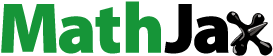
Abstract
The Coronavirus Disease 2019 (COVID-19) pandemic has caused massive disruptions to daily life in the United States, closing schools and businesses and increasing physical and social isolation, leading to deteriorations in mental health and well-being in people of all ages. Many studies have linked chronic stress with long-term changes in cortisol secretion, which has been implicated in many stress-related physical and mental health problems that commonly emerge in adolescence. However, the physiological consequences of the pandemic in youth remain understudied. Using hair cortisol concentrations (HCC), we quantified average longitudinal changes in cortisol secretion across a four-month period capturing before, during, and after the transition to pandemic-lockdown conditions in a sample of healthy youth (n = 49). Longitudinal changes in HCC were analyzed using linear mixed-effects models. Perceived levels of pandemic-related stress were measured and compared to the physiological changes in HCC. In children and adolescents, cortisol levels significantly increased across the course of the pandemic. These youth reported a multitude of stressors during this time, although changes in HCC were not associated with self-reported levels of COVID-19-related distress. We provide evidence that youth are experiencing significant physiological changes in cortisol activity across the COVID-19 pandemic, yet these biological responses are not associated with perceived stress levels. Youth may be especially vulnerable to the deleterious impacts of chronic cortisol exposure due to their current status in the sensitive periods for development, and the incongruency between biological and psychological stress responses may further complicate these developmental problems.
1. Introduction
Protective measures were enacted to mitigate the spread of the Coronavirus Disease 2019 (COVID-19) pandemic, causing massive disruptions to daily life and affecting communities nationwide. Widespread closures of businesses and schools impacted over 55-million students in the United States (Education Week, Citation2020), causing increasing levels of psychological distress and negatively impacting parental and youth well-being and behavioral health (Achterberg et al., Citation2021; McGinty et al., Citation2020; Patrick et al., Citation2020). Though most COVID-19 infections in children are less severe than in adults (Ludvigsson, Citation2020), the unpredictable experiences of physical and social isolation still leave youth highly susceptible to psychological consequences, as many children have reported declines in mental health (Bignardi et al., Citation2020; Liang et al., Citation2020).
Characterizing COVID-19-related stress is important, as chronic stress exposure early in life can permanently alter development (Dantzer et al., Citation2020). Physical and psychological stressors activate the hypothalamic–pituitary–adrenal (HPA) axis, which stimulates a variety of neurological and physiological responses, including the release of the glucocorticoid hormone, cortisol (Dedovic et al., Citation2009; Smith and Vale, Citation2006). Although activating the HPA-axis is necessary to adequately respond to stressors, exposure to sustained, elevated levels of cortisol may have deleterious effects, especially during important developmental windows. Maturation is highly susceptible to environmental influences in adolescence (Orben et al., Citation2020; Vijayakumar et al., Citation2018), and puberty is one of the most substantial periods of neuroendocrine development. Specifically, stress reactivity becomes more sensitive in adolescence relative to childhood (Gunnar et al., Citation2009; Spear, Citation2000). Increases in cortisol secretion have the potential to alter pubertal development of other neurobiological/physiological systems (Fox et al., Citation2010; Lupien et al., Citation2009; Spear, Citation2000); therefore, prolonged, exacerbated HPA-activity during the adolescent sensitive period in response to COVID-19 could disrupt normative developmental processes and stress dynamics that reverberate throughout the lifespan.
Stress biomarkers can provide biological signatures of adverse experiences and the neuroendocrinological impacts stress. Though salivary analyses are more common, this technique only measures acute levels of hormones and thus can be heavily influenced by momentary fluctuations (e.g. diurnal rhythms, situational stressors; (Knutsson et al., Citation1997; Matchock et al., Citation2007)). In contrast, hair-cortisol-concentrations (HCCs) are a stable measure of average cortisol production, which can help alleviate the temporal variability that can confound acute measures (Stalder et al., Citation2017). HCC provides reliable estimates of long-term free cortisol production, as 1 cm hair segments have been validated to reflect ∼30-day integrated measures of salivary cortisol (Short et al., Citation2016). Segmenting longer hair samples into 1 cm sections allows for the retrospective study of hormone levels, and higher HCC indexed chronic stress following major life stressors, ranging from unemployment to natural disasters (Dajani et al., Citation2018; Luo et al., Citation2012; Stalder et al., Citation2017). Thus, HCC is an exemplary method to investigate longitudinal changes in unpredicted stress and cortisol activity across the early months of the pandemic, as families transitioned from normal life to lockdowns.
The current study aims to evaluate changes in stress during the COVID-19 pandemic in a group of typically developing, uninfected youth. Participants provided a hair sample that was analyzed to measure average monthly cortisol values across a period of four months. These measures assess cortisol both before and after preventative distancing measures were locally enacted to comprehensively capture the transition to the isolating lockdown conditions during the start of the pandemic. We hypothesized youth would exhibit increasing cortisol levels as the pandemic progressed due to the abrupt and dramatic changes to daily-life and the reported declines in behavioral and mental health.
2. Materials and methods
2.1. Participants
Youth who were enrolled in ongoing longitudinal studies of neurocognitive development (Stephen et al., Citation2021) were invited to participate remotely in the present study focusing on the physiological and psychological implications of the COVID-19 pandemic in youth. Forty-nine typically developing children and adolescents ages 10–18 years provided hair samples for hormone analyses. All participants were recruited from the University of Nebraska Medical Center (UNMC) site. All procedures were approved by the UNMC Institutional Review Board, and informed consent from the child’s parent or legal guardian, as well as assent from the child, were obtained electronically before proceeding with the study.
