Abstract
Stress is a normal response to situational pressures or demands. Exposure to stress activates the hypothalamic-pituitary-adrenal (HPA) axis and leads to the release of corticosteroids, which act in the brain via two distinct receptors: mineralocorticoid receptors (MR) and glucocorticoid receptors (GR). Persistent HPA axis overactivation or dysregulation can disrupt an individual’s homeostasis, thereby contributing to an increased risk for mental illness. On the other hand, successful coping with stressful events involves adaptive and cognitive processes in the brain that render individuals more resilient to similar stressors in the future. Here we review the role of the MR in these processes, starting with an overview of the physiological structure, ligand binding, and expression of MR, and further summarizing its role in the brain, its relevance to psychiatric disorders, and related rodent studies. Given the central role of MR in cognitive and emotional functioning, and its importance as a target for promoting resilience, future research should investigate how MR modulation can be used to alleviate disturbances in emotion and behavior, as well as cognitive impairment, in patients with stress-related psychiatric disorders.
1. Introduction
Mental health is of utmost importance for individual wellbeing and success in life. According to the Global Burden of Diseases, Injuries, and Risk Factors Study, nearly 1 billion people are living with mental disorders, with anxiety and depressive disorders having the highest prevalence. Mental disorders are one of the top ten health challenges faced by people worldwide (GBD 2019 Mental Disorders Collaborators, Citation2022). There are many proposed causes of mental illness, one of which is currently recognized as the malfunction of the hypothalamic-pituitary-adrenal (HPA) axis due to severe and unpredictable stress exposure. The HPA axis is an essential part of the neuroendocrine system and is involved in the control of stress responses that stimulate the production and release of adrenal cortisol. When the body encounters a stressor (both physiological and psychological), the paraventricular nucleus of the hypothalamus synthesizes and secretes corticotropin-releasing hormone (CRH), which in turn acts on the anterior pituitary gland to stimulate the release of adrenocorticotropic hormone (ACTH). ACTH promotes the synthesis and secretion of glucocorticoid (GC) hormones by binding to the melanocortin-2 receptor (MC2R) in the adrenal cortex (Fink, Citation2016; Gupta, Citation2019; Rao & Androulakis, Citation2019). Cortisol is the primary glucocorticoid in humans, whereas corticosterone (CORT) predominates in rodents. Glucocorticoid genomic signaling involves the binding of glucocorticoids to two nuclear receptors: type I is the mineralocorticoid receptor (MR), and type II is the glucocorticoid receptor (GR) (Antoni, Citation2019; Rao & Androulakis, Citation2019; Reul & de Kloet, Citation1985; Yiallouris et al., Citation2019).
There has been a lot of research on GR and stress-related psychiatric disorders (De Nicola et al., Citation2020; Finsterwald & Alberini, Citation2014; Spijker & van Rossum, Citation2009), but the contribution of MRs has not received as much attention. However, in recent years, studies have confirmed that by conveying hormone signals and stimulating the expression of target genes, MRs regulate a number of physiological and pathological responses relevant to brain function and disease. MRs have a vital role in cognition, emotion, and behavior, in addition to regulating water and salt balance (Chen et al., Citation2016; Paul et al., Citation2022; Wingenfeld & Otte, Citation2019). This article not only reviews the structure, distribution, and ligand selectivity of MR, but also focuses on the function of MR in the brain and its relationship to various psychiatric disorders. We will showcase that the context-specific timing of MR involvement as well as the affected brain region or cell type influence the function of this receptor.
2. MR structure
2.1. Gene structure
The human MR (hMR) NR3C2 gene is located at q31.1-q31.2 region of chromosome 4, consists of 450 bases, and encodes a 984 amino acid protein of 107 KDa (Arriza et al., Citation1987; Fan et al., Citation1989; Morrison et al., Citation1990). There are 10 exons in the hMR gene; the first 2 exons (1α and 1β) are not translated, and the remaining 8 exons encode the entire MR protein, thereby resulting in the generation of two possible transcripts, hMRα and hMRβ (Zennaro et al., Citation1995). Different from humans, the MR gene in rats is located on chromosome 19q11, has 3 MR splice variants: αMR, βMR, and γMR, and encodes 981 amino acids (Kwak et al., Citation1993; Viengchareun et al., Citation2007). Further, some studies have found the splice variant MR + 4 of MR in various human and rat tissues (Wickert et al., Citation1998). In mice, the MR gene has a similar structure of that in rats and encodes 978 amino acids (Viengchareun et al., Citation2007). The MR gene is evolutionarily conserved in most mammals (Rivers et al., Citation2009). Patel et al. (Citation1989) found that in the coding region and the 3′ untranslated region, there is an 88% open reading frame nucleic acid homology between rat and human kidney MR.
2.2. Protein structure
As a member of the nuclear receptor superfamily, MR shares a common protein structure with GR (Koning et al., Citation2019), which is composed of three independent domains. These three main functional domains are the N-terminal domain (NTD), the DNA binding domain (DBD), and the C-terminal ligand-binding domain (LBD) (Couette et al., Citation1996). The NTD is located at the NH2-terminal and plays a key role in regulating the specificity of MR action. It is highly conserved, with at least 50% homology between different species (Pascual-Le Tallec & Lombès, Citation2005; Viengchareun et al., Citation2007). MR DBD, which located in the middle of the protein, exhibits a considerable degree of similarity (roughly 94%) to GR DBD, and has a role in binding specific target DNA sequences and hormone response elements (HRE) (Hudson et al., Citation2014; Viengchareun et al., Citation2007). Finally, the LBD is located at the COOH-terminal and is responsible for binding to specific hormone ligands, and mediating the translocation of MR from the cytoplasm to the nucleus, and its homology between species is as high as 80%-97% (K. Fischer et al., Citation2010; Fuller et al., Citation2012; Grossmann et al., Citation2022; Mangelsdorf et al., Citation1995; Sheppard, Citation2002; Viengchareun et al., Citation2007; Zennaro et al., Citation1995).
3. MR ligands
Steroid hormone receptors are ligand-activated and switch from an inactive state to an active state by binding to their corresponding hormones (Torchia et al., Citation1998). Since MR LBD shares high homology with GR LBD, MR has two main endogenous ligands: aldosterone and cortisol in humans, or CORT in rodents (Baker et al., Citation2013). MR has a high affinity for cortisol (Kd = 0.5 nM), 10-fold higher than GR (Kd = 5 nM) (Meijer et al., Citation2018). The circulating concentration of cortisol in the blood is about 100–1000 times that of aldosterone (Syed & Qureshi, Citation2012). Even if only 5%-10% of cortisol is actively free, cortisol levels remain much higher than aldosterone in plasma (Cizza & Rother, Citation2012; Mifsud & Reul, Citation2018). Consequently, MR will be entirely occupied by cortisol except for when the circadian cycle of cortisol release is at its lowest point. Aldosterone has the same affinity for binding MR as cortisol, and since aldosterone dissociates from MR more slowly than cortisol, the aldosterone-MR complex is more stable and potent.
