Abstract
Bombesin receptor-activated protein (BRAP) and its homologous protein in mice, which is encoded by bc004004 gene, were expressed abundantly in brain tissues with unknown functions. We treated bc004004-/- mice with chronic unpredictable mild stress (CUMS) to test whether those mice were more vulnerable to stress-related disorders. The results of forced swimming test, sucrose preference test, and open field test showed that after being treated with CUMS for 28 days or 35 days both bc004004-/- and bc004004+/+ mice exhibited behavioural changes and there was no significant difference between bc004004+/+ and bc004004-/-. However, behavioural changes were observed only in bc004004-/- mice after being exposed to CUMS for 21 days, but not in bc004004+/+ after 21-day CUMS exposure, indicating that lack of BRAP homologous protein may cause vulnerability to stress-related disorders in mice. In addition, bc004004-/- mice showed a reduction in recognition memory as revealed by novel object recognition test. Since memory changes and stress related behavioural changes are all closely related to the hippocampus function we further analyzed the changes of dendrites and synapses of hippocampal neurons as well as expression levels of some proteins closely related to synaptic function. bc004004-/- mice exhibited decreased dendritic lengths and increased amount of immature spines, as well as altered expression pattern of synaptic related proteins including GluN2A, synaptophysin and BDNF in the hippocampus. Those findings suggest that BRAP homologous protein may have a protective effect on the behavioural response to stress via regulating dendritic spine formation and synaptic plasticity in the hippocampus.
1. Introduction
Nervous tissue has a tremendous capacity of plasticity. In response to intrinsic or extrinsic stimuli neural networks change based upon synapse regulation and reorganization of circuitry. Psychosocial stressors or stressful life events have been shown to have profound effects on neural structure and function in susceptible individuals. For example, neuroplasticity hypothesis, which was proposed for understanding the mechanisms of the development of depression, incorporates the continually remodeling of key brain systems in response to various situations. It suggests that a disrupt or dysfunction of neural plasticity contributes to behaviors related to depression (Duman et al., Citation2016; B. Liu et al., Citation2017; Pittenger & Duman, Citation2008; Racagni & Popoli, Citation2008). Typical antidepressants may improve neuroplasticity through monoamine neurotransmitters’ stimulation of the postsynaptic monoamine receptors (Racagni & Popoli, Citation2008). In addition, other treatments such as psychotherapy, cognitive behavioral therapy and electroconvulsive shock treatment may also have therapeutic effects on depression through regulating neural plasticity in experimental animals (B. Liu et al., Citation2017).
Cognitive impairment is considered as an important endophenotype of mood disorders. Some brain regions which play critical roles in learning and memory were found to be involved in the pathophysiology of some stress-related mood disorders such as depression. Those brain regions include the dorsolateral prefrontal cortex (DLPFC), the hippocampus, the medial temporal lobe, the nucleus accumbens and the ventral tegmental area (VTA) (Baxter et al., Citation1989; Harvey et al., Citation2005; Lisman & Grace, Citation2005; Squire et al., Citation2004; Stockmeier et al., Citation2004; Warner-Schmidt & Duman, Citation2006). Among those regions, the hippocampus is of particular interest since the hippocampal sizes in patients with depression were shown to be decreased and stress also caused the hippocampus atrophy in animal models of depression (MacQueen et al., Citation2003; Sapolsky, Citation2000). Furthermore, the hippocampus exhibited many changes in neural plasticity in stress-induced depression models (Pittenger & Duman, Citation2008; Sapolsky, Citation2003). However, stress induced neural plasticity involved in the pathophysiology of mood disorders including depression is still not understood well due to the difficulty of analyzing behavior and the behavior-related molecular events in humans or animal models.
In our recent studies, we have found the homologous protein of bombesin receptor-activated protein (BRAP) might be involved in the development of mood disorders in mice. BRAP, encoded by C6orf89 in human, is a protein of 354 amino acids, and contains a transmembrane sequence within its N terminus. It was found by our group in a bacteria two-hybrid search as a potential partner that interacts with bombesin receptor subtype-3 (BRS-3), an orphan receptor belonging to mammalian bombesin receptor family (H. J. Liu et al., Citation2011;Qu et al., Citation2013) . BRAP shares about 83% identity to its homologous protein in mice, which is encoded by bc004004 gene. In our previous studies, we found that overexpression of BRAP could inhibit the transcriptional activity of NF-κB in cultured human bronchial epithelial cells and suppress the antigen uptake ability of those cells (Y. Liu et al., Citation2016). In order to further elucidate the mechanisms underlying its biological role, we constructed a gene knockout mouse bc004004−/− in which bc004004 was disrupted by CRISPR/Cas9-mediated genome engineering (Wang et al., Citation2022). When maintaining and handling those mice we observed reduced aggressive behaviors in the knockout mice. Further behavioral tests suggested that the male bc004004−/− mice exhibited mild anxiety-like behaviors. And western blot analysis of brain tissues revealed that the homologous protein of BRAP was also expressed in mouse brain, indicating a potential role of this protein in nervous system. Therefore, we treated bc004004−/− mice with chronic unpredictable mild stress (CUMS) to examine the possible role of BRAP homologous protein in mood disorders. By using this model we found that mice deficient in BRAP homologous protein exhibited vulnerability to stress-related disorders. Furthermore, lack of BRAP homologous protein led to changes in synapses of hippocampus in mice, which might contribute to behavioral changes in those mice induced by stressful events. Our findings of the current study provide evidence that BRAP homologous protein plays a role in brain function.
2. Materials and methods
2.1. Animals
All mice used in this study were on C57BL/6 background and approved by Xiangya animal care and use committee at Central South University. CRISPR/Cas9-mediated genome engineering was used to knock out bc004004 gene in mice to create bc004004−/− mice in our previous study (Wang et al., Citation2022). 8 weeks old male bc004004−/− mice and their isogenic control C57BL/6 mice (bc004004+/+), weighing between 20 and 25 g, were used in this study. The parental mice of bc004004−/− used in this study were heterozygous mice (bc004004+/-). The genotypes of the offspring of those heterozygous mice were determined by PCR to select homozygous (bc004004−/−) for further study (Wang et al., Citation2022). All mice were maintained on a 12-h light/dark cycle with free access to water and food unless a particular stressor required otherwise. From the first day of CUMS treatment mice were single- housed in cages (32 × 16 × 16cm, L × W × H) kept in a room with controlled temperature (22 ∼ 23 °C) and humidity (50 ∼ 60%).
2.2. CUMS used to induce behavioral changes in mice
Mice were randomly divided into CUMS treatment and CUMS-absent groups. Then CUMS treatment was used to induce behavioral changes in CUMS groups (bc004004+/++CUMS and bc004004−/−+CUMS), as described in previous studies (Biala et al., Citation2017; Nollet et al., Citation2013). CUMS treatment was absent for NO CUMS groups (bc004004+/+−CUMS and bc004004−/− −CUMS).
CUMS stressors included reversed light/dark cycle (12-h dark/12-h light instead of normal 12-h light/12-h dark), food deprivation for 24 h, water deprivation for 24 h, damping sawdust for 24 h, tail clamping for 3 min, restraint for 2 h, and level shaking for 10 min. CUMS groups were randomly exposed to one of those stressors every day. Each stressor was applied for at least three times to one mouse over the duration of 21 day-CUMS treatment or five times to one mouse over 35 days of treatment.