We computed a power analysis in G*Power 3.1 (Faul et al., Citation2007) to determine how many participants would be required to detect an effect size of f = 0.38, alpha = .05, and power = 0.80, consistent with prior studies investigating chronic stressors and hair cortisol concentrations (Staufenbiel et al., Citation2013). Thus, a projected sample size of at least N = 45 would provide ample power for detecting this medium-large effect size.
2.2. Procedure
2.2.1. Hair sample collection and analysis
Parents were provided with video instructions on home sample collection and cut 4 cm hair segments from the posterior vertex of the head to be shipped back to the laboratory for analysis. (See supplement for further details on hair collection methods).
The hair sample was separated into 1 cm sections, so four 1 cm segments of hair were used per participant (hair most proximal to the cut/scalp was used if the sample provided was longer than 4 cm). Corresponding date ranges for each of the four 1 cm segments were calculated retrospectively from the original collection date using this representative rate of hair growth (i.e. 1 cm = 1 month), thus constructing a four-month period of hormone measurements. Samples were collected from May 10 through June 18, with each whole sample representing approximately 4 months of time retrospectively. This provided a range of hormone measures dating from January 11 through June 18, 2020 across all participants (). Of note, the majority of local school closures and lockdowns began around March 15 (1). Following an established protocol, each hair segment was analyzed for cortisol concentration (See supplement for further details on hair hormone analysis methods). The inter- and intra-assay coefficients of variation were 5.70% and 8.54%, respectively.
Figure 1. Change in cortisol levels across time during the COVID-19 pandemic in 2020. Real-time data points show the date corresponding to the midpoint of each hair segment on the x-axis, and raw hair cortisol concentrations (HCC) for each segment on the y-axis. Individual trajectories are mapped per participant across a four-month period. A general trend of increasing cortisol levels with later dates/increasing exposure to COVID-19 repercussions is shown qualitatively. Dashed line indicates March 15, 2020, the average date of local school district closures (i.e. functional date of enacting preventative measures and physical distancing).
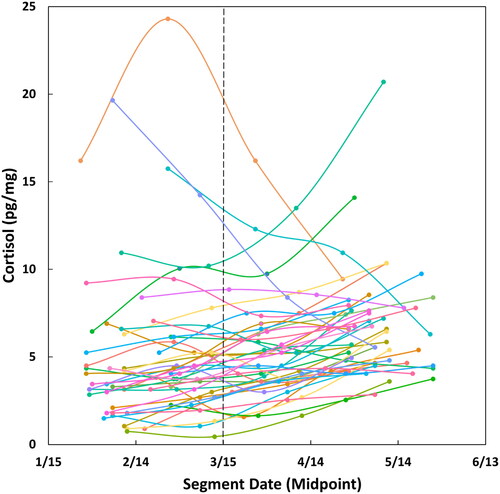
Outlier analyses were performed in two waves. First, participants with all four hair cortisol values exceeding 2.5 SD of the overall mean were excluded from subsequent analyses. Next, individual extreme cortisol values (i.e. a single outlier hair segment, with other hair segments provided by the participant being within range) were winsorized to 2.5 SD of the sample mean per time point.
2.2.2. Statistical analyses
To examine the change in HCCs over the course of the pandemic, statistical analyses were conducted with multilevel modeling (MLM) using R and package nlme (Pinheiro et al., Citation2021). These mixed-effects designs can account for both within- and between-individual variances in longitudinal and repeated-measures samples (i.e. can model the hormone trajectory within a single participant [within-individual], as well as the differences between participant trajectories [between-individuals] (Nezlek, Citation2012)). Due to the vast individual variability in basal cortisol levels and cortisol responsivity, MLM allows the longitudinal analysis of the cumulative trajectory of cortisol over time within the full sample, while accounting for these multiple sources of variance.
HCC was modeled as a function of time (i.e. hair segment), with cortisol measurements nested within participant by estimating a random intercept and random slope for each participant (see model syntax below). Time point 1 reflects cortisol measures from the earliest hair segment sample (i.e. most distal segment), while time point 4 reflects hormone levels from the most recent segment (i.e. most proximal segment).
Of note, this model was tested with and without random effects, but only the best fitting model was reported.
Follow-up analyses probed whether demographic variables interacted with the overall increase in cortisol over time. Time-by-sex, time-by-age, and time-by-collection date interactions were included as predictors of cortisol measures, with similar data groupings for each participant (i.e. random intercept and random slope). Continuous variables were grand mean centered.
2.2.3. Questionnaires
Youth completed a battery of questionnaires probing repercussions of the COVID-19 pandemic, including a portion of the “Emotional Experience” section of the COVID-19 Adolescent Symptom & Psychological Experience (CASPE) where they were asked to rate the level of concern they felt about pandemic- and lockdown-relevant stressors in the past seven days (Ladouceur, Citation2020) (See supplement for further details on questionnaire administration). Ratings were completed on a 4-point Likert scale (0 = not at all, 4 = a great deal). Any level of endorsement (score >0) of a given stressor was summed into a total stress score per participant.
Follow-up exploratory analyses were used to examine the relationship between youths’ perception of these stressors and their biological cortisol responses. The slope of each individual’s change in cortisol was extracted from the MLM model. The association between HCC changes and COVID-19-related distress, measured by total stress scores reported from the CASPE, was additionally probed using spearman’s correlation tests.