As a member of the nuclear receptor superfamily, MR is essential for the control of sodium and potassium transport in epithelial cells, especially in the kidney and colon (Fuller & Young, Citation2005; Viengchareun et al., Citation2007). MR is also widely distributed in non-epithelial tissues both humans and animals. For example, MR is expressed not only in cardiac myocytes, but also in endothelial and smooth muscle cells of the vascular system (Christy et al., Citation2003). In the central nervous system, the hippocampus has the highest MR abundance. While the dominant ligands of brain MR are cortisol/CORT, MR binds to aldosterone in selective brain regions, i.e. in the brainstem, to regulate the physiology and behavior associated with salt balance (Fuller & Verity, Citation1990). In the reproductive system, MR is expressed in the granulosa cells of the ovary (Fru et al., Citation2006). Progesterone, androgens and their derivatives can also bind to MR and exhibit partial activation or antagonism (Quinkler et al., Citation2004). Therefore, progesterone is considered by some as another physiological ligand for MR. In humans and rodents, progesterone antagonizes MR activation via aldosterone, and in sharks and chickens, progesterone activates MR directly (Baker & Katsu, Citation2020). It appears that MR acts as a "type I corticosteroid receptor" in these species. The existing mineralocorticoid antagonists, such as spironolactone and eplerenone, have been used clinically as antihypertensive and cardiovascular protection drugs (Lainscak et al., Citation2015). Drospirenone as a new progestin also has certain MR antagonist activity (Motivala & Pitt, Citation2007). Taken together, this shows that MR ligands are diverse (see ) (Fuller et al., Citation2012; Paul et al., Citation2022; Reul et al., Citation2000) and additional mechanisms are required to regulate their selectivity.
Table 1. The ligands of mineralocorticoid receptor.
4. Cellular mechanisms of MR
In the absence of ligands, MR mostly remains in the cytoplasm, and is inactivated by binding to heat shock protein 90 (hsp90) and other chaperone proteins (Lombès et al., Citation1994; Robertson et al., Citation1993). When the ligand enters the cell and binds to the MR, specific activation domains control the activity of MR functions. One is an autonomous activation function 1 (AF-1) located at the N-terminus, and the other is transcriptional activation function 2 (AF-2), which is located at the C-terminus (Yokota et al., Citation2007). The ligand-receptor complex undergoes a conformational change, dissociating from hsp90, and the MR is converted into a DBD-active form, thereby initiating a nuclear translocation signal (Weikum et al., Citation2018; Yang & Young, Citation2009). After MR enters the nucleus, it binds to the HRE on the target gene via DBD, and mediates transcriptional activation or repression under the combined action of transcriptional co-regulators (see ) (Fuller & Young, Citation2005). When aldosterone engages with MR, the interaction between N-terminus and C-terminus is enhanced and the internal structure of MR changes, resulting in the recruitment of particular synergistic transcription factors and the promotion of the matching aldosterone effects. This interaction between NTD and LBD is aldosterone-dependent, as cortisol and MR have no such impact when combined (Fuller, Citation2015).
Figure 1. Schematic representation of intracellular genomic and non-genomic signaling pathways following MR ligand binding. Rapid MR signaling via non-genomic pathways activates downstream receptor kinases and second messenger systems, ultimately leading to alterations in neuronal activity. Conversely, for the genomic pathway, ligand-activated MR dissociates from its chaperone protein complex, translocates to the nucleus and regulates gene transcription of HRE-containing target cells. In 11ß-HSD2 containing cells the main natural ligand of MR is aldosterone, as the high-affinity ligand cortisol (or corticosterone) will be converted. In cells not containing 11ß-HSD2, as for example many neurons, the main ligand for MR is cortisol or corticosterone. MR: mineralocorticoid receptor; HRE: hormone response element; RAS: renin-angiotensin system; ERK: extracellular signal-regulated kinase; PI3K: Phosphatidylinositide 3-kinases; Akt: protein kinase B; PKC: protein kinases C; PKD: protein kinases D. (Illustration is created with Biorender.com).
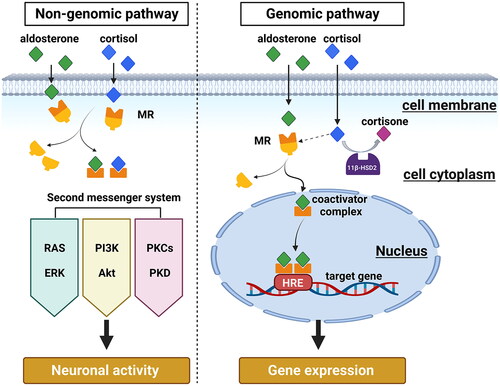
In addition, some researchers have proposed a fast, non-genomic action of membrane-associated MR, distinct from the classical intracellular function of MR as transcription factor (Groeneweg et al., Citation2012; Wehling, Citation2005). This non-genomic effect manifests rapidly, and also disappears quickly, indicating that its action is exerted at the level of the cell membrane (Keller-Wood & Dallman, Citation1984). For instance, within the first hour of administration, cortisol exerts numerous effects on cognition and mood, and these early effects can only be explained by early non-genomic effects, also highlighting the significance of MR in the initial stages of the stress response (Joëls et al., Citation2012).
Although it has been found that other steroids, such as estrogen, may exert rapid non-genomic effects by binding to signal transduction protein-associated membrane receptors (Arnal et al., Citation2017; Schumacher, Citation1990), MR, unlike other steroid receptors, lacks palmitoyl ionization sites and cannot be inserted into the membrane (Nicolaides et al., Citation2017). Nevertheless, some researchers suggested that MR can communicate with the cell membrane through the scaffold proteins striatin and caveolin-1 (CAV1) (Ashton et al., Citation2015; Coutinho et al., Citation2014). Other studies have found that MR exploits many intracellular signaling cascades to more rapidly alter cellular function through transactivation of receptor kinases. Examples include epidermal growth factor receptor (EGFR), platelet-derived growth factor receptor (PDGFR), and insulin-like growth factor1 receptor (IGF1R), as well as some second messenger systems like extracellular signal-regulated kinase (ERK) cascade, phosphoinositide-3-kinase–protein kinase B/Akt (PI3K-PKB/Akt) pathway, and protein kinases C (PKC) and D (PKD) (see ) (Nagata et al., Citation2019; Ong & Young, Citation2017; Parker et al., Citation2018; Ruhs et al., Citation2017). MRs that mediate non-genomic effects are thought to be identical to MRs involved in genomic signaling, with the exception that they may be translocated to the membrane rather than the nucleus, and rapid effects of aldosterone can be induced by aldosterone-BSA in cells without nuclear MRs (Karst et al., Citation2022). Additional evidence for MR-mediated rapid and non-genomic effects were reported for MR ligands fused to BSA, thereby prohibiting the intracellular diffusion of the ligands. For example, a study using Aldosterone-BSA found that this extracellular MR ligand activates the PKCα pathway, induces MR phosphorylation, and triggers cross-talk between the nongenomic and genomic responses in renal collecting duct cells (Le Moëllic et al., Citation2004). While there is substantial evidence of rapid MR-mediated effects in peripheral tissues (Hermidorff et al., Citation2017), the situation in the brain and specifically in 11β-hydroxysteroid dehydrogenases (11β-HSD) type 2 (11β-HSD2) negative cells is still largely unclear. Visual proof of MR located and acting at the membrane level is difficult, therefore the membrane receptor hypothesis so far lacks direct evidence. Integrating the nongenomic and genomic activities of MR is an intriguing topic that needs more research.