2.3. Behavioral tests in mice
2.3.1. Elevated plus maze test (EPM)
The EPM is a behavioral test measuring anxiety -like behavior in rodents. It is based on the animal’s aversion to open spaces and exploratory behavior in novel environments. The apparatus is an elevated plus maze made of plastic materials which is 50 cm above the floor. The open arms (29 × 5 × 0.5 cm) are perpendicular to the closed arms (29 × 5 × 21 cm). Mice were transferred to the testing room 30 min prior to beginning of the test to become habituated to the condition of the room. They were given access to all the open arms and closed arms and moved freely between all the arms for 5 min. The test was recorded using a video camera attached to a computer. Mice with anxiety-like behaviors tend to have fewer entries into the open arms or spent less time in the open arms (Horii et al., Citation2018; Walf & Frye, Citation2007).
2.3.2. Open field test (OFT)
The OFT is a test measuring exploratory behavior and gross locomotor activity in rodents. The basic outcome can provide a variety of behavioral information ranging from general activity levels to data regarding the emotionality of the animal such as anxiety and willingness to explore. Exploration of central area is often related to anxiety in rodents and the center distance - total distance ratio can be used as an index of anxiety-related responses. After being acclimated to the testing room for 30 min, a mouse was gently placed in the middle of the open field chamber with dimensions (W × D × H, 400 × 400 × 38 mm) and allowed to explore the apparatus for 5 min. The footage recorded by an overhead camera was analyzed for the parameters including total distance moved and time spent in indicated zones (Kraeuter et al., Citation2019; Seibenhener & Wooten, Citation2015).
2.3.3. Forced swimming test (FST)
Mice were individually placed in an inescapable plexiglas cylinder of 20 cm in diameter and 50 cm in height. The cylinder was filled with water of 20 cm in height to prevent the mouse from touching its paws or tail to the floor of the cylinder. Mouse was forced to swim in the cylinder for 6 min while being recorded by a camera. The immobility time during the last 4 min was then measured by an independent observer (Arauchi et al., Citation2018; Tian et al., Citation2018).
2.3.4. Sucrose preference test (SPT)
The SPT for rodents is based on assumption that the animal’s preference for sweets is proportional to the pleasure that the animal experiences when it consumes them. This test is considered to reflect the animal’s capacity to experience hedonic pleasure evoked by the sweet solution and has been used to investigate the inability to feel pleasure. Mice were housed singly in cages and were habituated to the presence of two drinking bottles (one containing 200 ml of 1% sucrose and the other containing reverse osmosis (RO) filtered water) for two days. Following acclimation, mice in each cage have free access to either 200 ml of 1% sucrose or 200 ml of RO purified water. Bottles were switched every 12 h to eliminate side preference. After 24 h, two bottles were removed and weighed to calculate the consumption of sugar and pure water for each mouse (sucrose preference = sucrose water consumption/total water consumption × 100%) (Pandya et al., Citation2017; Tian et al., Citation2018).
2.3.5. Tail suspension test (TST)
The TST is based on the assumption that when a mouse is subjected to short term inescapable stress it will actively try to escape and then become immobile. Longer period of immobility in the TST indicates that the mouse was in a depressive-like state. Mice were suspended by their tails with adhesive tape (2 cm from the tail tip) above the ground to prevent tail climbing behavior. Each mouse was placed within its own three-walled rectangular compartment (55 cm height × 15 width × 12 cm depth) to prevent them from observing or interacting with each other. The test lasted for 6 min. When the animal stopped struggling and hung immobile it was then considered to have “given up.” Immobility time during the last 4 min was recorded for each mouse (Arauchi et al., Citation2018; Cryan et al., Citation2005).
2.3.6. Novel object recognition task (NOR)
The NOR task is a test for evaluating rodent memories. Rodents have the spontaneous drive to spend more time exploring a novel object than a familiar one. Preferential exploration of the novel object reflects the use of learning and recognition memory. The task comprised three succeeding phases: habituation, familiarization and test. In the habituation phase, mice were placed in the open field box for 10 min to adapt to the apparatus. Then the next day they were placed individually in the same arena with two identical objects (red glass cylinders which had a diameter of 6.6 cm and a height of 4.5 cm) placed in two opposite corners for 10 min (familiarization phase). 24 h later, one of the objects was replaced by a novel object (a transparent elongated sphere-like object with an orange cap which had a diameter of 5.5 cm and a height of 8.5 cm). Then each mouse was placed in the center of the open field box individually for 10 min and was allowed to freely explore those objects. The time spent to explore each object by each mouse was recorded by camera (test phase) (Lapter et al., Citation2009; Pan et al., Citation2017).
2.4. Immunohistochemistry analysis
Paraffined brain tissue sections from four human post mortem brains were obtained from the Department of Pathology, the Second Xiangya Hospital, Central South University. The IRB of School of Basic Medical Science of Central South University has approved the consent and research protocols used in this study.
The immunohistochemistry analysis (IHC) was performed according to the method described previously (Y. Liu et al., Citation2016). Briefly, after being acclimated in 4% paraformaldehyde, the brain tissues were embedded in paraffin. Tissue sections with thickness of 5 µm were deparaffinized, hydrated, and then subjected to antigenic retrieval by incubation with tris-EDTA buffer (10 mM Tris-HCl, 1 mM EDTA solution, 0.05% tween 20, pH 9.0) at 98 °C for 20 min. Sections were treated with 3% H2O2 in deionized water for 10 min to block endogenous peroxidase activity and then incubated with the following primary antibodies overnight at 4 °C with the dilutions indicated respectively: BRAP (Abcam, cat: ab181073, 1:50), brain derived neurotrophic factor (BDNF) (Abcam, cat:ab108319, 1:100), synaptophysin (ABclonal, cat:A6344,1: 150), BDNF/NT-3 growth factors receptor (Trk-B) (Boster, cat: BA0623, 1: 100), glutamate receptor ionotropic, NMDA 2 A (GluN2A) (CUSABIO, cat: CSB-PA14129A0Rb, 1:50), postsynaptic density protein 95 (PSD95) (Sangon Biotech, cat: D261092, 1:50), BRS-3 (SANTA CRUZ, cat: sc-271712, 1:50). The sections were washed with TBS buffer containing 0.025% Triton X-100 and incubated with HRP-conjugated goat anti-rabbit IgG (ZSGB-Bio, cat: PV-6002) for 30 min at room temperature. After being washed with TBS containing 0.025% Triton X-100 for three times, sections were incubated with AEC reagent (ZSGB-Bio, cat: ZLI-9036) for 5 min at room temperature. Sections were counterstained with hematoxylin and mounted in GVA (ZSGB-Bio, cat: ZLI-9551). For immunofluorescence analysis, the sections were visualized using Invitrogen Alexor Fluor Plus 647 after being incubated with goat anti-rabbit fluorescent secondary antibody at a dilution of 1:5000 (Thermo Fisher, cat:A32733), and the nuclei were counterstained with DAPI in immunofluorescence at a dilution of 1:10 (Servicebio, cat: G1012).