3. Results
3.1. Hormone results
Of the 49 participants who provided hair samples, one was excluded due to abnormally high cortisol levels (i.e. all values exceeding 2.5 SDs above the sample mean). Thus, the final sample consisted of 48 youth (Mage=14.79 years, SD = 1.95; 34 females) with complete data sets, constituting 192 hormone measures used in the final analysis (Mcortisol=5.60 pg/mg, SD = 3.07). Initial cortisol activity measures (T1, pre-pandemic) did not differ by sex (t = 1.63, p = .11) or age (r = .12, p = .90). No hair-related parameters (e.g. hair type, color, treatment, etc.) were significantly associated with HCCs (all ps > .50).
3.2. MLM results
Multilevel models revealed a significant increase in cortisol values with increasing time spent during the pandemic (F(3,141) = 5.905, p<.001; ). Although initial cortisol levels varied by participant (F(1,141) = 335.23, p <.001), this effect of time on cortisol was significant above-and-beyond these inter-individual differences in initial cortisol levels. Thus, the MLM structure showed the fixed effect of cortisol increased over time, while controlling for both within- and between-subjects random effects.
Figure 2. Changes in cortisol levels across time. Random slopes from the linear mixed-effects model used in statistical analyses were extracted and mapped individually per participant, which showed an overall significant increase in cortisol levels with increasing time across the whole sample (p =.001). Data points are grouped by time point / hair segment using winsorized data. Time point 1 reflects cortisol measures from the earliest hair segment sample (i.e. most distal segment), while time point 4 reflects hormone levels from the most recent segment (i.e. most proximal segment to the scalp). Significant local and national events related to COVID-19 are depicted below and loosely correspond to the overall range of time (i.e. January 11–June 18) of collected cortisol levels.
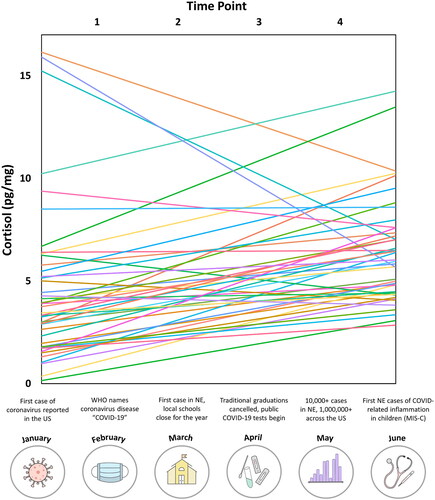
Follow-up analyses showed this increase in cortisol over time did not differ by sex (F(3,138) = 0.56, p = .54), age (F(3,137) = 1.67, p = .18), or the day the hair sample was collected (F(3,137) = 1.29, p = .28). Thus, the trajectories of increasing cortisol did not interact with other potential factors within the sample.
3.3. Questionnaire results
The COVID-19 Adolescent Symptom & Psychological Experience revealed that 97.8% of the sample experienced at least one stressor related to the pandemic and its repercussions. The stressors most common and rated most severe were “not seeing friends in person” (M = 1.94, SD = 1.40, 74%), “people might die if they get sick” (M = 1.54, SD = 1.28, 75%), and “family might get sick” (M = 1.56, SD = 1.30, 74%). Descriptive statistics of all 16 pandemic-related stressors are shown in .
Table 1. Descriptive statistics of ratings of COVID-19 related stressors.
Follow-up analyses revealed that COVID-19-related distress ratings were not associated with physiological cortisol changes across the pandemic (ρ=-.048, p = .75), such that youth showing larger increases in cortisol across the pandemic did not necessarily perceive the impacts of COVID-19 to be more stressful than their peers exhibiting less physiological changes in the cortisol response.
4. Discussion
In this study, we examined the trajectory of cortisol responses across the COVID-19 pandemic in a sample of typically developing adolescents. We observed significant individual differences in baseline cortisol activity measures, in addition to overall increases in hair cortisol levels across a four-month period, indicating chronically elevated levels of stress hormones during the pandemic. Ratings of concern about COVID-19 repercussions revealed a multitude of stressors that likely contributed to the augmented cortisol response observed, though no direct associations between perceived stress and the resulting physiological responses were found. Nonetheless, these findings suggest dysregulation of circulating cortisol levels during the pandemic, which may have long-term implications for subsequent physiological and psychological development.
HCCs reflect long-term exposure to chronic stress (Stalder et al., Citation2017; Short et al., Citation2016), therefore our findings of significantly increasing cortisol levels across the course of the pandemic reveals youth did experience prolonged, physiological stress during this extended event. Though cortisol secretion in response to acute threats has beneficial adaptive purposes, prolonged exposure to glucocorticoids, as seen in the present study, can facilitate an array of physical and psychological problems (McEwen, Citation1998; Sapolsky, Citation2000). Of particular concern, maturational processes are highly vulnerable to environmental influence during designated sensitive periods, and chronic cortisol exposure in youth can impair the proper development of stress-reactive systems later in life. Early life adversity is well documented to cause lasting changes to neurobiological systems involved in stress regulation and reactivity later in life and alter cognitive and emotional processes that develop in early childhood (Hunter et al., Citation2011; Lupien et al., Citation2009).
