j.pnpbp.2010.11.016
- de Wildt, D. J., de Jong, Y., Hillen, F. C., Steerenberg, P. A., & van Hoesel, Q. G. (1985). Cardiovascular effects of doxorubicin-induced toxicity in the intact Lou/M Wsl rat and in isolated heart preparations. The Journal of Pharmacology and Experimental Therapeutics, 235(1), 234–240.
- Dutta, S., & Sengupta, P. (2016). Men and mice: Relating their ages. Life Sciences, 152, 244–248. https://doi.org/10.1016/j.lfs.2015.10.025
- Ehrhardt, M. J., Mulrooney, D. A., Li, C., Baassiri, M. J., Bjornard, K., Sandlund, J. T., Brinkman, T. M., Huang, I. C., Srivastava, D. K., Ness, K. K., Robison, L. L., Hudson, M. M., & Krull, K. R. (2018). Neurocognitive, psychosocial, and quality-of-life outcomes in adult survivors of childhood non-Hodgkin lymphoma. Cancer, 124(2), 417–425. https://doi.org/10.1002/cncr.31019
- Faber, J., Wingerter, A., Neu, M. A., Henninger, N., Eckerle, S., Munzel, T., Lackner, K. J., Beutel, M. E., Blettner, M., Rathmann, W., Peters, A., Meisinger, C., Linkohr, B., Neuhauser, H., Kaatsch, P., Spix, C., Schneider, A., Merzenich, H., Panova-Noeva, M., Prochaska, J. H., & Wild, P. S. (2018). Burden of cardiovascular risk factors and cardiovascular disease in childhood cancer survivors: Data from the German CVSS-study. European Heart Journal, 39(17), 1555–1562. https://doi.org/10.1093/eurheartj/ehy026
- Ford, D. E., Mead, L. A., Chang, P. P., Cooper-Patrick, L., Wang, N. Y., & Klag, M. J. (1998). Depression is a risk factor for coronary artery disease in men: The precursors study. Archives of Internal Medicine, 158(13), 1422–1426. https://doi.org/10.1001/archinte.158.13.1422
- Germanakis, I., Kalmanti, M., Parthenakis, F., Nikitovic, D., Stiakaki, E., Patrianakos, A., & Vardas, P. E. (2006). Correlation of plasma N-terminal pro-brain natriuretic peptide levels with left ventricle mass in children treated with anthracyclines. International Journal of Cardiology, 108(2), 212–215. https://doi.org/10.1016/j.ijcard.2005.05.006
- Johannsdottir, I. M. R., Hamre, H., Fossa, S. D., Loge, J. H., Drolsum, L., Lund, M. B., Nordsletten, L., & Kiserud, C. (2017). Adverse health outcomes and associations with self-reported general health in childhood lymphoma survivors. Journal of Adolescent and Young Adult Oncology, 6(3), 470–476. https://doi.org/10.1089/jayao.2017.0018
- Kamphuis, M. H., Kalmijn, S., Tijhuis, M. A., Geerlings, M. I., Giampaoli, S., Nissinen, A., Grobbee, D. E., & Kromhout, D. (2006). Depressive symptoms as risk factor of cardiovascular mortality in older European men: The Finland, Italy and Netherlands Elderly (FINE) study. European Journal of Cardiovascular Prevention and Rehabilitation, 13(2), 199–206. https://doi.org/10.1097/01.hjr.0000188242.64590.92
- Kelly, K. M. (2012). Management of children with high-risk Hodgkin lymphoma. British Journal of Haematology, 157(1), 3–13. https://doi.org/10.1111/j.1365-2141.2011.08975.x
- Kivimaki, M., Virtanen, M., Elovainio, M., Kouvonen, A., Vaananen, A., & Vahtera, J. (2006). Work stress in the etiology of coronary heart disease–a meta-analysis. Scandinavian Journal of Work, Environment & Health, 32(6), 431–442. https://doi.org/10.5271/sjweh.1049