5. MR selectivity
As mentioned above, many substances have similar affinities to MR, and MR signals can be modulated at various levels. The following will describe how MR selects the corresponding ligand and produces a specific response.
5.1. Selectivity at the pre-receptor level
The tissue distribution, expression, and coordination aspects of MR influence the mineralocorticoid actions it mediates. Specifically, the modulation of local MR ligand concentrations through specific enzymes is modulating MR function at the pre-receptor level (Penning et al., Citation2008). Important enzymes in this regard are the 11β-HSD type 1 (11β-HSD1) and 11β-HSD2 which regulate intracellular glucocorticoid levels. In humans, cortisol is converted to cortisone by 11β-HSD2, which is a derivative with very low affinity for MR and cannot bind or activate MR, hence allowing tissue-specific MR regulation (Funder, Citation1991; Odermatt & Atanasov, Citation2009). Similarly in rodents, corticosterone is converted to 11-deoxycorticosterone, and neither product of the conversion can bind to or activate MR (Odermatt & Atanasov, Citation2009). Aldosterone can activate MR in aldosterone target cells due to 11β-HSD2’s inactivation of cortisol and corticosterone (Gomez-Sanchez & Gomez-Sanchez, Citation2014). Therefore, the entry of aldosterone and the absence of cortisol action on MR are governed by the activity of 11β-HSD2 (Edwards et al., Citation1988; Funder et al., Citation1988). However, since 11β-HSD2 is not expressed in many non-epithelial tissues, such as heart, nervous system or adipocytes, MR selectivity may be controlled by mechanisms at the post-receptor level (Faresse, Citation2014; Gomez-Sanchez & Gomez-Sanchez, Citation2014; Penning, Citation2003). Further, there are few neurons in the brain that can co-express 11β-HSD2 and MR, and are aldosterone-sensitive. This kind of neurons have been found to be located mainly in the nucleus tractus solitarius (NTS) and to be efferent to the nucleus accumbens (NAc) via a multisynaptic pathway relayed from the dorsolateral pons and paraventricular thalamic nucleus (Gasparini et al., Citation2019; Geerling et al., Citation2006; Shekhtman et al., Citation2007). However, 11β-HSD2 mRNA is certainly not sufficient to explain MR selectivity, which is further modulated at the post-receptor level.
5.2. Selectivity at the post-receptor level
MR requires the presence of transcriptional co-factors in order to bind to ligands and exert its physiological effects, and their binding is affected by multiple post-translational modifications (PTMs), including phosphorylation, glycosylation, ubiquitination, methylation, acetylation, sumoylation, oxidative stress, etc. (Gadasheva et al., Citation2021). Among the large number of transcriptional co-factors that have been found, their functions and cellular expression are in various forms—some of which play a role in transcriptional activation, while others affect transcriptional repression (McKenna et al., Citation1999; Torchia et al., Citation1998; Yang & Young, Citation2009). Coactivators and corepressors are involved in chromatin remodeling (Liu et al., Citation1999). As a transcriptional MR coactivators, both 11–19 lysine-rich leukemia (ELL) and FLICE-associated huge protein (FLASH), can interact with AF-1 (Yang & Young, Citation2009); PPAR-γ coactivator 1 (PGC-1), on the other hand, acts by interacting with AF-2 (Knutti et al., Citation2000), and Ubiquitin conjugating enzyme 9 (Ubc9) interacts with the NTD of MR and thus exerts physiological effects (Yokota et al., Citation2007). Another example is the protein tesmin, which can coactivate aldosterone- and deoxycorticosterone-induced MR transactivation, but not cortisol-induced MR-mediated transactivation (Fuller, Citation2015). There are proteins that function as coactivators for numerous transcription factors, but the function of p160 family members or steroid receptor coactivators (SRCs) appears to be restricted to the nuclear receptor family (Meijer et al., Citation2000). As a corepressor, the nuclear receptor corepressor (NCoR) and thyroid hormone receptor (SMRT) interact with LBD (Wang et al., Citation2004), and death domain-associated protein (DAXX) and protein inhibitor of activated STAT1 (PIAS1) interact with NTD Role (Yang & Young, Citation2009). Fas-associated factor 1 (FAF1) has the ability to suppress aldosterone-activated MR transactivation after interacting with the sumoylated MR, and aldosterone increases the activation of MR target genes when endogenous FAF1 in cells was silenced (Wang et al., Citation2019). Different ligands in conjunction with MR can recruit distinct transcription coordinating factors, and these transcription-coordinating factors decide distinct outcomes (Faresse, Citation2014; Gadasheva et al., Citation2021; Weber et al., Citation2008).
6. Expression and distribution of MR
MR is expressed in many tissues throughout the body. MR expression in the periphery is predominant in epithelial tissues such as distal parts of the nephron, liver, distal colon, airway, sweat glands, inner ear, etc. (Gorini et al., Citation2019). In epithelial tissues, where cortisol has a low affinity for MR, the conversion of cortisol to corticosterone can be accomplished by 11β-HSD2, thus ensuring that aldosterone, the main physiological ligand in epithelial tissues, can bind to MR to exert its proper physiological effects (Edwards et al., Citation1988). In addition, many non-epithelial tissues such as cardiovascular, skin, placenta, ovary and testis, adipose tissue and the brain have been identified as expressing MR (Cole & Young, Citation2017). In contrast to epithelial tissues, the expression of 11β-HSD2 is almost absent or relatively low in many of these tissues. Thus, glucocorticoids become the main ligands for MR in most non-epithelial tissues (Marzolla et al., Citation2014). Differences in the tissue distribution of MR and GR were also related to receptor selectivity, and MR and GR are expressed at varying amounts on various cell types. In the immune system, for instance, GR is substantially more expressed than MR. In the central nervous system, however, many brain areas express CORT-preferred MR, including the prefrontal cortex, hippocampus, lateral septal thalamic nucleus, hypothalamic nucleus, and medial and central amygdala (Reul & de Kloet, Citation1985). In the majority of cases MR is co-expressed with GR, for example in the hippocampus (Gomez-Sanchez, Citation2014; Sarabdjitsingh et al., Citation2012). A single-cell sequencing study in the human temporal lobe cortex showed that in most inhibitory neurons, the expression levels of MR and GR were not high, and only two kinds of GABA-ergic neurons show higher expression of MR than of GR. The opposite was found to be true in glutamatergic neurons, where GR is generally expressed at a higher level than MR (Koning et al., Citation2019). Although in the brain, MR is commonly regarded as expressed mainly in neurons, recent single-cell mRNA expression analyses reveal that it is also expressed in astrocytes (Viho et al., Citation2022) and microglia (Bast et al., Citation2018; Odermatt & Kratschmar, Citation2012). Little attention has been given to potential sex differences in MR expression and distribution so far, although an early study studies found that in the hippocampus, MR mRNA was significantly higher expressed in females than in males (Watzka et al., Citation2000). As differential phenotypes were also observed between male and female forebrain-specific MR knockout mice (Ter Horst et al., Citation2012), a more detailed study of MR-related sex differences is highly warranted.