The adolescent transition is a period of vast physical, neurological, and behavioral development that is susceptible to environmental influences, including the neuroendocrine responses of the HPA axis (Spear, Citation2000; Vijayakumar et al., Citation2018). In the brain, steroid hormones such as cortisol can directly influence neural structure and function by inducing synaptogenesis, dendritic remodeling, neurogenesis, and cell death (McEwen, Citation2008). Although some basal levels of glucocorticoids are required for proper neural development (McEwen, Citation2008), brain regions involved in emotionality and regulating stress responsiveness (e.g. hippocampus, amygdala, and prefrontal cortex) are highly sensitive to stress hormones. Excessive cortisol exposure can cause dysregulated maturation of these regions and potentially impair adult functionality (Dedovic et al., Citation2009; Lo Iacono and Carola, Citation2018). For this reason, chronic stress experienced in early life and adolescence has longer-term, more permanent impacts on the HPA axis and neuroendocrine development than the same stressors experienced in adulthood (Lupien et al., Citation2009). Thus, even though individuals of all ages have experienced psychological distress and impaired well-being during COVID-19 lockdowns (Achterberg et al., Citation2021; McGinty et al., Citation2020; Patrick et al., Citation2020), youth may be particularly vulnerable to more permanent changes to the brain and HPA axis from chronic cortisol exposure during the sensitive period for adolescent neuroendocrine development.
The lasting impacts of chronic adolescent stress are of particular significance, as self-reported distress in the present study was not associated with physiological changes in HCC. An individual’s subjective appraisal of a stressor often does not directly predict the subsequent degree of HPA axis activation, both in acute and chronic stress conditions (Campbell and Ehlert, Citation2012; O'Brien et al., Citation2013). Some youth may not have reported the pandemic as a highly stressful event; however, this lack of endorsed distress does not necessarily signify those same individuals did not mount a substantial cortisol response. This disconnect between perceived stress levels and biological cortisol activity suggests youth may still be susceptible to the deleterious effects of chronic cortisol exposure across the pandemic, even if these youth did not subjectively report the pandemic as highly stressful. Regardless of whether sustained biological stress is momentarily or evolutionarily beneficial, the present results suggest that chronically elevated cortisol has the potential to impair the development of several physical and psychological systems, and youth’s subjective reports of their pandemic-related stress levels cannot accurately predict which individuals may be more vulnerable in developing these downstream problems. This inconsistency is problematic, as most diagnostic and therapeutic distinctions focus on subjective reporting. Children and adolescents may not perceive certain experiences as highly stressful; nonetheless, they are not immune to concurrently developing physiological consequences. Although perceived distress is a primary motivator for seeking therapeutic treatment, and subsequent interventions may alleviate these initial experiences of stress, these practices do not address the potential for problematic development stemming from chronic exposure to biological stress responses. Of note, previous studies of pandemic-related stress have only examined how changes in cortisol relate to parent-reports of child behavioral health (Perry et al., Citation2022). It is possible that a parent’s report of their children’s behavior, at least in part reflects their own feelings of stress and anxiety, rather than being an unbiased measure of youth wellbeing. Thus, our findings fill this critical gap in the literature by examining the direct relationship between youth’s physiological and psychological pandemic-related stress responses, without parental bias, which corroborates a lack of association between physiological changes in youth HCC and self-reported perceptions of pandemic-related stress.
Though the present study looked at physiological changes in stress during the pandemic, it is important to integrate these findings into the broader context of youth mental health and development. Adolescence is a unique period of social and cognitive behavioral maturation (Sisk & Foster, Citation2004). Specifically, peer acceptance, rejection, social isolation, and exclusion have greater impacts on adolescent well-being than that of children or adults (Orben et al., Citation2020). Social relationships can directly impact health outcomes and proper social development, at both behavioral and brain levels (Burke et al., Citation2017). Loneliness and social deprivation during adolescent development is associated with the emergence of mental health disorders and elevated cortisol levels (Burke et al., Citation2017; Loades et al., Citation2020), where the duration of loneliness aligns with the future severity of mental health symptoms (Loades et al., Citation2020). This may be of particular concern for youth due to the extensive physical and social isolation enforced during the pandemic, especially since “not seeing friends in person” was the highest endorsed stressor in the present study. Emotional and behavioral problems also commonly emerge during adolescence, and dysregulated HPA hormones and stress-induced alterations to stress-reactive brain regions have been associated with mental health problems (Marceau et al., Citation2013; Oyola & Handa, Citation2017), including internalizing, substance use, and anxiety disorders (Chaplin et al. Citation2018; Marceau et al., Citation2013; Shirtcliff & Essex, Citation2008; Taylor et al., Citation2020). Chronic stress has also negatively impacted mental health following other natural disasters and pandemics (Esterwood & Saeed, Citation2020; Fletcher, Citation2003). Since youth have already reported declines in mental health during the COVID-19 lockdowns (Bignardi et al., Citation2020; Liang et al., Citation2020) and are exhibiting dramatic physiological stress responses in the present study, it will be imperative to track the potential emergence of a plethora of mental health problems. Providing adequate access to treatments and interventions, as well as encouraging preventative resources for youth that are at high risk for developing mental health problems will be crucial to protecting proper neuroendocrine maturation in youth.
Though hormonal response profiles can exhibit substantial variability between individuals, the overall trend of increasing cortisol across the pandemic was significant across the entire youth sample. For example, there is vast individual variability in the timing and tempo of pubertal hormonal maturation (Sisk & Foster, Citation2004) and various factors, such as genetics, can impact basal cortisol levels (Bartels et al., Citation2003; Knutsson et al., Citation1997; Marceau et al., Citation2013). As this variability likely existed in the initial sample, the use of random linear mixed-effects models allowed for the initial, pre-pandemic cortisol levels (i.e. intercept) and different trajectories of cortisol change during the pandemic (i.e. slope) to vary across individuals. Of note, since the best fitting model had random slopes and random intercepts, this suggests some uniformity at the group level, but enough individual variability to make the random effects important to consider, as the pandemic-related increases in cortisol (and decreases in some individuals) were not fully homogenous across the sample. Thus, the sample comprehensively showed augmented stress during COVID-19 above and beyond individual differences in both initial cortisol levels and unique stress response patterns.