- Kuba, K., Esser, P., Mehnert, A., Hinz, A., Johansen, C., Lordick, F., & Gotze, H. (2019). Risk for depression and anxiety in long-term survivors of hematologic cancer. Health Psychology, 38(3), 187–195. https://doi.org/10.1037/hea0000713
- Leger, K., Slone, T., Lemler, M., Leonard, D., Cochran, C., Bowman, W. P., Bashore, L., & Winick, N. (2015). Subclinical cardiotoxicity in childhood cancer survivors exposed to very low dose anthracycline therapy. Pediatric Blood & Cancer, 62(1), 123–127. https://doi.org/10.1002/pbc.25206
- Liao, C. W., Chou, C. H., Wu, X. M., Chen, Z. W., Chen, Y. H., Chang, Y. Y., Wu, V. C., Rose-John, S., Hung, C. S., & Lin, Y. H. (2020). Interleukin-6 plays a critical role in aldosterone-induced macrophage recruitment and infiltration in the myocardium. Biochimica et Biophysica Acta Molecular Basis of Disease, 1866(3), 165627. https://doi.org/10.1016/j.bbadis.2019.165627
- Lipshultz, S. E., Diamond, M. B., Franco, V. I., Aggarwal, S., Leger, K., Santos, M. V., Sallan, S. E., & Chow, E. J. (2014). Managing chemotherapy-related cardiotoxicity in survivors of childhood cancers. Paediatric Drugs, 16(5), 373–389. https://doi.org/10.1007/s40272-014-0085-1
- Lipshultz, S. E., Lipsitz, S. R., Kutok, J. L., Miller, T. L., Colan, S. D., Neuberg, D. S., Stevenson, K. E., Fleming, M. D., Sallan, S. E., Franco, V. I., Henkel, J. M., Asselin, B. L., Athale, U. H., Clavell, L. A., Michon, B., Laverdiere, C., Larsen, E., Kelly, K. M., & Silverman, L. B. (2013). Impact of hemochromatosis gene mutations on cardiac status in doxorubicin-treated survivors of childhood high-risk leukemia. Cancer, 119(19), 3555–3562. https://doi.org/10.1002/cncr.28256
- Lipshultz, S. E., Lipsitz, S. R., Sallan, S. E., Simbre, V. C., 2nd, Shaikh, S. L., Mone, S. M., Gelber, R. D., & Colan, S. D. (2002). Long-term enalapril therapy for left ventricular dysfunction in doxorubicin-treated survivors of childhood cancer. Journal of Clinical Oncology, 20(23), 4517–4522. https://doi.org/10.1200/JCO.2002.12.102
- Lipshultz, S. E., Miller, T. L., Lipsitz, S. R., Neuberg, D. S., Dahlberg, S. E., Colan, S. D., Silverman, L. B., Henkel, J. M., Franco, V. I., Cushman, L. L., Asselin, B. L., Clavell, L. A., Athale, U., Michon, B., Laverdiere, C., Schorin, M. A., Larsen, E., Usmani, N., & Sallan, S. E. (2012). Continuous versus bolus infusion of doxorubicin in children with all: Long-term cardiac outcomes. Pediatrics, 130(6), 1003–1011. https://doi.org/10.1542/peds.2012-0727
- Lipshultz, S. E., Sambatakos, P., Maguire, M., Karnik, R., Ross, S. W., Franco, V. I., & Miller, T. L. (2014). Cardiotoxicity and cardioprotection in childhood cancer. Acta Haematologica, 132(3–4), 391–399. https://doi.org/10.1159/000360238
- Maayah, Z. H., Alam, A. S., Takahara, S., Soni, S., Ferdaoussi, M., Matsumura, N., Zordoky, B. N., Eisenstat, D. D., & Dyck, J. R. B. (2021). Resveratrol reduces cardiac NLRP3-inflammasome activation and systemic inflammation to lessen doxorubicin-induced cardiotoxicity in juvenile mice. FEBS Letters, 595(12), 1681–1695. https://doi.org/10.1002/1873-3468.14091
- Matsumura, N., Zordoky, B. N., Robertson, I. M., Hamza, S. M., Parajuli, N., Soltys, C.-L M., Beker, D. L., Grant, M. K., Razzoli, M., Bartolomucci, A., & Dyck, J. R. B. (2018). Co-administration of resveratrol with doxorubicin in young mice attenuates detrimental late-occurring cardiovascular changes. Cardiovascular Research, 114, 1350–1359. https://doi.org/10.1093/cvr/cvy064