7. MR in the brain
There are many studies on the relationship between MR and the renal and cardiovascular systems, which are summarized elsewhere (Nakamura et al., Citation2022; Ravid & Laffin, Citation2022; van der Heijden et al., Citation2022). However, MR does not only play a physiological role in the aforementioned organ systems, but also in the brain despite being only widely distributed in the brain’s limbic system. Below we discuss the main functions of MR in the brain (see ).
Figure 2. Overview of MR functions in the brain. In the brain, MR regulates the HPA axis activity and circadian rhythm, modulates neurogenesis, participates in neuroinflammation and affects mood, behavior, and cognition.
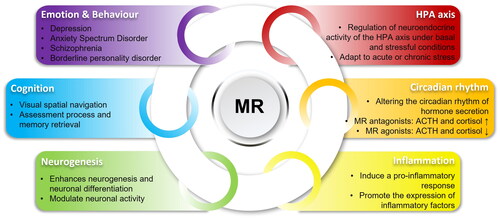
7.1. HPA axis and circadian rhythm
In the brain, MR is mainly expressed in neurons in the limbic system and prefrontal cortex (Kloet et al., Citation1998), both of which are known to regulate HPA axis activity. Here, MR is thought to function through γ-aminobutyric acid (GABA)-ergic neuron-mediated tonic inhibition projections to the medial parvicellular neurons of the PVN, which control ACTH secretion through CRH and AVP (Goncharova, Citation2020; Herman et al., Citation2016). It is well known that MR can modulate the neuroendocrine activity of the HPA axis, both under basal conditions and in stressful environments (Berardelli et al., Citation2013; Chen et al., Citation2016; Murck et al., Citation2014). Moreover, different MR variants have different effects on the activity of the basal HPA axis. MR (hydrochloride 2 C/G) G-carriers exhibit higher basal cortisol levels than C-carriers (Kuningas et al., Citation2007; Muhtz et al., Citation2011). For the MR GA haplotype, the inhibitory effect of dexamethasone on CAR was significantly higher in female carriers than in male carriers (van Leeuwen et al., Citation2010), highlighting the variant-specific and sex-specific functions of MR. Using forebrain-specific MR deletion in animal studies, it was found that forebrain MR may modulate the HPA axis by inhibiting the secretion of corticosterone. Both basal corticosterone secretion and stress-induced corticosterone secretion were altered in transgenic mice, and corticosterone levels were significantly increased under stress inhibition (Ter Horst et al., Citation2012). Under stress conditions, administering metyrapone to rats was discovered to enhance the binding of MR and GR to GRE present in the genes targeted by GC, and the transcriptional activity of these genes varied depending on the gene. But metyrapone did not completely result in the elimination of the effects of stress on plasma CORT, MR, and GR binding to HRE (Kennedy et al., Citation2020).
HPA axis activity follows a distinct circadian rhythm. CORT secretion is usually high at the beginning of the activity phase (e.g., morning in humans and evening in rodents) and declines to reach a trough at the start of the rest phase. GCs exhibit strong time dependence, with almost all physiological effects accompanied by similar rhythms (De Nobrega et al., Citation2020; Gaffey et al., Citation2016; Hood & Amir, Citation2017; Oster et al., Citation2017). MR plays a unique role in regulating the HPA axis, and it cooperates with GR to control the basal activity of the HPA axis. Administration of MR antagonists, whether in the morning or in the afternoon, increases ACTH and cortisol secretion during circadian quiescence, altering circadian-driven HPA activity (Berardelli et al., Citation2010; Oitzl et al., Citation1995; van Haarst et al., Citation1997). In addition, it has been shown that systemic treatment of the MR antagonist potassium canrenoate during the nadir phase of the circadian rhythm increases cortisol secretion (Grottoli et al., Citation2002). Following administration of the MR agonist fludrocortisone (0.5 mg), nocturnal HPA axis activity was significantly suppressed and cortisol and ACTH were sharply reduced (Buckley et al., Citation2007). However, limbic MR deficiency has no effect on basal circadian HPA axis activity in the cornu Ammonis 1 (CA1) region (Berger et al., Citation2006).
7.2. Neurogenesis
In adult mammals, new neurons continue to be generated in the granule cells of the hippocampal dentate gyrus (DG) (Cameron et al., Citation1993; Kempermann et al., Citation2015) and the subventricular zone (SVZ) (Doetsch et al., Citation1999; Ponti et al., Citation2013). Recently, there is also some new evidence supporting the idea that selected neurogenesis may also occur in other brain regions, such as the hypothalamus, striatum, substantia nigra, cortex, and amygdala (Jurkowski et al., Citation2020). However, the role of the MR in relation to neurogenesis has so far only been studied in the hippocampus. Specifically, endogenous glucocorticoids are a known regulator of neurogenesis, and a prominent involvement of MR in neurogenesis and modulation of neuronal activity in the hippocampus has been demonstrated (Brown, Citation2008; Marver et al., Citation1974). Several studies have revealed increased proliferation following MR activation (Anacker et al., Citation2013; Fischer et al., Citation2002). Anacker et al. (Citation2013) discovered that activation of MR promoted proliferation after exposure to low concentrations of cortisol in hippocampal progenitor cells, and that MR mediated proliferation of astrocytes. In contrast, high concentrations of cortisol, which result in the additional activation of GR, lead to reduced hippocampal progenitor cell proliferation and neuronal differentiation, as well as inhibited MR-induced increases in astrogliosis. Mechanistically, low concentrations of cortisol were shown to enhance Notch/Hes signaling, while high concentrations of cortisol inhibit transforming growth factor-β-SMAD2/3 (TGFβ-SMAD2/3) signaling, both of which contribute to the proliferation of neural progenitor cells. In a prenatal stress model in rats, short-term stress and mild prenatal stress can enhance hippocampal neurogenesis and neuronal differentiation, which can improve the learning ability of adult offspring (Fujioka et al., Citation2001; Citation2006). Interestingly, only MR was demonstrated to be involved in this process. In this case, MR in fetal hippocampal neurons may be activated by corticosterone, while GR is only activated by high concentrations of corticosterone under long-term and severe stress (Fujioka et al., Citation1999).
Conversely, MR activation has been shown to block adrenalectomy-induced cell proliferation (Rodriguez et al., Citation1998). Montaron et al. (Citation2003) showed similar results, where low doses of MR agonist (20 mg/L aldosterone) prevented adrenalectomy-induced cell death, while high doses of MR agonist (30 mg/L aldosterone) prevented adrenalectomy-induced cell proliferation. These findings contradict those of previously mentioned studies, but the mechanisms of adrenalectomy and MR activation are not fully understood, and differences in these results may be due to a variety of reasons.
Similar to what was observed after adrenalectomy, Gass et al. (Citation2000) found reduced neurogenesis and amount of dentate granule cells in MR knockout mice, likely reflecting elevated corticosterone levels. Interestingly, a similar effect was not found in GR knockout mice, underlining an important role of the MR after adrenalectomy. A similar situation was observed in rats, with increased apoptosis in the dentate gyrus following administration of the GR agonist dexamethasone, possibly due to decreased MR activity and plasma corticosterone levels (Hassan et al., Citation1996; Sousa et al., Citation1999). Taken together, MR activation and the balance of MR and GR seem to be crucial to regulate hippocampal neurogenesis.