It is also important to note that the cortisol trajectory did not interact with age or sex, despite data showing that HPA axis activity changes across puberty with some aspects maturing in a sexually dimorphic manner (Gray et al., Citation2018; McLaughlin et al., Citation2009; Stalder et al., Citation2017). This is consistent with the current measurement window; it is improbable long-term pubertal changes would be observed over four months. Further, gonadal hormones have been proposed to mediate sex-specific structural changes in response to stress and slight sex differences in HPA regulation emerge in puberty (McLaughlin et al., Citation2009); these are potential underlying sources for the different vulnerabilities between males and females for developing stress-related mental disorders in adolescence, such as anxiety and depression (Natsuaki et al., Citation2009). Though there were no sex differences in physiological responses to COVID-19 stress, the general elevations in cortisol may impact downstream developmental processes uniquely in different individuals.
Before closing, it is important to acknowledge limitations of the present study. Though HCC provides great measures of longitudinal cortisol secretion, these retrospective analyses can only measure a few months prior to the date of sample collection due to the natural reduction of hair integrity with increasing environmental exposure. For example, frequency of hair washing and use of hair products could alter HCC, and though these factors have shown minimal impact on the most proximal hair from the scalp (Gray et al., Citation2018; Russell et al., Citation2012), different environmental factors could cause some variability in measurable HCC levels. Of note, no hair-related measures (i.e. hair type, color, treatment, etc.) were associated with HCC in the present study, though a natural proximal-to-distal decline in HCC cannot be entirely ruled out when using a segmental approach to conduct hair hormone analyses. Additionally, the novel home collection of hair samples was not supervised, and although instructions for the validated collection methods were provided to parents, there was likely some variability in protocol adherence. The present study also had a modest sex bias, as males tend to have shorter haircuts preventing analyses over several months. The modest sample size also may not have been sensitive enough to observe smaller effects, such as interactions with development and sex. Future work investigating physiological responses to chronic stress with larger samples may be able to detect these patterns. Finally, more extensive pre-pandemic measures of stress and mental health function would have strengthened the study and allowed more far-reaching conclusions regarding risk factors for more severe responses to the pandemic-related stressors.
In conclusion, the present study investigated changes in stress in typically-developing youth during the COVID-19 lockdowns by measuring HCCs and perceived COVID-19-related distress across the inception of the pandemic. HCC systematically increased above-and-beyond individual differences in initial cortisol levels and stress response patterns, though these cortisol changes did not predict subjective levels of perceived stress. This is highly concerning. Clinical assessments are vastly reliant on perceived symptomology, and subjective distress drives individuals to treatment. Current methods of assessing risk for future psychopathology following stressful experiences, including pandemic exposure, may be failing to detect vast neuroendocrine changes. This has important implications for future mental health, and thus our findings highlight the importance of integrating biological findings with clinical practices. Though the severity of physical and social distancing restrictions may begin to wane with more widespread vaccine administration (52), it will be an international priority to promote healthy psychological and neuroendocrine development in youth and help mitigate the unpredictable consequences of COVID-19-related stress for years to come.
Author contributions
Madison H. Fung: Conceptualization, Formal Analysis, Methodology, Software, Visualization, Writing – Original Draft, Writing – Review & Editing; Brittany K. Taylor: Conceptualization, Supervision, Writing – Review & Editing; Christine M. Embury: Methodology, Software, Writing – Review & Editing; Rachel K. Spooner: Methodology, Writing – Review & Editing; Hallie J. Johnson, Madelyn P. Willett, Michaela R. Frenzel: Investigation, Project Administration; Amy S. Badura-Brack, Stuart F. White: Conceptualization, Funding Acquisition, Writing – Review & Editing; Tony W. Wilson: Conceptualization, Funding Acquisition, Supervision, Writing – Review & Editing
Supplemental Material
Download MS Word (27.9 KB)Acknowledgements
The authors thank Elizabeth Shirtcliff, PhD, Wen Wang, PhD, Lotte van Dammen, PhD, and the whole Stress Physiology Investigative Team (SPIT) lab at Iowa State University and the University of Oregon for their consulting efforts, and for processing the hair cortisol data for the present study.
Disclosure statement
All authors report no financial interests or conflicts of interest. All data that support the findings of this study are available upon reasonable request to the corresponding author (TWW). Data will be made publicly available upon study completion.
Additional information
Funding
Notes on contributors
Madison H. Fung
Madison H. Fung is a graduate PhD student in Developmental Psychology in the Institute of Child Development at the University of Minnesota, and was a research assistant in the Dynamic Imaging of Cognition and Neuromodulation (DICoN) Laboratory at the Institute for Human Neuroscience at Boys Town National Research Hospital.
Brittany K. Taylor
Dr. Brittany K. Taylor is the Director of the Neurodiversity Laboratory at the Institute for Human Neuroscience at Boys Town National Research Hospital.
Christine M. Embury
Dr. Christine M. Embury is a postdoctoral fellow in the Dynamic Imaging of Cognition and Neuromodulation (DICoN) Laboratory at the Institute for Human Neuroscience at Boys Town National Research Hospital.
Rachel K. Spooner
Dr. Rachel K. Spooner is a postdoctoral fellow in the Institute of Clinical Neuroscience and Medical Psychology at Heinrich-Heine University, and was a PhD neuroscience student in the Dynamic Imaging of Cognition and Neuromodulation (DICoN) Laboratory at the Institute for Human Neuroscience at Boys Town National Research Hospital.