The relative quantification of positively staining areas in immunohistochemistry and immunofluorescence assays were analyzed and calculated by ImageJ. The intensity of grayscale image was converted to an OD value, and then the OD threshold was set for each antibody by ImageJ (BRS3 0.37–0.26, synaptophysin: 2.71–0.29, PSD95: 2.71–0.43, GluN2A: 2.71–0.32, BDNF: 0.80–0, Trk-B: 2.71–0.33). The positive OD value was analyzed and the percentages of positive expression area were then calculated.
2.5. Golgi-Cox staining and analysis of dendritic spines
Mice were sacrificed by intraperitoneal injection of lethal dose of phenobarbital in accordance with the Xiangya animal care and protocols by Central South University. Brain tissues from 6 mice of each group were removed immediately and fixed in at least 20 volumes of 4% paraformaldehyde. Then the tissue preparation and Golgi-Cox staining were conducted following the instructions of FD Rapid GolgiStainTM Kit (FD NeuroTechnologies, PK401). Tissues were cut into 200 µm thick sections with a vibratome. The image of hippocampus was obtained by optical microscopy (BX53, Olympus Corporation). Five neurons were selected from CA1 or CA3 regions of each sample. The apical spines on the secondary and tertiary dendrites of the neurons were counted and the length of spines were measured by ImageJ. The dendritic length and number of the branches were calculated according to Sholl analysis (Sholl, Citation1953) using ImageJ software with the NeuronJ plugin.
2.6. Western blot analysis
The hippocampal tissue of the mice were lysed in RIPA lysis buffer (50 mM Tris-HCl, 150 mM NaCl, 1 mM EDTA-Na2, 1%Triton X-100, 1% sodium deoxycholate, 0.1% SDS) (Servicebio, cat: G2002). Protein concentration was measured using the BCA protein assay kit (Beyotime, cat: P0006). After being heated at 95 °C for 10 min, the proteins were fractionated by electrophoresis on 10% polyacrylamide gels and transferred to polyvinylidene fluoride membrane (PVDF). Western blotting was performed as described previously (Y. Liu et al., Citation2016; Qu et al., Citation2013). The transferred PVDF membranes were incubated with the following primary antibodies with dilutions indicated respectively: β-actin (Sigma, cat: A5441, 1:10,000), BDNF (Abcam, cat: ab108319, 1:1000), Trk-B (Boster, cat: BA0623, 1:1000), BRAP (Abcam, cat: ab181073, 1:1000), GluN2A (CUSABIO, cat: CSB-PA14129A0Rb, 1:500), PSD95 (Sangon Biotech, cat: D261092, 1:500), synaptophysin (ABclonal, cat: A6344, 1:1000). The sources of other antibodies are indicated as follows: horseradish peroxidase-labeled goat anti-rabbit antibody (Abcam, cat: Ab6721, 1:5000) and horseradish peroxidase-labeled goat anti-mouse antibody (Abcam, cat: Ab6789, 1:3000).
2.7. Statistical analysis
Statistical analyses were performed in GraphPad Prism 7. In this study, most results of behavioral tests and experiments including dendritic length were designed as two-factorial studies. Two-way ANOVA was used to analyze whether there are effects of two factors (for example, CUMS treatment or genotype). If there was interaction between those two factors, further test were then conducted for the main effect of each factor and Tukey test was used for further comparisons between groups. If no significant interaction was found between two factors, and if there is a main effect for only one factor, multiple comparisons among the treatment means for this factor are considered to be required to identify which specific means differ (Wei et al., Citation2012). In this study, if no significant interaction was found between the two factors (e.g. CUMS and genotype), and the main effect of one factor is significant, the Tukey test was then used to determine if the changes is related to an individual factor. In this case, the effect of one factor on changes is not dependent on the other factor. A p value of .05 or less was considered statistically significant.
3. Results
3.1. Deficiency in BRAP homologous protein expression caused mild anxiety-like behaviors in male mice
IHC analysis was performed to detect the expression of BRAP in sections of human brain tissue samples. As shown in , BRAP was present in neurons within human brain tissue samples using an antibody against human BRAP (EPR13621 Cat. No. ab181073, Abcam). This antibody is a rabbit monoclonal antibody which was produced based on a recombinant fragment containing amino acids 100–250 within human BRAP protein. As described in our previous study we found that this antibody could detect BRAP or its mouse homologous protein by western blotting analysis using protein extracts from both human and mouse tissues (Wang et al., Citation2022). And it could detect BRAP in human tissue sections with IHC analysis, too. However, this antibody could not be used in IHC analysis with mouse samples. Therefore western blot analysis was performed to explore the expression of BRAP homologous protein in mice. The total protein extracts were made from tissues of hippocampus region in mice brain and then subjected for the following western blot. As shown in , BRAP homologous protein was expressed in both wild type bc004004+/+ mice, and heterozygous bc004004+/- mice, but was absent in bc004004−/− mice. The amount of BRAP homologous protein present in heterozygous bc004004+/- mice was much less compared with that of the wild type mice. The presence of BRAP or its homologous protein in brain tissues indicates a potential role in the nervous system.
Figure 1. Deficiency in BRAP homologous protein expression caused mild anxiety-like behaviors in male mice.

Two behavioral tests, EPM and the OFT, were performed to examine the effects of deficiency in BRAP homologous protein on behaviors. In the EPM, both male homozygous (bc004004−/−) and heterozygous (bc004004+/-) exhibited decreased entries into the open arms compared with the control bc004004+/+ (main effect of genotype: F(2, 42) = 8.65, p = .0007), as shown in . However, there was no difference for the entries into the open arms between different female groups. There was a significant interaction between genotype and sex (F(2,42) = 3.73, p = .03). As shown in Supplementary Figure 2, male bc004004−/− and bc004004+/- also exhibited decreased total entries in the EPM compared with male bc004004+/+ while there was no difference for the total entries between different female groups. The percentage of open arm entry was decreased in male bc004004−/− compared with male bc004004+/+. There is no statistical significant main effect of either sex or genotype on the time spent in the open arms in the EPM test as shown in . And no significant interaction between sex and genotype was found on the time spent in the open arms in the EPM test ().
Figure 2. The analysis of behavioral tests after exposure to chronic unpredictable mild stress (CUMS). (A) Body weight changes throughout the CUMS procedure. (B) Immobility time of bc004004+/+ and bc004004−/− mice in the forced swimming test with treatment of CUMS. (C) Analysis of sucrose preference tests during the CUMS procedure. (D) Immobility time of bc004004+/+ and bc004004−/− mice in the tail suspension test after 21-day CUMS exposure. (E) Total distance traveled in the different regions in the open field test after 21-day CUMS exposure. Two-way ANOVA was performed followed by Tukey’s multiple comparisons. (F) The expression of BRAP homologous protein in mice detected by western blot with anti-BRAP antibody. The protein extracts of hippocampus tissues of the mice was used in western blot after 21-day of CUMS treatment. The expression of β-actin from the same sample was used as loading controls. Data are presented as mean ± SD. *p <.05, **p <.01, ***p <.001,****p <.0001, n = 8.

In the OFT (), the effect of genotype on the distance traveled in central area in the OFT is statistically significant (F(2, 42)=3.24, p = .04). The male homozygous bc004004−/− mice exhibited decreased distance traveled over the central area of the apparatus compared with the wild type control bc004004+/+ mice. Since exploration of central area is often related to anxiety in rodents it indicated that male homozygous bc004004−/− mice had more anxiety-related responses compared with the male wild type control bc004004+/+ mice. There was no interaction between genotype and sex, which indicates that the effect of genotype on behavioral changes is not dependent on sex. For the female genotype groups there is no difference on the total distance or the distance traveled in central area. The above results of the EPM and OFT tests suggested that the deficiency of BRAP homologous protein might cause behavioral changes in male mice although those observed changes were mild. We wondered whether chronic stress would cause more prominent behavioral changes in the knockout mice. Therefore, a CUMS procedure was applied to test the effects of lacking BRAP homologous protein on mice behaviors.