- Mawad, W., Mertens, L., Pagano, J. J., Riesenkampff, E., Reichert, M. J. E., Mital, S., Kantor, P. F., Greenberg, M., Liu, P., Nathan, P. C., & Grosse-Wortmann, L. (2020). Effect of anthracycline therapy on myocardial function and markers of fibrotic remodelling in childhood cancer survivors. Eur Heart J Cardiovasc Imaging, 22, 435–442. https://doi.org/10.1093/ehjci/jeaa093
- Ness, K. K., Armstrong, G. T., Kundu, M., Wilson, C. L., Tchkonia, T., & Kirkland, J. L. (2015). Frailty in childhood cancer survivors. Cancer, 121(10), 1540–1547. https://doi.org/10.1002/cncr.29211
- Nysom, K., Holm, K., Lipsitz, S. R., Mone, S. M., Colan, S. D., Orav, E. J., Sallan, S. E., Olsen, J. H., Hertz, H., Jacobsen, J. R., & Lipshultz, S. E. (1998). Relationship between cumulative anthracycline dose and late cardiotoxicity in childhood acute lymphoblastic leukemia. Journal of Clinical Oncology, 16(2), 545–550. https://doi.org/10.1200/JCO.1998.16.2.545
- Oeffinger, K. C., Mertens, A. C., Sklar, C. A., Kawashima, T., Hudson, M. M., Meadows, A. T., Friedman, D. L., Marina, N., Hobbie, W., Kadan-Lottick, N. S., Schwartz, C. L., Leisenring, W., Robison, L. L., & Childhood Cancer Survivor Study. (2006). Chronic health conditions in adult survivors of childhood cancer. The New England Journal of Medicine, 355(15), 1572–1582. https://doi.org/10.1056/NEJMsa060185
- Patterson, Z. R., Khazall, R., Mackay, H., Anisman, H., & Abizaid, A. (2013). Central ghrelin signaling mediates the metabolic response of C57BL/6 male mice to chronic social defeat stress. Endocrinology, 154(3), 1080–1091. https://doi.org/10.1210/en.2012-1834
- Prasad, P. K., Hardy, K. K., Zhang, N., Edelstein, K., Srivastava, D., Zeltzer, L., Stovall, M., Seibel, N. L., Leisenring, W., Armstrong, G. T., Robison, L. L., & Krull, K. (2015). Psychosocial and neurocognitive outcomes in adult survivors of adolescent and early young adult cancer: A report from the childhood cancer survivor study. Journal of Clinical Oncology, 33(23), 2545–2552. https://doi.org/10.1200/JCO.2014.57.7528
- Rabelo, E., De Angelis, K., Bock, P., Gatelli Fernandes, T., Cervo, F., Bello Klein, A., Clausell, N., & Claudia Irigoyen, M. (2001). Baroreflex sensitivity and oxidative stress in adriamycin-induced heart failure. Hypertension (Dallas, TX: 1979), 38(3 Pt 2), 576–580. https://doi.org/10.1161/hy09t1.096185
- Razzoli, M., Frontini, A., Gurney, A., Mondini, E., Cubuk, C., Katz, L. S., Cero, C., Bolan, P. J., Dopazo, J., Vidal-Puig, A., Cinti, S., & Bartolomucci, A. (2016). Stress-induced activation of brown adipose tissue prevents obesity in conditions of low adaptive thermogenesis. Molecular Metabolism, 5(1), 19–33. https://doi.org/10.1016/j.molmet.2015.10.005
- Razzoli, M., Karsten, C., Yoder, J. M., Bartolomucci, A., & Engeland, W. C. (2014). Chronic subordination stress phase advances adrenal and anterior pituitary clock gene rhythms. American Journal of Physiology, Regulatory, Integrative and Comparative Physiology, 307(2), R198–205. https://doi.org/10.1152/ajpregu.00101.2014