Hallie J. Johnson
Hallie J. Johnson was a research assistant in the Dynamic Imaging of Cognition and Neuromodulation (DICoN) Laboratory at the Institute for Human Neuroscience at Boys Town National Research Hospital. She is now a graduate student in clinical psychology at Widener University.
Madelyn P. Willett
Madelyn P. Willett was a research assistant in the Dynamic Imaging of Cognition and Neuromodulation (DICoN) Laboratory at the Institute for Human Neuroscience at Boys Town National Research Hospital. She is now a graduate student in the speech-language pathology program at Cal State East Bay University.
Michaela R. Frenzel
Michaela R. Frenzel was a research coordinator in the Dynamic Imaging of Cognition and Neuromodulation (DICoN) Laboratory at the Institute for Human Neuroscience at Boys Town National Research Hospital.
Amy S. Badura-Brack
Dr. Amy S. Badura-Brack is a Professor of Psychological Science at Creighton University.
Stuart F. White
Dr. Stuart F. White is the Director of the Developmental Clinical Neuroscience Laboratory in the Institute for Human Neuroscience at Boys Town National Research Hospital.
Tony W. Wilson
Dr. Tony W. Wilson is the Director of the Institute for Human Neuroscience and holds the Patrick E. Brookhouser Endowed Chair in Cognitive Neuroscience. He is also the Director of the Dynamic Imaging of Cognition and Neuromodulation (DICoN) Laboratory at Boys Town National Research Hospital.
References
- Achterberg, M., Dobbelaar, S., Boer, O. D., & Crone, E. A. (2021). Perceived stress as mediator for longitudinal effects of the COVID-19 lockdown on wellbeing of parents and children. Scientific Reports, 11(1), 2971. https://doi.org/10.1038/s41598-021-81720-8
- Bartels, M., Van den Berg, M., Sluyter, F., Boomsma, D. I., & de Geus, E. J. C. (2003). Heritability of cortisol levels: Review and simultaneous analysis of twin studies. Psychoneuroendocrinology, 28(2), 121–137. https://doi.org/10.1016/S0306-4530(02)00003-3
- Bignardi, G., Dalmaijer, E. S., Anwyl-Irvine, A. L., Smith, T. A., Siugzdaite, R., & Uh, S. (2020). Longitudinal increases in childhood depression symptoms during the COVID-19 lockdown. Arch Dis Child, 1–7.
- Burke, A., McCormick, C., Pellis, S., & Lukkes, J. (2017). Impact of adolescent social experiences on behavior and neural circuits implicated in mental illnesses. Neuroscience and Biobehavioral Reviews, 76:280–300.
- Campbell, J., & Ehlert, U. (2012). Acute psychosocial stress: Does the emotional stress response correspond with physiological responses? Psychoneuroendocrinology, 37(8), 1111–1134. https://doi.org/10.1016/j.psyneuen.2011.12.010
- CDC (2020). COVID Data Tracker [Internet]. Centers for Disease Control and Prevention. https://covid.cdc.gov/covid-data-tracker
- Chaplin, T. M., Niehaus, C., & Gonçalves, S. F. (2018). Stress reactivity and the developmental psychopathology of adolescent substance use. Neurobiology of Stress, 9, 133–139. https://doi.org/10.1016/j.ynstr.2018.09.002
- Dajani, R., Hadfield, K., van Uum, S., Greff, M., & Panter-Brick, C. (2018). Hair cortisol concentrations in war-affected adolescents: A prospective intervention trial. Psychoneuroendocrinology, 89, 138–146. https://doi.org/10.1016/j.psyneuen.2017.12.012
- Dantzer, R., Heuser, I., & Lupien, S. (2020). Covid-19: An urgent need for a psychoneuroendocrine perspective. Psychoneuroendocrinology, 116, 104703. https://doi.org/10.1016/j.psyneuen.2020.104703
- Dedovic, K., Duchesne, A., Andrews, J., Engert, V., & Pruessner, J. C. (2009). The brain and the stress axis: The neural correlates of cortisol regulation in response to stress. NeuroImage, 47(3), 864–871. https://doi.org/10.1016/j.neuroimage.2009.05.074
- Esterwood, E., & Saeed, S. A. (2020). Past epidemics, natural disasters, COVID19, and mental health: Learning from history as we deal with the present and prepare for the future. The Psychiatric Quarterly, 91(4), 1121–1133. https://doi.org/10.1007/s11126-020-09808-4
- Faul, F., Erdfelder, E., Lang, A. G., & Buchner, A. (2007). G*Power 3: A flexible statistical power analysis program for the social, behavioral, and biomedical sciences. Behavior Research Methods, 39(2), 175–191. https://doi.org/10.3758/bf03193146
- Fletcher, K. E. (2003). Childhood posttraumatic stress disorder. In E. J. Mash & R. A. Barkley (Eds.), Child psychopathology, 2nd ed. Guilford Press. p. 330–371.