7.3. Neuroinflammation: Focus on microglia
A characteristic hallmark of chronic stress is the induction of pro-inflammatory mechanisms (Hayashi & Cortopassi, Citation2015; Miller & Sadeh, Citation2014). In numerous rodent experiments, researchers have found that microglia alter morphology, phagocytic activity, and synaptic plasticity, and are increased in sensitivity after chronic stress (Bisht et al., Citation2018). As microglia are the main immune cells of the central nervous system (CNS), their activation can lead to inflammation and neuronal damage following chronic stress. Glucocorticoids are important regulators of inflammation, and thus, MR and GR play important roles in the regulation of inflammation (Bay-Richter et al., Citation2012; Ozacmak et al., Citation2014). In microglia, MR and GR are co-expressed in the presence of 11β-HSD1 (Odermatt & Kratschmar, Citation2012). In regulating neuroinflammation and NF-κB activity in microglia, MR controls the NF-κB pathway and inflammatory mediators in microglia by coordinating with GR. 11β-HSD1 can locally regulate the balance mediated by MR and GR, and only low (20 nM) and moderate (50 nM) concentrations of corticosterone can promote the expression of interleukin 6 (IL-6) and tumor necrosis factor-α (TNF-α) in a MR-dependent manner (Chantong et al., Citation2012). After aldosterone treatment, LPS-induced interleukin-1β (IL-1β) and IL-6 mRNA expression was found to be increased in the PFC and CSF of rats (Bay-Richter et al., Citation2012). In the spontaneously hypertensive rat (SHR) model, lesion-induced brain tissue damage triggers a pro-inflammatory feedforward cascade driven by MR activation in response to endogenous corticosterone. In hippocampus, hyperactive MR and enhanced MR/GR co-localization promote inflammatory effects. Further, in spontaneously hypertensive rats, hyperactivation of MR is associated with a pro-inflammatory environment, resulting from an activated state of microglia (Brocca et al., Citation2019). CORT-activated MRs were also shown to mediate inflammatory and fibrotic responses in the presence of the 11β-HSD2 inhibitor carbenoxolone and salt (Wilson et al., Citation2009). Given these findings of MR in the brain’s inflammatory response, the role of MR in the regulation of CNS inflammation needs further, in-depth study.
8. MR and psychiatric disorders
There are many types of psychiatric disorders, but in general, they mainly manifest as disturbances in thinking and cognition, emotional regulation impairment, or behavioral abnormalities. In the following section, we will review the relationship between MR and psychiatric disorders in two parts: abnormal emotions and behaviors, and cognitive impairment.
8.1. Abnormal emotions and behaviors
Depression and Anxiety Spectrum Disorders are the major disorders of emotional and behavioral impairment in contemporary society. In the regulation of stress responses, MR and GR control adaptation to environmental demands in a complementary manner. Disruption of the MR/GR balance may lead to hyperactivity of the HPA axis, and this is one of the hypotheses for the etiology of depression (Qi et al., Citation2013). Hyperactivity of the HPA axis and elevated GC affect brain serotonin and stress adaptation-dependent responses, possibly leading to depression (Haleem & Gul, Citation2020; Keller et al., Citation2017). Persistent effects of stress on the HPA axis early in growth and development may lead to increased susceptibility to depression in adulthood (de Abreu Feijó de Mello et al., Citation2003; Heim et al., Citation2000; Shea et al., Citation2005). In adolescence, elevated cortisol often precedes the onset of depression and may be a risk factor of this disease (Zajkowska et al., Citation2022). Other researchers have discovered that in comparison to healthy individuals, patients experiencing depression have lower concentrations of morning cortisol and a disrupted daily rhythm of aldosterone release, resulting in an increased level of aldosterone at night (Izakova et al., Citation2020). Another study reported that compared with healthy controls, basal cortisol levels were elevated in depressed patients throughout the circadian cycle (Belvederi Murri et al., Citation2014). Irregular work schedules and chronic stress can lead to disruption of circadian rhythms and increase the risk of mental illness (den Boon & Sarabdjitsingh, Citation2017). In line with these observations, MR mRNA expression in hippocampus, prefrontal cortex, and cingulate gyrus is significantly reduced in depressed patients (Klok et al., Citation2011a; Medina et al., Citation2013; Patel et al., Citation2000). There is accumulating genetic and functional evidence for a direct contribution of MR to mood disorders, which is further amplified by sex differences. For example, the MR CA haplotype is associated with female susceptibility to depression, and this effect has also been linked to childhood abuse (Endedijk et al., Citation2020; Vinkers et al., Citation2015). Further, MR haplotype 2 genetic variants are associated with individual differences in antidepressant ability in women, as evidenced by more feelings of optimistm and fewer feelings of hopelessness (Klok et al., Citation2011b).
In addition, there are interesting associations of MR with 5-Hydroxytryptamin (serotonin, 5-HT), a monoamine neurotransmitter involved in the regulation of mood, learning and memory (Šalamon Arčan et al., Citation2022). The 5-HT1A receptor is abundant in the hippocampus, where it colocalizes with MR (Meltzer, Citation1989; Citation1990). 5-HT release and transport are inhibited by MR and GR, and 5-HT1A receptor-mediated hyperpolarization is reduced following MR downregulation (Joëls, Citation2001). Furthermore, it was shown that use of antidepressants can increase MR expression (DeRijk et al., Citation2008). During antidepressant treatment, adjunctive use of MR agonist fludrocortisone can reduce the latency of SSRIs therapeutic effect and improve efficacy (Otte et al., Citation2010).
Enhanced negative feedback of the HPA axis was found not only in patients with depression, but also in patients with post-traumatic stress disorder (PTSD) (Young et al., Citation1994). Individuals with no diagnosis but elevated scores on the Anxiety Scale, along with those diagnosed with anxiety disorders and depression, exhibit heightened sensitivity of the HPA axis to stress and greater susceptibility to post-traumatic stress disorder (Ancelin et al., Citation2017; Faye et al., Citation2018; Juruena et al., Citation2018; Lupien et al., Citation2018; Quevedo et al., Citation2019). After receiving the mineralocorticoid receptor antagonist spironolactone, patients with PTSD exhibit heightened cortisol responses and increased cortisol production in response to CRH activation (Kellner et al., Citation2002). Other investigators have sought to explore changes in HPA response and its relationship to MR by blocking cortisol synthesis with metyrapone, but several studies have found inconsistent results. One study reported no significant differential HPA response between PTSD patients and controls (Kellner et al., Citation2004), while in another study, PTSD patients have been shown to have a decreased ACTH response to metyrapone (Neylan et al., Citation2003). Yet another study found increased ACTH and cortisol values in PTSD patients and no group differences in salt corticosteroid receptor-mediated feedback inhibition (Otte et al., Citation2006). In PTSD patients, abnormally low or down-regulated MR in hippocampus may result in considerable tissue loss (Zhe et al., Citation2008). However, the role of MR in anxiety behavior remains controversial and a recent study has found that MR may contribute differentially to depressive or anxiety symptoms. After adrenalectomy, the patient’s aldosterone level returned to normal, and the depression scale showed relief of depressive symptoms, but the anxiety scale scores did not change significantly; with MR antagonists, the patient had high levels of aldosterone, and anxiety scales showed improvement in anxiety symptoms, but there were no significant changes in depression scale scores (Murck et al., Citation2021). There are also findings suggesting that patients with increased aldosterone are more prone to comorbidities of anxiety disorder (Sonino et al., Citation2011). Thus, further research is needed to clarify the role of MR in anxiety (Kellner & Wiedemann, Citation2008).