3.2. bc004004−/− mice presented more depression-like behaviors after 21-day CUMS exposure
We subjected both male bc004004−/− and bc004004+/+ mice to a 21-day or 35-day CUMS procedure or a control period. The parental mice of bc004004−/− used in this study were heterozygous mice (bc004004+/-). Since no anxiety-like behavioral changes were found for the female bc004004+/--, the development of their offspring were considered not being affected by the maternal behaviors of mothers. We monitored the body weight of those mice before and throughout the CUMS procedure. CUMS exposure caused a decrease of body weight gain for both bc004004+/+ and bc004004−/− mice (). F(1, 7) = 6.51, p = .04 for Day 7, F(1, 7) = 8.82, p = .03 for Day 14; F(1, 7) =30.28, p = .0009 for Day 21, F(1, 7) =14.47, p = .007 for Day 28, F(1, 7) = 47.23, p = .0002 for Day 35. However, the effect of the genotype on body weight changes was not statistically significant. There was no interaction between genotype and CUMS treatment.
A FST test could reflect stress-coping strategy when mice were facing an acute inescapable stress and was used to assess the stress response. In FST test, the main effects of both genotype and CUMS on the immobility time were significant (effect of genotype: F(1, 9) = 15.6, p = .003, effect of CUMS: F(2, 18) = 20.81, p < .0001). However, there was no interaction between genotype and CUMS treatment. Pairwise comparison showed that the immobility time was significantly increased after 35-day CUMS exposure for both bc004004+/+ mice and bc004004−/− mice. But there was no difference between bc004004+/+ mice and bc004004−/− mice after 35-day treatment. While the immobility time of bc004004+/+ mice did not show significant increase after 21-day exposure, bc004004−/− mice did exhibit significant increase on the immobility time after 21-day exposure. Those results suggested that data from Day 21 could be chosen to further analyze the effect of genotype on behavioral changes since the difference between wild type and knockout mice would be diminished when CUMS treatment was prolonged to 35 days, even though longer treatment would cause more prominent behavioral changes in both bc004004+/+ and bc004004−/− groups.
SPT helps to assess the inability to feel pleasure in animal models of depression. In this study, both the 28-day and 35-day CUMS exposures caused decreased sucrose preference in bc004004+/+ mice and bc004004−/− mice although no significant difference were found between bc004004+/+ mice and bc004004−/− mice. But after 21-day CUMS exposure there was no statistical difference between CUMS treatment and no CUMS treatment groups for bc004004+/+ mice as shown in . In contrast to bc004004+/+ mice, CUMS exposure caused a significant decrease in the preference for sucrose solution in bc004004−/− mice. Both main effects of genotype (F(1, 9) = 9.21, p = .01) and 21-day of CUMS treatment (F(1, 9) =8.34, p = .02) were significant. There was interaction between genotype and 21-day of CUMS on the preference for sucrose solution (F(1, 9) = 5.17, p = .04).
Both the FST and the SPT tests suggested that bc004004−/− mice might have behavioral changes after the 21-day treatment whereas their wild type control bc004004+/+ mice had not developed those behavioral changes until CUMS was prolonged to 28 days and 35 days. In TST, neither the main effect of CUMS nor the effect of genotype was significant (). There was interaction between 21-day of CUMS treatment and genotype (F(1, 11) = 4.95, p = .04). In OFT, both effects of CUMS (F(1, 7) = 5.88, p = .04) and genotype (F(1, 7) = 12.12, p = .01) on total distance traveled were significant. There was interaction between CUMS and genotype (F(1, 7) = 6.45, p = .04). Further pairwise comparison revealed that 21-day CUMS exposure led to decreased locomotor activity for bc004004−/− mice, while 21-day CUMS procedure did not cause significant difference for bc004004+/+ mice. This test did not reveal difference of anxiety-related responses between bc004004+/+ mice and bc004004−/− mice after 21-day of exposure since no significant difference of exploration of central area (the distance traveled in central area) was found (data not shown). We also examined the effect of CUMS exposure on the expression level of BRAP homologous protein in hippocampus region by western blot analysis. CUMS did not cause significant change in the expression level of this protein as shown in . The above behavioral tests revealed a consistent tendency for bc004004−/− mice to develop depressive-like behavior on Day 21 after CUMS treatment. Those data suggested that BRAP may play a role in protecting mice from developing depressive-like behaviors under chronic stress to some extent.
3.3. Lack of BRAP homologous protein led to reduction in learning and recognition memory in mice exposed to CUMS
NOR results showed that there was no difference in the total exploring time spent between groups after 21-day of CUMS treatment (, no significant main effect of either factor, and no interaction between CUMS and genotype). Both CUMS (F(1, 7) =5.75 p = .04) and genotype (F(1, 7) = 17.41, p = .004) have main effects on the recognition index. CUMS procedure did not cause significant difference in the recognition index on exploring a novel object for bc004004+/+ mice (). But CUMS treatment caused decreased recognition index for bc004004−/− mice. The interaction between CUMS treatment and genotype on the recognition index on exploring a novel object was found (F(1, 7) = 6.24, p = .04). Those data suggest that lacking BRAP homologous protein in mice may cause cognitive impairment and memory loss in addition to stress related behavioral changes. A link may exist between functions of BRAP and hippocampus region. Therefore, we tried to identify changes in neurons such as dendrites morphology that may affect functions of hippocampal neurons in bc004004−/− mice.
Figure 3. Novel object recognition (NOR) test in mice exposed to 21-day of CUMS treatment.

3.4. Mice lacking BRAP homologous protein displayed dendritic spine alterations in the hippocampus proper
The entire dendritic trees of the hippocampus neurons were stained by Golgi-Cox method and the images of dendrites were obtained by optical microscopy as illustrated in . Representative hippocampal neurons were reconstructed to assess dendritic complexity by Sholl analysis. Length and density of dendritic spines were measured using ImageJ. For the dendritic length of hippocampus neurons in CA1, both CUMS (F(1, 19) =9.77, p = .006) and genotype (F(1, 19) = 17.03, p = .0006) have effects on the dendritic length in CA1. The dendritic length of bc004004−/− mice was much shorter than that of bc004004+/+. CUMS caused decrease on dendritic length in CA1 neurons () only in bc004004+/+. After CUMS exposure no significant difference of dendritic length between bc004004+/+mice and bc004004−/− mice was found. In both bc004004+/+ and bc004004−/− the decrease of dendritic length of neurons caused by CUMS reached the upper limit due to a ceiling effect of CUMS. Since there was already a decrease in the dendritic length bc004004−/− mice compared with bc004004+/+, only bc004004+/+ mice exhibited significant decrease of dendritic length after CUMS was applied. Interaction between 21-day of CUMS treatment and genotype was found (F(1, 19) = 11.71, p = .003). For CA3 neurons, the effect of genotype on dendritic length is significant (F(1, 19) = 18.11, p = .0004). The dendritic length of neurons in CA3 was much shorter in bc004004−/− compared with bc004004+/+ in the absence of CUMS procedure. CUMS did not cause further change in both groups (). There was no interaction between CUMS and genotype.