- Fox, S. E., Levitt, P., & Nelson, C. A. (2010). How the timing and quality of early experiences influence the development of brain architecture. Child Development, 81(1), 28–40. https://doi.org/10.1111/j.1467-8624.2009.01380.x
- Gray, N. A., Dhana, A., Van Der Vyver, L., Van Wyk, J., Khumalo, N. P., & Stein, D. J. (2018). Determinants of hair cortisol concentration in children: A systematic review. Psychoneuroendocrinology, 87, 204–214. https://doi.org/10.1016/j.psyneuen.2017.10.022
- Gunnar, M. R., Wewerka, S., Frenn, K., Long, J., & Griggs, C. (2009). Developmental changes in hypothalamus-pituitary-adrenal activity over the transition to adolescence: Normative changes and associations with puberty. Development and Psychopathology, 21(1), 69–85. https://doi.org/10.1017/S0954579409000054
- Hunter, A. L., Minnis, H., & Wilson, P. (2011). Altered stress responses in children exposed to early adversity: A systematic review of salivary cortisol studies. Stress (Amsterdam, Netherlands), 14(6), 614–626. https://doi.org/10.3109/10253890.2011.577848
- Knutsson, U., Dahlgren, J., Marcus, C., Rosberg, S., Nnegård, M. B., & Stierna, P. (1997). Circadian Cortisol Rhythms in Healthy Boys and Girls: Relationship with Age. Growth, Body Composition, and Pubertal Development, 82(2), 5.
- Ladouceur, C. (2020). COVID-19 Adolescent Symptom & Psychological Experience Questionnaire (CASPE).
- Liang, L., Ren, H., Cao, R., Hu, Y., Qin, Z., Li, C., & Mei, S. (2020). The effect of COVID-19 on youth mental health. Psychiatric Quarterly, 91(3), 841–852. https://doi.org/10.1007/s11126-020-09744-3
- Lo Iacono, L., & Carola, V. (2018). The impact of adolescent stress experiences on neurobiological development. Seminars in Cell & Developmental Biology, 77, 93–103. https://doi.org/10.1016/j.semcdb.2017.09.040
- Loades, M. E., Chatburn, E., Higson-Sweeney, N., Reynolds, S., Shafran, R., Brigden, A., Linney, C., McManus, M. N., Borwick, C., & Crawley, E. (2020). Rapid systematic review: the impact of social isolation and loneliness on the mental health of children and adolescents in the context of COVID-19. Journal of the American Academy of Child and Adolescent Psychiatry, 59(11), 1218–1239.e3. https://doi.org/10.1016/j.jaac.2020.05.009
- Ludvigsson, J. F. (2020). Systematic review of COVID-19 in children shows milder cases and a better prognosis than adults. Acta Paediatrica (Oslo, Norway : 1992), 109(6), 1088–1095. https://doi.org/10.1111/apa.15270
- Luo, H., Hu, X., Liu, X., Ma, X., Guo, W., Qiu, C., Wang, Y., Wang, Q., Zhang, X., Zhang, W., Hannum, G., Zhang, K., Liu, X., & Li, T. (2012). Hair cortisol level as a biomarker for altered hypothalamic-pituitary-adrenal activity in female adolescents with posttraumatic stress disorder after the 2008 wenchuan earthquake. Biological Psychiatry, 72(1), 65–69. https://doi.org/10.1016/j.biopsych.2011.12.020
- Lupien, S. J., McEwen, B. S., Gunnar, M. R., & Heim, C. (2009). Effects of stress throughout the lifespan on the brain, behaviour and cognition. Nature Reviews. Neuroscience, 10(6), 434–445. https://doi.org/10.1038/nrn2639
- Education Week. (2020). Map: coronavirus and school closures in 2019–2020. https://www.edweek.org/leadership/map-coronavirus-and-school-closures-in-2019-2020/2020/03
- Marceau, K., Ram, N., Neiderhiser, J. M., Laurent, H. K., Shaw, D. S., Fisher, P., Natsuaki, M. N., & Leve, L. D. (2013). Disentangling the effects of genetic, prenatal and parenting influences on children’s cortisol variability. Stress (Amsterdam, Netherlands), 16(6), 607–615. https://doi.org/10.3109/10253890.2013.825766
- Matchock, R. L., Dorn, L. D., & Susman, E. J. (2007). Diurnal and seasonal cortisol, testosterone, and DHEA rhythms in boys and girls during puberty. Chronobiology International, 24(5), 969–990. https://doi.org/10.1080/07420520701649471
- McEwen, B. S. (1998). Stress, adaptation, and disease: Allostasis and allostatic load. Annals of the New York Academy of Sciences, 840(1), 33–44. https://doi.org/10.1111/j.1749-6632.1998.tb09546.x
- McEwen, B. S. (2008). Central effects of stress hormones in health and disease: Understanding the protective and damaging effects of stress and stress mediators. European Journal of Pharmacology, 583(2-3), 174–185. https://doi.org/10.1016/j.ejphar.2007.11.071
- McGinty, E. E., Presskreischer, R., Anderson, K. E., Han, H., & Barry, C. L. (2020). Psychological distress and COVID-19–related stressors reported in a Longitudinal Cohort of US Adults in April and July 2020. JAMA, 324(24), 2555–2557. https://doi.org/10.1001/jama.2020.21231
- McLaughlin, K. J., Baran, S. E., & Conrad, C. D. (2009). Chronic stress- and sex-specific neuromorphological and functional changes in limbic structures. Molecular Neurobiology, 40(2), 166–182. https://doi.org/10.1007/s12035-009-8079-7
- Natsuaki, M. N., Klimes-Dougan, B., Ge, X., Shirtcliff, E. A., Hastings, P. D., & Zahn-Waxler, C. (2009). Early pubertal maturation and internalizing problems in adolescence: sex differences in the role of cortisol reactivity to interpersonal stress. Journal of Clinical Child and Adolescent Psychology : The Official Journal for the Society of Clinical Child and Adolescent Psychology, American Psychological Association, Division 53, 38(4), 513–524. https://doi.org/10.1080/15374410902976320
- Nezlek, J. B. (2012). Multilevel modeling for psychologists. In APA handbook of research methods in psychology., Vol 3: Data analysis and research publication American Psychological Association. p. 219–241.