- Razzoli, M., Lindsay, A., Law, M. L., Chamberlain, C. M., Southern, W. M., Berg, M., Osborn, J., Engeland, W. C., Metzger, J. M., Ervasti, J. M., & Bartolomucci, A. (2020). Social stress is lethal in the mdx model of Duchenne muscular dystrophy. EBioMedicine, 55, 102700. https://doi.org/10.1016/j.ebiom.2020.102700
- Razzoli, M., Sanghez, V., & Bartolomucci, A. (2015). Chronic subordination stress induces hyperphagia and disrupts eating behavior in mice modeling binge-eating-like disorder. Frontiers in Nutrition, 1, 30. https://doi.org/10.3389/fnut.2014.00030
- Reagan-Shaw, S., Nihal, M., & Ahmad, N. (2008). Dose translation from animal to human studies revisited. FASEB Journal, 22(3), 659–661. https://doi.org/10.1096/fj.07-9574LSF
- Rozanski, A., Blumenthal, J. A., & Kaplan, J. (1999). Impact of psychological factors on the pathogenesis of cardiovascular disease and implications for therapy. Circulation, 99(16), 2192–2217. https://doi.org/10.1161/01.cir.99.16.2192
- Sanghez, V., Cubuk, C., Sebastian-Leon, P., Carobbio, S., Dopazo, J., Vidal-Puig, A., & Bartolomucci, A. (2016). Chronic subordination stress selectively downregulates the insulin signaling pathway in liver and skeletal muscle but not in adipose tissue of male mice. Stress (Amsterdam, Netherlands), 19(2), 214–224. https://doi.org/10.3109/10253890.2016.1151491
- Sanghez, V., Razzoli, M., Carobbio, S., Campbell, M., McCallum, J., Cero, C., Ceresini, G., Cabassi, A., Govoni, P., Franceschini, P., de Santis, V., Gurney, A., Ninkovic, I., Parmigiani, S., Palanza, P., Vidal-Puig, A., & Bartolomucci, A. (2013). Psychosocial stress induces hyperphagia and exacerbates diet-induced insulin resistance and the manifestations of the metabolic syndrome. Psychoneuroendocrinology, 38(12), 2933–2942. https://doi.org/10.1016/j.psyneuen.2013.07.022
- Schoormans, D. (2021). RE: Cardiovascular disease in breast cancer survivors: An important topic in breast cancer survivorship. Journal of the National Cancer Institute, 113(2), 212–213. https://doi.org/10.1093/jnci/djaa157
- Schoormans, D., Pedersen, S. S., Dalton, S., Rottmann, N., & Poll-Franse, L. V. D. (2016). Cardiovascular co-morbidity in cancer patients: The role of psychological distress. Cardio-Oncology (London, England), 2(1), 9. https://doi.org/10.1186/s40959-016-0019-x
- Schoormans, D., van de Poll-Franse, L., Vissers, P., van Herk-Sukel, M. P. P., Pedersen, S. S., Rottmann, N., Horsbol, T., Dalton, S., & Denollet, J. (2017). Pharmaceutically treated anxiety but not depression prior to cancer diagnosis predicts the onset of cardiovascular disease among breast cancer survivors. Breast Cancer Research and Treatment, 166(1), 259–266. https://doi.org/10.1007/s10549-017-4387-1
- Shen, H., Wang, J., Min, J., Xi, W., Gao, Y., Yin, L., Yu, Y., Liu, K., Xiao, J., Zhang, Y. F., & Wang, Z. N. (2018). Activation of TGF-beta1/alpha-SMA/Col I profibrotic pathway in fibroblasts by galectin-3 contributes to atrial fibrosis in experimental models and patients. Cellular Physiology and Biochemistry: International Journal of Experimental Cellular Physiology, Biochemistry, and Pharmacology, 47(2), 851–863. https://doi.org/10.1159/000490077
- Simas, B. B., Nunes, E. A., Crestani, C. C., & Speretta, G. F. (2018). Cardiovascular and metabolic consequences of the association between chronic stress and high-fat diet in rats. Stress (Amsterdam, Netherlands), 21(3), 247–256. https://doi.org/10.1080/10253890.2018.1437413