The density of dendritic spines was analyzed and two-way ANOVA followed by Tukey’s test for multiple comparisons was performed (). For simplicity purposes we indicated the significances of interaction and main effects of CUMS treatment and genotype in the figure and omitted the F values and p values. bc004004−/− mice displayed lower density compared with bc004004+/+ only at 91–101 μm from the cell body in CA1 and at 41 μm from the cell body in CA3 in the absence of CUMS (the first panels of both ). The application of 21-day CUMS decreased the number of dendritic branches substantially at 61 -141 μm from the cell body in the CA1 in both bc004004+/+ and bc004004−/− mice, whereas CUMS did not affect the dendrite branching of neurons of the CA3 in both groups (the second and third panels of both ). In CA1, after 21-day CUMS the dendritic branches of neurons (from 61 μm to 151 μm) in bc004004−/− were significantly fewer than that of their wild type control (the fourth panel of ). In CA3, the number of dendritic branches around 51 μm from soma in bc004004−/− was statistically fewer after CUMS compared with that of bc004004+/+ (the fourth panel of ). Those data indicate that lack of BRAP decreases the density of dendritic branches and thus influences function of the neurons in the hippocampus.
Dendritic spines come in different shapes and sizes. They were classified as “stubby” (short and thick with no neck), “mushroom” (a large head and a constricted neck), or “thin” (thin with a small head) using the geometric measures. This classification is commonly used to assess the proportions of mature and immature spines within a population. We calculated the percentages of each shape of spines including filopodia-like protrusions (“filopodia”). There was no difference on total spine numbers in both bc004004+/+ and bc004004−/− mice with or without CUMS in both CA1 and CA3. ().
Figure 4. Analysis of dendritic morphology of the hippocampal neurons after 21-day of CUMS exposure.
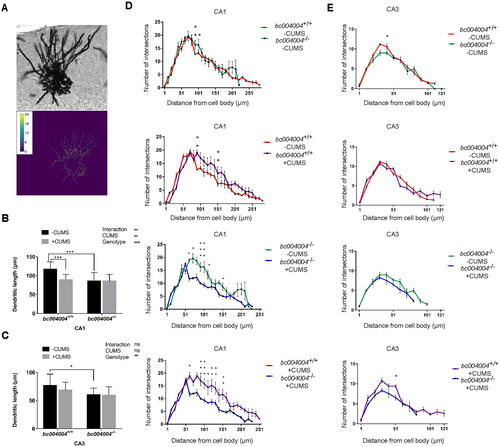
However, in CA1, the percentage of mushroom and “stubby” spines in bc004004−/− was less compared with that of bc004004+/+ in the absence of CUMS. After CUMS treatment the percentage of “stubby” spines in bc004004−/− was less compared with that of bc004004+/+ (). In CA3 field, the percentage of “thin” spines was higher in knockout mice compared with that of bc004004+/+ in the absence of CUMS (). Those observations show that lack of BRAP homologous protein also affects the morphology of spines in addition to changes of dendrites density of the hippocampus neurons and suggest a potential role of this protein in regulating the function of the hippocampal neurons.
3.5. Lack of BRAP homologous protein affected some proteins involved in synapse plasticity in CUMS-induced depression model
Our study suggests that vulnerability of knockout mice to depression caused by stressors may be related to the alterations of the structure of dendrites and shapes of dendrite spines of hippocampal neurons. Dendritic spines usually receive excitatory input from a single axon at the synapse and alterations of their shapes and numbers in knockout mice indicate different pattern of synaptic plasticity may exist in those mice. We examined the expression of some proteins that are important in signal transduction in the neuronal network including synaptophysin, PSD95, GluN2A and in hippocampus tissues.
Synaptophysin is a synaptic vesicle glycoprotein present in nearly all neurons that participate in synaptic transmission. It is often used for quantification of synapses ubiquity at the synapse (Raja et al., Citation2019). Analysis of immunostaining of hippocampal sections showed that the percentage of the area stained by synaptophysin antibody decreased in bc004004−/− mice (). The expression of this protein in the hippocampal tissues analyzed by western blot is shown in . After 21-day of CUMS both main effects of CUMS (F(1, 5) =7.5, p = .04) and genotype (F(1, 5)=13.13, p = .02) on synaptophysin expression were significant. There was interaction between genotype and CUMS treatment (F (1, 5) = 11.97, p = .02). The expression of this protein decreased in knockout mice compared with those of control mice after 21-day of CUMS.
Figure 5. The amount and morphology of different dendritic spines on hippocampal CA1 and CA3 neurons.
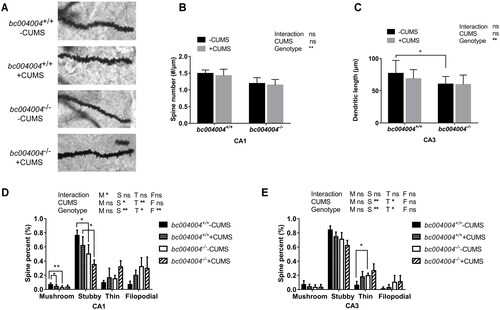
PSD95 is one of the major regulators of synaptic maturation and acts as an essential scaffolding protein during synaptogenesis. It was reported to interact, stabilize and help N-methyl-D-aspartic acid receptors (NMDARs) traffic to the postsynaptic membrane and is required for synaptic plasticity associated with NMDA receptor signaling (Chen et al., Citation2011). No significant loss of PSD95 was observed in hippocampal sections from bc004004−/−mice compared with wild type control mice (). Western blot analysis of PSD95 in the hippocampal tissues is shown in . Although the main effect of genotype (F (1, 5) = 10.54, p = .02) was significant, further pairwise comparison did not find significant difference on PSD95 expression between knockout mice and control mice. The main effect of CUMS treatment (F (1, 5) = 6.55, p = .05) was not significant and there was no interaction between CUMS treatment and genotype (F (1, 5) = 0.39, p = .56).
GluN2A is one of the GluN2-type regulatory subunits which comprise NMDAR tetramers. It is one of the most abundant NMDAR regulatory subunits in the mammalian brain. GluN2A-containing NMDARs are abundant in the postsynaptic membrane of the hippocampus neurons (Monyer et al., Citation1994; Paoletti et al., Citation2013). The percentage of the area stained by GluN2A antibody was less in hippocampal sections from knockout mice (). CUMS exposure induced further decrease of positive GluN2A stained area in knockout mice. But CUMS exposure had no effect on GluN2A stained area in control mice. Western blot analysis of this protein in hippocampal tissues is shown in . The effect of genotype (F (1, 5) = 11.98, p = .02) was significant while the effect of CUMS treatment (F (1, 5) = 4.35, p = .09) was not significant. There was interaction between genotype and CUMS treatment (F (1, 5) = 15.52, p = .01). CUMS exposure led to a decrease on GluN2A expression in bc004004−/− mice compared with the control mice.
3.6. Lack of BRAP homologous protein influenced BDNF and TrkB expressions of the hippocampus in CUMS model
BDNF and its high affinity receptor (Trk-B) are widely expressed in mammalian brain. BDNF-TrkB signaling plays significant roles in various stages of synaptic development and is important for the regulation of synaptic plasticity (Niculescu et al., Citation2018).