- O'Brien, K. M., Tronick, E. Z., & Moore, C. L. (2013). Relationship between Hair cortisol and perceived chronic stress in a diverse sample. Stress and Health : journal of the International Society for the Investigation of Stress, 29(4), 337–344. https://doi.org/10.1002/smi.2475
- Orben, A., Tomova, L., & Blakemore, S. J. (2020). The effects of social deprivation on adolescent development and mental health. The Lancet. Child & Adolescent Health, 4(8), 634–640. https://doi.org/10.1016/S2352-4642(20)30186-3
- Oyola, M. G., & Handa, R. J. (2017). Hypothalamic–pituitary–adrenal and hypothalamic–pituitary–gonadal axes: sex differences in regulation of stress responsivity. Stress (Amsterdam, Netherlands), 20(5), 476–494. https://doi.org/10.1080/10253890.2017.1369523
- Patrick, S. W., Henkhaus, L. E., Zickafoose, J. S., Lovell, K., Halvorson, A., Loch, S., Letterie, M., & Davis, M. M. (2020). Well-being of parents and children during the COVID-19 pandemic: A national survey. Pediatrics, 146(4), e2020016824. https://doi.org/10.1542/peds.2020-016824
- Perry, N. B., Donzella, B., Troy, M. F., & Barnes, A. J. (2022). Mother and child hair cortisol during the COVID-19 pandemic: Associations among physiological stress, pandemic-related behaviors, and child emotional-behavioral health. Psychoneuroendocrinology, 137, 105656. https://doi.org/10.1016/j.psyneuen.2021.105656
- Pinheiro, J., Bates, D., DebRoy, S., & Sarkar, D. (2021). nlme: Linear and Nonlinear Mixed Effects Models. R Package Version, 3, 1–152.
- Russell, E., Koren, G., Rieder, M., & Van Uum, S. (2012). Hair cortisol as a biological marker of chronic stress: Current status, future directions and unanswered questions. Psychoneuroendocrinology, 37(5), 589–601. https://doi.org/10.1016/j.psyneuen.2011.09.009
- Sapolsky, R. M. (2000). Glucocorticoids and hippocampal atrophy in neuropsychiatric disorders. Archives of General Psychiatry, 57(10), 925–935. https://doi.org/10.1001/archpsyc.57.10.925
- Shirtcliff, E. A., & Essex, M. J. (2008). Concurrent and longitudinal associations of basal and diurnal cortisol with mental health symptoms in early adolescence. Developmental Psychobiology, 50(7), 690–703. https://doi.org/10.1002/dev.20336
- Short, S. J., Stalder, T., Marceau, K., Entringer, S., Moog, N. K., Shirtcliff, E. A., Wadhwa, P. D., & Buss, C. (2016). Correspondence between hair cortisol concentrations and 30-day integrated daily salivary and weekly urinary cortisol measures. Psychoneuroendocrinology, 71, 12–18. https://doi.org/10.1016/j.psyneuen.2016.05.007
- Sisk, C. L., & Foster, D. L. (2004). The neural basis of puberty and adolescence. Nature Neuroscience, 7(10), 1040–1047. https://doi.org/10.1038/nn1326
- Smith, S. M., & Vale, W. W. (2006). The role of the hypothalamic-pituitary-adrenal axis in neuroendocrine responses to stress. Dialogues in Clinical Neuroscience, 8(4), 383–395. https://doi.org/10.31887/DCNS.2006.8.4/ssmith
- Spear, L. P. (2000). The adolescent brain and age-related behavioral manifestations. Neuroscience and Biobehavioral Reviews, 24(4), 417–463. https://doi.org/10.1016/s0149-7634(00)00014-2
- Stalder, T., Steudte-Schmiedgen, S., Alexander, N., Klucken, T., Vater, A., Wichmann, S., Kirschbaum, C., & Miller, R. (2017). Stress-related and basic determinants of hair cortisol in humans: A meta-analysis. Psychoneuroendocrinology, 77, 261–274. https://doi.org/10.1016/j.psyneuen.2016.12.017
- Staufenbiel, S. M., Penninx, B. W. J. H., Spijker, A. T., Elzinga, B. M., & van Rossum, E. F. C. (2013). Hair cortisol, stress exposure, and mental health in humans: A systematic review. Psychoneuroendocrinology, 38(8), 1220–1235. https://doi.org/10.1016/j.psyneuen.2012.11.015
- Stephen, J. M., Solis, I., Janowich, J., Stern, M., Frenzel, M. R., Eastman, J. A., Mills, M. S., Embury, C. M., Coolidge, N. M., Heinrichs-Graham, E., Mayer, A., Liu, J., Wang, Y. P., Wilson, T. W., & Calhoun, V. D. (2021). The Developmental Chronnecto-Genomics (Dev-CoG) study: A multimodal study on the developing brain. NeuroImage, 225, 117438. https://doi.org/10.1016/j.neuroimage.2020.117438
- Taylor, B. K., Eastman, J. A., Frenzel, M. R., Embury, C. M., Wang, Y. P., & Stephen, J. M. (2020). Subclinical anxiety and posttraumatic stress influence cortical thinning during adolescence. Journal of the American Academy of Child and Adolescent Psychiatry, 60(10):1288–1299.
- Vijayakumar, N., Op de Macks, Z., Shirtcliff, E. A., & Pfeifer, J. H. (2018). Puberty and the human brain: Insights into adolescent development. Neuroscience and Biobehavioral Reviews, 92, 417–436. https://doi.org/10.1016/j.neubiorev.2018.06.004