- Teng, A. E., Noor, B., Ajijola, O. A., & Yang, E. H. (2021). Chemotherapy and radiation-associated cardiac autonomic dysfunction. Current Oncology Reports, 23(2), 14. https://doi.org/10.1007/s11912-020-01013-7
- Tham, E. B., Haykowsky, M. J., Chow, K., Spavor, M., Kaneko, S., Khoo, N. S., Pagano, J. J., Mackie, A. S., & Thompson, R. B. (2013). Diffuse myocardial fibrosis by T1-mapping in children with subclinical anthracycline cardiotoxicity: Relationship to exercise capacity, cumulative dose and remodeling. Journal of Cardiovascular Magnetic Resonance, 15, 48. https://doi.org/10.1186/1532-429X-15-48
- Tong, X., Li, V. W., Liu, A. P., So, E. K., Chan, Q., Ho, K. K., Yau, J. P., Cheuk, D. K., Cheung, Y. F., & Ng, M. Y. (2019). Cardiac magnetic resonance T1 mapping in adolescent and young adult survivors of childhood cancers. Circulation: Cardiovascular Imaging, 12, e008453. https://doi.org/10.1161/CIRCIMAGING.118.008453
- Tremolada, M., Bonichini, S., Basso, G., & Pillon, M. (2016). Post-traumatic stress symptoms and post-traumatic growth in 223 childhood cancer survivors: Predictive risk factors. Frontiers in Psychology, 7, 287. https://doi.org/10.3389/fpsyg.2016.00287
- van Dalen, E. C., Raphael, M. F., Caron, H. N., & Kremer, L. C. (2011). Treatment including anthracyclines versus treatment not including anthracyclines for childhood cancer. Cochrane Database Systematic Review, 19;(1):CD006647.
- Vandecruys, E., Mondelaers, V., De Wolf, D., Benoit, Y., & Suys, B. (2012). Late cardiotoxicity after low dose of anthracycline therapy for acute lymphoblastic leukemia in childhood. Journal of Cancer Survivorship, 6(1), 95–101. https://doi.org/10.1007/s11764-011-0186-6
- Vuotto, S. C., Krull, K. R., Li, C., Oeffinger, K. C., Green, D. M., Patel, S. K., Srivastava, D., Stovall, M., Ness, K. K., Armstrong, G. T., Robison, L. L., & Brinkman, T. M. (2017). Impact of chronic disease on emotional distress in adult survivors of childhood cancer: A report from the Childhood Cancer Survivor Study. Cancer, 123(3), 521–528. https://doi.org/10.1002/cncr.30348
- Wilcox, R. R. (1987). New statistical procedures for the social sciences: Modern solutions to basic problems. L. Erlbaum Associates.
- Yazaki, K., Takahashi, K., Shigemitsu, S., Yamada, M., Iso, T., Kobayashi, M., Akimoto, K., Tamaichi, H., Fujimura, J., Saito, M., Nii, M., & Shimizu, T. (2018). In-depth insight into the mechanisms of cardiac dysfunction in patients with childhood cancer after anthracycline treatment using layer-specific strain analysis. Circulation Journal, 82(3), 715–723. https://doi.org/10.1253/circj.CJ-17-0874
- Yeh, E. T., & Vejpongsa, P. (2015). Subclinical cardiotoxicity associated with cancer therapy: Early detection and future directions. Journal of the American College of Cardiology, 65(23), 2523–2525. https://doi.org/10.1016/j.jacc.2015.04.012
- Ylanen, K., Poutanen, T., Savikurki-Heikkila, P., Rinta-Kiikka, I., Eerola, A., & Vettenranta, K. (2013). Cardiac magnetic resonance imaging in the evaluation of the late effects of anthracyclines among long-term survivors of childhood cancer. Journal of the American College of Cardiology, 61(14), 1539–1547. https://doi.org/10.1016/j.jacc.2013.01.019