In addition to depression and anxiety spectrum disorders, there are also other psychiatric disorders with emotional-behavioral disturbances that were associated with MR expression or function. For example, The NR3C2-4 region is highly methylated in female schizophrenic patients (Qing et al., Citation2020). Decreased MR mRNA expression was found in postmortem prefrontal cortex of bipolar disorder (BP) and schizophrenia patients (Xing et al., Citation2004). Moreover, treatment with the MR antagonist spironolactone partially improved anxiety symptoms in patients with bipolar disorder (Juruena et al., Citation2009) and has been proposed as a potential therapeutic agent for improving negative symptoms, such as lack of motivation, anhedonia, apathy, social withdrawal (Zandifar et al., Citation2022). Furthermore, MR stimulation has been shown to improve affective empathy in individuals. Wingenfeld et al. (Citation2014) found that fludrocortisone-stimulated MR enhances emotional empathy in women with borderline personality disorder (BPD).
In rodent animal models, the HPA axis was also more sensitive to stress exposure in animals that exhibited anxiety- and depression-like behaviors (Faye et al., Citation2018; Fox et al., Citation2018; Goncharova & Oganyan, Citation2018; Oh et al., Citation2018). Hippocampal MR was shown to affect anxiety-like behavior in rats (Smythe et al., Citation1997). For example animals lacking forebrain MR exhibit increased anxiety-like behaviors (Brinks et al., Citation2009) whereas MR overexpression in the forebrain can reduce anxiety behavior (Lai et al., Citation2007; Mitra et al., Citation2009). In a social isolation model in rats, MR expression was found to be down-regulated in the hippocampus, which may lead to reduced resilience to acute stress (Boero et al., Citation2018). A similar result was found in another study, where acute restraint stress of less than 2 hours reduced MR heteronuclear RNA in hippocampus, but not MR mRNA (Herman & Watson, Citation1995). However, it has also been shown that acute stress (forced swimming and novelty stress) caused an increase in MR expression levels in the rat hippocampus, followed by an increased inhibitory tonus mediated by MR on HPA axis activity (Gesing et al., Citation2001). Importantly, while most studies focused exclusively on males, there are also several reports showing that MR effects are sex-specific. For example, chronic severe stress increases MR expression in the hippocampus of female adult rats, but not males. However, when rats were exposed to chronic unpredictable stress when they were young, females showed MR downregulation, but males showed MR upregulation as they matured. This difference may be related to the length of time between rats being subjected to stress stimuli and being sacrificed (Karandrea et al., Citation2000; Kitraki et al., Citation2004). Chronic corticosterone treatment in mice resulted in decreased hippocampal and hypothalamic MR expression and depressive-like behaviors, but co-administration of the MR antagonist spironolactone prevented the mice from developing depression-like behaviors (Wu et al., Citation2013). The aldosterone system has been observed to have a correlation with depressive symptoms, and prolonged administration of the mineralocorticoid aldosterone may result in augmented depression- and anxiety-like behaviors; however, the underlying mechanism remains to be elucidated (Bay-Richter et al., Citation2012; Hlavacova et al., Citation2012; Hlavacova & Jezova, Citation2008). In addition, researchers found that non-genomic effects of MR may modulate anxiety-like behaviors, as anxiety-like behaviors occur 30 minutes after MR antagonist administration (Chen et al., Citation2019; Groeneweg et al., Citation2012).
8.2. Cognitive impairment
Glutamate is essential for the creation and maintenance of synapses, learning and memory, and cellular metabolism. Glucocorticoids, and both acute and chronic stress stimulate glutamate release in the brain (Popoli et al., Citation2011). In response to stress, CA1 hippocampal excitability is increased, resulting in MR activation via a quick and transitory non-genomic action and an increase in presynaptic membrane glutamate release (Karst et al., Citation2005; Olijslagers et al., Citation2008). With the assistance of limbic system-related membrane proteins (Qiu et al., Citation2010), MR can facilitate information acquisition and retrieval of stored information, enabling humans to govern the evaluation of novel settings and pick appropriate behavioral response strategies. However, the increase in excitability and the release of glutamate are inhibited by the activated GR (de Kloet et al., Citation2009; Myers et al., Citation2014; Oitzl & de Kloet, Citation1992). Several investigations have demonstrated that non-contextual delivery of stress or use of the MR antagonist spironolactone prior to contextual fear conditioning can lessen contextual fear by interfering with memory formation via non-genomic effects (Sajadi et al., Citation2006; Zhou et al., Citation2010, Citation2011). Other studies have indicated that the MR antagonist spironolactone produces impairments in long-term potentiation (LTP), selective attention, and working memory function under stress (Avital et al., Citation2006; Cornelisse et al., Citation2011). Stimulating MR with flucortisone induces more emotional risk-behavior responses in young, healthy participants rather than conservative rational strategies (Deuter et al., Citation2017).
An important aspect of MR function, especially in the context of cognition, is its effect on the neuronal network level. MR has been reported to modulate neurotransmitter release from presynaptic terminals (Gulyaeva, Citation2021), but so far it is largely unclear how MR function at this level is regulated by neuronal activity. There are few studies suggesting that stress-induced changes in learning and memory may involve upregulation of connections between the amygdala and the hippocampus and striatum, which are sensitive to MR blockade (Schwabe et al., Citation2013). Further, following stress exposure MR-dependent amygdala activity is delayed, suggesting a time-dependent effect of stress on neural activity and memory processes (Vogel et al., Citation2017). While GR activation is believed to involve information acquirement and memory consolidation, the process of MR activation involves the utilization of contextual information retrieval (Joëls, Citation2008; Joëls et al., Citation2012; Lupien & McEwen, Citation1997; Roozendaal et al., Citation2009; Zhou et al., Citation2011). However, further studies are needed to elucidate the role of MR especially on the non-genomic level to affect neurotransmission and consequently brain circuit activity.