To explore whether BDNF-TrkB signaling is involved in the alterations of synaptic plasticity in bc004004−/− mice, we measured the expression of Trk-B and BDNF in the hippocampus by immunofluorescence assay and western blotting. BDNF positively stained neurons were detected by immunofluorescence assay in hippocampal sections using an anti-BDNF antibody as the primary antibody and Invitrogen Alexor Fluor Plus 647 goat anti-rabbit fluorescent antibody as the secondary antibody (). After CUMS treatment, the percentage of BDNF positively stained area decreased in bc004004−/− mice compared with bc004004+/+ mice ().
Figure 6. The expression of some proteins involved in synapse plasticity of the hippocampus.
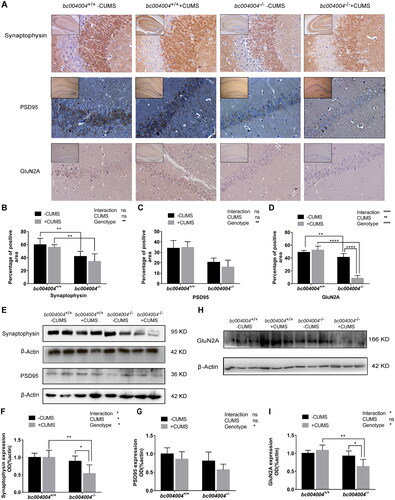
Figure 7. The impact of CUMS treatment on the expression of BDNF and TrkB in the hippocampal region of mice.
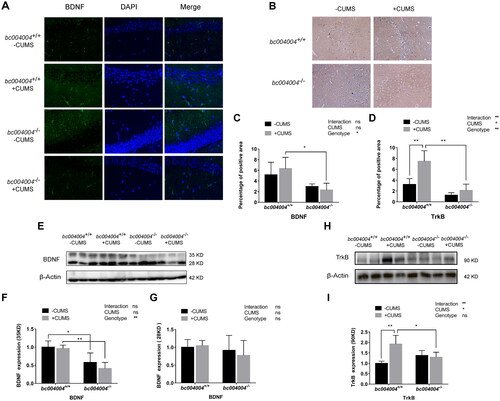
The precursor of BDNF has several isoforms and can be glycosylated. The 28 kDa precursor undergoes N-terminal cleavage within the trans-Golgi network and/or immature secretory vesicles to generate mature 14 kDa BDNF. We did not observe the mature form which is expected at 14 kDa (monomer) from the protein extract of hippocampus tissue by western blot analysis. The BDNF antibody detected two bands at about 28 kDa and 35 kDa (). The 35 kDa band could be a possible immature (unprocessed) precursor or glycosylated protein. The effect of genotype (F (1, 4) = 25.45, p = .01) was significant while the effect of CUMS treatment (F (1, 4) = 5.00, p = .09) was not significant. There was no interaction between genotype and CUMS treatment (F (1, 4)=1.39, p = .30). The expression of the 35 kDa band decreased in mice lacking BRAP homologous protein no matter CUMS exposure was applied or not, compared with their isogenic control mice (). However, there was no significant difference in the expression level for the band of 28 kDa among different groups (; main effect of genotype: F (1, 4) = 0.98, p = .38; main effect of CUMS: F (1, 4) = 0.29, p = .62; no interaction between genotype and CUMS: F (1, 4) = 0.75, p = .44).
The immunohistochemistry analysis of Trk-B, the receptor of BDNF, in hippocampal sections was also performed. As shown in , 21-day of CUMS increased Trk-B expression in bc004004+/+ compared with bc004004−/−.
Western blot analysis using the antibody against Trk-B detected a band at around 90 kDa in the protein extracts of hippocampal tissues as shown in Figure H. The effect of CUMS treatment (F (1, 5) = 10.73, p = .02) was significant while the effect of genotype (F (1, 5) = 0.94, p = .38) was not significant. There was interaction between genotype and CUMS treatment (F (1, 5) = 32.75, p = .002). CUMS treatment led to an increase of the expression of TrK-B in bc004004+/+ whereas CUMS exposure had no significant effect on the expression of Trk-B in bc004004−/− mice (). Those data indicate that the signaling of BDNF-TrkB pathway might be inhibited after CUMS treatment in bc004004−/− mice compared with bc004004+/+ mice. The altered expression pattern of BDNF and Trk-B might contribute to the alterations of synaptic plasticity in mice lacking BRAP homologous protein.
4. Discussion
Both human BRAP and its homologous protein in mice are widely expressed in many tissues with unknown functions. In the current study the expression of BRAP in human brain tissues and the presence of BRAP homologous protein in mouse brain were validated. In addition to the evidence of BRAP expression in the brain, another line of evidence that supports a biological role of BRAP in the brain was found. Immunostaining with an anti-BRS-3 antibody revealed that the expression of BRS-3 was down-regulated in the hippocampus proper of bc004004−/− mice compared with that of bc004004+/+ mice (Supplementary Figure 1). This finding suggests a link between the function of BRAP and BRS-3 in the brain. And it also provides evidence that BRAP homologous protein plays a biological role in the brain since BRS-3 was believed to have function in the brain (Jensen et al., Citation2008). However, the co-immunoprecipitation experiment failed to validate the physical interaction between BRAP and BRS-3 in a cultured human bronchial epithelial cell line 16HBE14o- in which both of them were abundantly expressed (data not presented).
We used several behavioral tests to assess the impact of BRAP homologous protein deficiency on mice behaviors. However, there were only eight animals in each group for those tests. The relatively small sample size is a limitation of this study.
In this study, mice lacking BRAP exhibited loss of memory and recognition after CUMS exposure as revealed by NOR task, indicating that chronic stress might cause damage to hippocampus function. The underlying mechanism might be the decrease of dendritic length and altered dendritic morphology caused by BRAP deficiency. In the absence of CUMS treatment, BRAP deficiency led to the changes of neurons in hippocampus of bc004004−/− mice. Those changes were characterized as shorter dendritic length, decreased amount of mature forms and increased amount of immature spines compared with control mice. There could be a ceiling effect regarding the decrease of dendritic length in CA1 of bc004004−/− mice. In those mice the decrease of dendritic length caused by lack of BRAP reached the upper limit. Thus CUMS exposure could not make further decrease on the dendritic length in those mice and no significant difference of dendritic length between different genotypes was observed after CUMS exposure. In addition to dendritic length, the genotype also affected the shapes and sizes of dendritic spines in hippocampus. BRAP deficiency increased the number of “thin” immature spines in CA3 and decreased the number of “stubby” mature form of spines in CA1. CUMS treatment caused further decrease of the number of “stubby” spines in bc004004−/− in CA1. The alterations of dendrites morphology in hippocampus may contribute to impairment of memory and recognition and thus may account partially for behavioral changes in bc004004−/− mice. The changes of dendrite morphology in hippocampus were also collaborated with the aberrant expression levels of some proteins involved in synapse formation or function in hippocampal tissues from the knockout mice. Among those proteins, BDNF is quite important since it plays a critical role in the maintenance of neurons and the regulation of synaptogenesis in key neural networks involved in cognitive function. Modulation of BDNF signaling was found to be altered in animal models of stress – induced disorders (Phillips, Citation2017). In the current study, after CUMS treatment, the BDNF expression was decreased in bc004004−/− mice and Trk-B expression was increased in bc004004+/+ compared with bc004004−/−. Those changes suggest an altered BDNF signaling in the gene knockout mice. This may contribute to the alterations of synaptic plasticity in mice lacking BRAP homologous protein. In addition to BDNF signaling pathway, synaptophysin and GluN2A are also important in signal transduction in the neuronal network of hippocampus. BRAP deficiency caused decreased expression of both synaptophysin and GluN2A in hippocampus. CUMS caused a further decrease in the expression levels of those proteins in bc004004−/− mice. Those data regarding the above alterations of proteins important for neural network and connectivity suggest a possible mechanism underlying the role of BRAP in regulating neuroplasticity in the brain.