MR has been demonstrated in many clinical studies to affect cognitive function in patients with mental illness. In mild cognitive impairment (MCI) patients, cognition is impaired possibly because of the reduced MR/GR ratio due to hippocampal atrophy (Kline & Mega, Citation2020). The MR antagonist spironolactone ameliorated β-amyloid (Aβ) induced cognitive impairment in Alzheimer’s disease and reduced expression of Aβ (Chen et al., Citation2020). The situation is more complex with MR agonist treatment, where depending on context and setting, both improvements and impairments of memory performance were reported. Among depressed subjects, only older patients had impaired verbal learning and visuospatial memory following treatment with the MR agonist fludrocortisone (Otte, Wingenfeld, Kuehl, Richter, et al., Citation2015). Conversely, fludrocortisone significantly improved memory and executive function in young depressed patients (Otte, Wingenfeld, Kuehl, Kaczmarczyk, et al., Citation2015). PTSD patients and BPD patients improved working memory after fludrocortisone treatment (Wingenfeld & Wolf, Citation2015). However, another study has found that in women with BPD, verbal learning and visuospatial memory were impaired in comparison with controls (Wingenfeld & Otte, Citation2019). Based on these studies, MR agonist therapy seems to have different effects on different types of patients. Finally, there are also genetic studies showing that under stress induction, carriers of the MR gene Val variant have deficits in reward learning, exhibiting an impaired ability to modulate behavior (Bogdan et al., Citation2010). MR variation in the hippocampus and medial temporal region is associated with encoding and retrieval of long-term memory, and some NR3C2 single-nucleotide polymorphism (SNPs) can cause changes in verbal memory performance (Keller et al., Citation2017). Together, these data underline the important role of MR in cognitive function, but the directionality of the effect is still controversial and likely context-dependent.
The important role of MR in cognition has been corroborated using animal studies. Knockout of MR in the mouse forebrain leads to impaired learning, deficits in working memory (Berger et al., Citation2006), and improved spatial memory following increased neuronal MR expression (Lai et al., Citation2007; Mitra et al., Citation2009; Rozeboom et al., Citation2007). Further, rats injected with spironolactone have impaired spatial memory and altered behavioral strategies (Oitzl & de Kloet, Citation1992; Yau et al., Citation1999). Interestingly, other studies have shown the opposite result: chronic administration of MR antagonist spironolactone to Nrg1-tg transgenic mice partially improved working memory, and improved the manifestations of what are considered human schizophrenia-positive symptoms (Wehr et al., Citation2017). Similar to studies in humans the corticosterone synthesis blocker metyrapone was also used to differentiate between MR and GR-mediated effects on cognition. Using a higher dose of metyrapone (50 mg/kg) to inhibit corticosterone synthesis in male Sprague-Dawley rats, the latency of animals looking for an escape platform in Morris water maze (MWM) was significantly longer than that in the control group (Roozendaal et al., Citation1996). The high dose of metyrapone also dramatically decreased the percentage of fear-induced immobility. This may be due to a sharp drop in corticosterone levels that reduced MR occupancy, resulting in impaired information acquisition performance (Oitzl & de Kloet, Citation1992; Roozendaal et al., Citation1996). However, more recent studies also question the utility of metyrapone due to apparent off-target effects on MR and GR activity (Kennedy et al., Citation2020). The different outcomes of genetic versus pharmacological MR manipulation might be due to developmental effects of MR deletion, time-dependence of MR deactivation or region- and cell type-specificity of the effects. For example, McEown and Treit’s study (McEown & Treit, Citation2011) showed that in the three brain regions, dorsal hippocampus, ventral hippocampus and medial prefrontal cortex (mPFC), only after the ventral hippocampus was injected with MR antagonists, would unconditioned fear of rats be reduced. The effect of MR on cognitive function was also shown to be related to sex. Following chronic stress, increased MR expression was found only in the hippocampal cornu Ammonis 3 (CA3) region of female, but not male rats, paralleling their spatial memory improvement (Kitraki et al., Citation2004). Forebrain MR-deficient female mice were unable to distinguish between cued and contextual fears as accurately as male mice in a fear conditioning memory test (Ter Horst et al., Citation2012). Taken together, the animal data on MR function on cognition support the human data, showing that MR modulation has a direct effect on cognitive processes that are dependent on the specific context, including brain region, the cell type, the external condition, and sex.
9. Conlusions
In conclusion, we mainly reviewed the physiological structure and function of MR in the brain, and we assert that MR is closely related to various psychiatric disorders. In psychiatric disorders, activation of the steroid receptors MR and GR modulates memory, behavior, and mood. With the knowledge we have gained from MR rat models, mouse models, and human psychiatric disorders, it should be envisaged that MR becomes a target for various psychotropic drug treatments involving hippocampal dysfunction or altered HPA axis function.
Disclosure statement
No potential conflict of interest was reported by the author(s).
Additional information
Funding
Notes on contributors
Huanqing Yang
Huanqing Yang obtained her Bachelor’s degree in Medicine from Qiqihar Medical University and her Master’s degree in Medicine from Shanghai Jiaotong University School of Medicine in China. During her postgraduate studies, she conducted research on the fundamental and clinical aspects of neurocognitive disorders. She worked as a psychiatrist at Kangning Hospital Affiliated to Wenzhou Medical University in China for five years, obtained the qualification of practicing physician and completed her resident training. Currently, she is studying for a Ph.D. in Mathias V. Schmidt’s research group “Neurobiology of Stress Resilience” at the Max Planck Institute of Psychiatry.
Sowmya Narayan
Sowmya Narayan completed a Bachelor’s degree at the University of Minnesota, Twin Cities and then joined the Max Planck Florida Institute for Neuroscience as a post-baccalaureate researcher. Currently she is a PhD student in the research groups of Mathias V. Schmidt and Elisabeth Binder at the Max Planck Institute for Psychiatry.
Mathias V. Schmidt
Mathias V. Schmidt received his undergraduate and graduate training at the University of Bayreuth, Germany and the University of Delaware, USA, where he first became involved in stress research through the guidance of Prof. Seymour “Gig” Levine. He continued his research in the field of stress and development during his PhD training at the University of Leiden, the Netherlands, with Prof. Ron de Kloet. Mathias then moved to Munich, Germany, where he started his postdoctoral work at the Max Planck Institute of Psychiatry. Since 2010 he is leading the research group “Neurobiology of Stress Resilience” at the Max Planck Institute of Psychiatry.