In a recent published paper we reported that BRAP inhibited autophagosomes formation by interacting with ATG5, a key factor in autophagic process (Wang et al., Citation2022). And BRAP deficiency caused increased autophagy activity in isolated primary lung fibroblasts of mice. Autophagy is the process through which cytoplasmic materials are degraded in the lysosomes. The altered autophagy process was shown to be involved in many CNS disorders (Griffey & Yamamoto, Citation2022). Further investigations need to be done to elucidate whether BRAP deficiency causes enhanced autophagy in neurons and whether the role of BRAP in autophagy is relevant in regulating neural plasticity. Taken together, the present study thereby lays groundwork for future research into identification of the exact role of BRAP played in the nervous system. The evidence from this study also suggests that defects or mutations in BRAP could be a potential genetic marker in screening of stress-related disorders. And the modulation of BRAP expression in brain could be a novel target for the treatment of those disorders.
Author contributions
X. Yao performed and analyzed most of the experiments; H. Wang, J. Zheng, J. Wang and Z. Peng performed immunostaining of BRAP in brain tissues, and the co-immunoprecipitation; X. Yao, H.Wang, J. Zheng, R. Liu, W. Zhang, J.Zeng, S. Zuo and H. Chen maintained the animals; X. Qu designed the research and wrote the manuscript; X. Qin and H. C. Weber reviewed and commented on the manuscript; H. C. Weber corrected the language.
Supplemental Material
Download Zip (3.7 MB)Disclosure statement
No potential conflict of interest was reported by the author(s).
Additional information
Funding
References
- Arauchi, R., Hashioka, S., Tsuchie, K., Miyaoka, T., Tsumori, T., Limoa, E., Azis, I. A., Oh-Nishi, A., Miura, S., Otsuki, K., Kanayama, M., Izuhara, M., Nagahama, M., Kawano, K., Araki, T., Liaury, K., Abdullah, R. A., Wake, R., Hayashida, M., Inoue, K., & Horiguchi, J. (2018). Gunn rats with glial activation in the hippocampus show prolonged immobility time in the forced swimming test and tail suspension test. Brain and Behavior, 8(8), e01028. https://doi.org/10.1002/brb3.1028
- Baxter, L. R., Jr., Schwartz, J. M., Phelps, M. E., Mazziotta, J. C., Guze, B. H., Selin, C. E., Gerner, R. H., & Sumida, R. M. (1989). Reduction of prefrontal cortex glucose metabolism common to three types of depression. Archives of General Psychiatry, 46(3), 243–250. https://doi.org/10.1001/archpsyc.1989.01810030049007
- Biala, G., Pekala, K., Boguszewska-Czubara, A., Michalak, A., Kruk-Slomka, M., & Budzynska, B. (2017). Behavioral and biochemical interaction between nicotine and chronic unpredictable mild stress in mice. Molecular Neurobiology, 54(2), 904–921. https://doi.org/10.1007/s12035-016-9701-0
- Chen, X., Nelson, C. D., Li, X., Winters, C. A., Azzam, R., Sousa, A. A., Leapman, R. D., Gainer, H., Sheng, M., & Reese, T. S. (2011). PSD-95 is required to sustain the molecular organization of the postsynaptic density. The Journal of Neuroscience: The Official Journal of the Society for Neuroscience, 31(17), 6329–6338. https://doi.org/10.1523/JNEUROSCI.5968-10.2011
- Cryan, J. F., Mombereau, C., & Vassout, A. (2005). The tail suspension test as a model for assessing antidepressant activity: Review of pharmacological and genetic studies in mice. Neuroscience and Biobehavioral Reviews, 29(4-5), 571–625. https://doi.org/10.1016/j.neubiorev.2005.03.009
- Duman, R. S., Aghajanian, G. K., Sanacora, G., & Krystal, J. H. (2016). Synaptic plasticity and depression: New insights from stress and rapid-acting antidepressants. Nature Medicine, 22(3), 238–249. https://doi.org/10.1038/nm.4050
- Griffey, C. J., & Yamamoto, A. (2022). Macroautophagy in CNS health and disease. Nature Reviews. Neuroscience, 23(7), 411–427. https://doi.org/10.1038/s41583-022-00588-3
- Harvey, P. O., Fossati, P., Pochon, J. B., Levy, R., Lebastard, G., Lehéricy, S., Allilaire, J. F., & Dubois, B. (2005). Cognitive control and brain resources in major depression: An fMRI study using the n-back task. NeuroImage, 26(3), 860–869. https://doi.org/10.1016/j.neuroimage.2005.02.048
- Horii, Y., McTaggart, I., & Kawaguchi, M. (2018). Testing animal anxiety in rats: Effects of open arm ledges and closed arm wall transparency in elevated plus maze test. Journal of Visualized Experiments, 136, 56428. https://doi.org/10.3791/56428
- Jensen, R. T., Battey, J. F., Spindel, E. R., & Benya, R. V. (2008). International Union of Pharmacology. LXVIII. Mammalian bombesin receptors: Nomenclature, distribution, pharmacology, signaling, and functions in normal and disease states. Pharmacological Reviews, 60(1), 1–42. https://doi.org/10.1124/pr.107.07108
- Kraeuter, A. K., Guest, P. C., & Sarnyai, Z. (2019). The open field test for measuring locomotor activity and anxiety-like behavior. Methods in Molecular Biology, 1916, 99–103. https://doi.org/10.1007/978-1-4939-8994-2_9
- Lapter, S., Marom, A., Meshorer, A., Elmann, A., Sharabi, A., Vadai, E., Neufeld, A., Sztainberg, Y., Gil, S., Getselter, D., Chen, A., & Mozes, E. (2009). Amelioration of brain pathology and behavioral dysfunction in mice with lupus following treatment with a tolerogenic peptide. Arthritis and Rheumatism, 60(12), 3744–3754. https://doi.org/10.1002/art.25013
- Lisman, J. E., & Grace, A. A. (2005). The hippocampal-VTA loop: Controlling the entry of information into long-term memory. Neuron, 46(5), 703–713. https://doi.org/10.1016/j.neuron.2005.05.002
- Liu, B., Liu, J., Wang, M., Zhang, Y., & Li, L. (2017). From serotonin to neuroplasticity: Evolvement of theories for major depressive disorder. Frontiers in Cellular Neuroscience, 11, 305. https://doi.org/10.3389/fncel.2017.00305
- Liu, H. J., Tan, Y. R., Li, M. L., Liu, C., Xiang, Y., & Qin, X. Q. (2011). Cloning of a novel protein interacting with BRS-3 and its effects in wound repair of bronchial epithelial cells. PLoS One, 6(8), e23072. https://doi.org/10.1371/journal.pone.0023072
- Liu, Y., Qin, X. Q., Weber, H. C., Xiang, Y., Liu, C., Liu, H. J., Yang, H., Jiang, J., & Qu, X. (2016). Bombesin receptor-activated protein (BRAP) modulates NF-κB activation in bronchial epithelial cells by enhancing HDAC activity. Journal of Cellular Biochemistry, 117(5), 1069–1077. https://doi.org/10.1002/jcb.25406
- MacQueen, G. M., Campbell, S., McEwen, B. S., Macdonald, K., Amano, S., Joffe, R. T., Nahmias, C., & Young, L. T. (2003). Course of illness, hippocampal function, and hippocampal volume in major depression. Proceedings of the National Academy of Sciences of the United States of America, 100(3), 1387–1392. https://doi.org/10.1073/pnas.0337481100
- Monyer, H., Burnashev, N., Laurie, D. J., Sakmann, B., & Seeburg, P. H. (1994). Developmental and regional expression in the rat brain and functional properties of four NMDA receptors. Neuron, 12(3), 529–540. https://doi.org/10.1016/0896-6273(94)90210-0
- Niculescu, D., Michaelsen-Preusse, K., Güner, Ü., van Dorland, R., Wierenga, C. J., & Lohmann, C. (2018). A BDNF-mediated push-pull plasticity mechanism for synaptic clustering. Cell Reports, 24(8), 2063–2074. https://doi.org/10.1016/j.celrep.2018.07.073
- Nollet, M., Le Guisquet, A. M., & Belzung, C. (2013). Models of depression: Unpredictable chronic mild stress in mice (Chapter 5, Unit 5.65). Current Protocols in Pharmacology. https://doi.org/10.1002/0471141755.ph0565s61
- Pan, X., Jiang, T., Zhang, L., Zheng, H., Luo, J., & Hu, X. (2017). Physical exercise promotes novel object recognition memory in spontaneously hypertensive rats after ischemic stroke by promoting neural plasticity in the entorhinal cortex. Frontiers in Behavioral Neuroscience, 11, 185. https://doi.org/10.3389/fnbeh.2017.00185
- Pandya, C. D., Hoda, N., Crider, A., Peter, D., Kutiyanawalla, A., Kumar, S., Ahmed, A. O., Turecki, G., Hernandez, C. M., Terry, A. V., & Pillai, A. (2017). Transglutaminase 2 overexpression induces depressive-like behavior and impaired TrkB signaling in mice. Molecular Psychiatry, 22(5), 745–753. https://doi.org/10.1038/mp.2016.145
- Paoletti, P., Bellone, C., & Zhou, Q. (2013). NMDA receptor subunit diversity: Impact on receptor properties, synaptic plasticity and disease. Nature Reviews. Neuroscience, 14(6), 383–400. https://doi.org/10.1038/nrn3504
- Phillips, C. (2017). Brain-derived neurotrophic factor, depression, and physical activity: Making the neuroplastic connection. Neural Plasticity, 2017, 7260130. https://doi.org/10.1155/2017/7260130
- Pittenger, C., & Duman, R. S. (2008). Stress, depression, and neuroplasticity: A convergence of mechanisms. Neuropsychopharmacology: Official Publication of the American College of Neuropsychopharmacology, 33(1), 88–109. https://doi.org/10.1038/sj.npp.1301574
- Qu, X., Li, M., Liu, H. J., Xiang, Y., Tan, Y., Weber, H. C., & Qin, X. Q. (2013). Role of bombesin receptor activated protein in the antigen presentation by human bronchial epithelial cells. Journal of Cellular Biochemistry, 114(1), 238–244. https://doi.org/10.1002/jcb.24366
- Racagni, G., & Popoli, M. (2008). Cellular and molecular mechanisms in the long-term action of antidepressants. Dialogues in Clinical Neuroscience, 10(4), 385–400. https://doi.org/10.31887/DCNS.2008.10.4/gracagni
- Raja, M. K., Preobraschenski, J., Del Olmo-Cabrera, S., Martinez-Turrillas, R., Jahn, R., Perez-Otano, I., & Wesseling, J. F. (2019). Elevated synaptic vesicle release probability in synaptophysin/gyrin family quadruple knockouts. eLife, 8, e40744. https://doi.org/10.7554/eLife.40744
- Sapolsky, R. M. (2000). Glucocorticoids and hippocampal atrophy in neuropsychiatric disorders. Archives of General Psychiatry, 57(10), 925–935. https://doi.org/10.1001/archpsyc.57.10.925
- Sapolsky, R. M. (2003). Stress and plasticity in the limbic system. Neurochemical Research, 28(11), 1735–1742. https://doi.org/10.1023/A:1026021307833
- Seibenhener, M. L., & Wooten, M. C. (2015). Use of the open field maze to measure locomotor and anxiety-like behavior in mice. Journal of Visualized Experiments, 96, e52434. https://doi.org/10.3791/52434
- Sholl, D. A. (1953). Dendritic organization in the neurons of the visual and motor cortices of the cat. Journal of Anatomy, 87(4), 387–406.
- Squire, L. R., Stark, C. E., & Clark, R. E. (2004). The medial temporal lobe. Annual Review of Neuroscience, 27, 279–306. https://doi.org/10.1146/annurev.neuro.27.070203.144130
- Stockmeier, C. A., Mahajan, G. J., Konick, L. C., Overholser, J. C., Jurjus, G. J., Meltzer, H. Y., Uylings, H. B., Friedman, L., & Rajkowska, G. (2004). Cellular changes in the postmortem hippocampus in major depression. Biological Psychiatry, 56(9), 640–650. https://doi.org/10.1016/j.biopsych.2004.08.022
- Tian, Z., Dong, C., Zhang, K., Chang, L., & Hashimoto, K. (2018). Lack of antidepressant effects of low-voltage-sensitive T-type calcium channel blocker ethosuximide in a chronic social defeat stress model: Comparison with (R)-ketamine. The International Journal of Neuropsychopharmacology, 21(11), 1031–1036. https://doi.org/10.1093/ijnp/pyy072
- Walf, A. A., & Frye, C. A. (2007). The use of the elevated plus maze as an assay of anxiety-related behavior in rodents. Nature Protocols, 2(2), 322–328. https://doi.org/10.1038/nprot.2007.44
- Wang, H., Zhang, W., Liu, R., Zheng, J., Yao, X., Chen, H., Wang, J., Weber, H. C., Qin, X., Xiang, Y., Liu, C., Liu, H., Pan, L., & Qu, X. (2022). Lack of bombesin receptor-activated protein attenuates bleomycin-induced pulmonary fibrosis in mice. Life Science Alliance, 5(11), e202201368. https://doi.org/10.26508/lsa.202201368
- Warner-Schmidt, J. L., & Duman, R. S. (2006). Hippocampal neurogenesis: Opposing effects of stress and antidepressant treatment. Hippocampus, 16(3), 239–249. https://doi.org/10.1002/hipo.20156
- Wei, J., Carroll, R. J., Harden, K. K., & Wu, G. (2012). Comparisons of treatment means when factors do not interact in two-factorial studies. Amino Acids, 42(5), 2031–2035. https://doi.org/10.1007/s00726-011-0924-0