References
- Anacker, C., Cattaneo, A., Luoni, A., Musaelyan, K., Zunszain, P. A., Milanesi, E., Rybka, J., Berry, A., Cirulli, F., Thuret, S., Price, J., Riva, M. A., Gennarelli, M., & Pariante, C. M. (2013). Glucocorticoid-related molecular signaling pathways regulating hippocampal neurogenesis. Neuropsychopharmacology: official Publication of the American College of Neuropsychopharmacology, 38(5), 1–17. https://doi.org/10.1038/npp.2012.253
- Ancelin, M.-L., Scali, J., Norton, J., Ritchie, K., Dupuy, A.-M., Chaudieu, I., & Ryan, J. (2017). Heterogeneity in HPA axis dysregulation and serotonergic vulnerability to depression. Psychoneuroendocrinology, 77, 90–94. https://doi.org/10.1016/j.psyneuen.2016.11.016
- Antoni, F. A. (2019). Magnocellular vasopressin and the mechanism of “glucocorticoid escape. Frontiers in Endocrinology, 10, 422. https://doi.org/10.3389/fendo.2019.00422
- Arnal, J.-F., Lenfant, F., Metivier, R., Flouriot, G., Henrion, D., Adlanmerini, M., Fontaine, C., Gourdy, P., Chambon, P., Katzenellenbogen, B., & Katzenellenbogen, J. (2017). Membrane and nuclear estrogen receptor alpha actions: from tissue specificity to medical implications. Physiological Reviews, 97(3), 1045–1087. https://doi.org/10.1152/physrev.00024.2016
- Arriza, J. L., Weinberger, C., Cerelli, G., Glaser, T. M., Handelin, B. L., Housman, D. E., & Evans, R. M. (1987). Cloning of human mineralocorticoid receptor complementary DNA: Structural and functional kinship with the glucocorticoid receptor. Science (New York, N.Y.), 237(4812), 268–275. https://doi.org/10.1126/science.3037703
- Ashton, A. W., Le, T. Y. L., Gomez-Sanchez, C. E., Morel-Kopp, M.-C., McWhinney, B., Hudson, A., & Mihailidou, A. S. (2015). Role of nongenomic signaling pathways activated by aldosterone during cardiac reperfusion injury. Molecular Endocrinology (Baltimore, Md.), 29(8), 1144–1155. https://doi.org/10.1210/ME.2014-1410
- Avital, A., Segal, M., & Richter-Levin, G. (2006). Contrasting roles of corticosteroid receptors in hippocampal plasticity. The Journal of Neuroscience : The Official Journal of the Society for Neuroscience, 26(36), 9130–9134. https://doi.org/10.1523/JNEUROSCI.1628-06.2006
- Baker, M. E., Funder, J. W., & Kattoula, S. R. (2013). Evolution of hormone selectivity in glucocorticoid and mineralocorticoid receptors. The Journal of Steroid Biochemistry and Molecular Biology, 137, 57–70. https://doi.org/10.1016/j.jsbmb.2013.07.009
- Baker, M. E., & Katsu, Y. (2020). Progesterone: An enigmatic ligand for the mineralocorticoid receptor. Biochemical Pharmacology, 177, 113976. https://doi.org/10.1016/j.bcp.2020.113976
- Bast, B.-O., Rickert, U., Schneppenheim, J., Cossais, F., Wilms, H., Arnold, P., & Lucius, R. (2018). Aldosterone exerts anti-inflammatory effects on LPS stimulated microglia. Heliyon, 4(10), e00826. https://doi.org/10.1016/j.heliyon.2018.e00826
- Bay-Richter, C., Hallberg, L., Ventorp, F., Janelidze, S., & Brundin, L. (2012). Aldosterone synergizes with peripheral inflammation to induce brain IL-1β expression and depressive-like effects. Cytokine, 60(3), 749–754. https://doi.org/10.1016/j.cyto.2012.08.016
- Belvederi Murri, M., Pariante, C., Mondelli, V., Masotti, M., Atti, A. R., Mellacqua, Z., Antonioli, M., Ghio, L., Menchetti, M., Zanetidou, S., Innamorati, M., & Amore, M. (2014). HPA axis and aging in depression: Systematic review and meta-analysis. Psychoneuroendocrinology, 41, 46–62. https://doi.org/10.1016/j.psyneuen.2013.12.004
- Berardelli, R., Karamouzis, I., D’Angelo, V., Zichi, C., Fussotto, B., Giordano, R., Ghigo, E., & Arvat, E. (2013). Role of mineralocorticoid receptors on the hypothalamus–pituitary–adrenal axis in humans. Endocrine, 43(1), 51–58. https://doi.org/10.1007/s12020-012-9750-8
- Berardelli, R., Karamouzis, I., Marinazzo, E., Prats, E., Picu, A., Giordano, R., Ghigo, E., & Arvat, E. (2010). Effect of acute and prolonged mineralocorticoid receptor blockade on spontaneous and stimulated hypothalamic–pituitary–adrenal axis in humans. European Journal of Endocrinology, 162(6), 1067–1074. https://doi.org/10.1530/EJE-09-1076
- Berger, S., Wolfer, D. P., Selbach, O., Alter, H., Erdmann, G., Reichardt, H. M., Chepkova, A. N., Welzl, H., Haas, H. L., Lipp, H.-P., & Schütz, G. (2006). Loss of the limbic mineralocorticoid receptor impairs behavioral plasticity. Proceedings of the National Academy of Sciences of the United States of America, 103(1), 195–200. https://doi.org/10.1073/pnas.0503878102
- Bisht, K., Sharma, K., & Tremblay, M.-È. (2018). Chronic stress as a risk factor for Alzheimer’s disease: Roles of microglia-mediated synaptic remodeling, inflammation, and oxidative stress. Neurobiology of Stress, 9, 9–21. https://doi.org/10.1016/j.ynstr.2018.05.003
- Boero, G., Pisu, M. G., Biggio, F., Muredda, L., Carta, G., Banni, S., Paci, E., Follesa, P., Concas, A., Porcu, P., & Serra, M. (2018). Impaired glucocorticoid-mediated HPA axis negative feedback induced by juvenile social isolation in male rats. Neuropharmacology, 133, 242–253. https://doi.org/10.1016/j.neuropharm.2018.01.045
- Bogdan, R., Perlis, R. H., Fagerness, J., & Pizzagalli, D. A. (2010). The impact of mineralocorticoid receptor ISO/VAL Genotype (rs5522) and stress on reward learning. Genes, Brain, and Behavior, 9(6), 658–667. https://doi.org/10.1111/j.1601-183X.2010.00600.x
- Brinks, V., Berger, S., Gass, P., de Kloet, E. R., & Oitzl, M. S. (2009). Mineralocorticoid receptors in control of emotional arousal and fear memory. Hormones and Behavior, 56(2), 232–238. https://doi.org/10.1016/j.yhbeh.2009.05.003
- Brocca, M. E., Pietranera, L., de Kloet, E. R., & De Nicola, A. F. (2019). Mineralocorticoid receptors, neuroinflammation and hypertensive encephalopathy. Cellular and Molecular Neurobiology, 39(4), 483–492. https://doi.org/10.1007/s10571-018-0610-9
- Brown, N. J. (2008). Aldosterone and vascular inflammation. Hypertension (Dallas, Tex. : 1979), 51(2), 161–167. https://doi.org/10.1161/HYPERTENSIONAHA.107.095489
- Buckley, T. M., Mullen, B. C., & Schatzberg, A. F. (2007). The acute effects of a mineralocorticoid receptor (MR) agonist on nocturnal hypothalamic–adrenal–pituitary (HPA) axis activity in healthy controls. Psychoneuroendocrinology, 32(8-10), 859–864. https://doi.org/10.1016/j.psyneuen.2007.05.016
- Cameron, H. A., Woolley, C. S., McEwen, B. S., & Gould, E. (1993). Differentiation of newly born neurons and glia in the dentate gyrus of the adult rat. Neuroscience, 56(2), 337–344. https://doi.org/10.1016/0306-4522(93)90335-d
- Chantong, B., Kratschmar, D. V., Nashev, L. G., Balazs, Z., & Odermatt, A. (2012). Mineralocorticoid and glucocorticoid receptors differentially regulate NF-kappaB activity and pro-inflammatory cytokine production in murine BV-2 microglial cells. Journal of Neuroinflammation, 9(1), 260. https://doi.org/10.1186/1742-2094-9-260
- Chen, L., Shi, R., She, X., Gu, C., Chong, L., Zhang, L., & Li, R. (2020). Mineralocorticoid receptor antagonist-mediated cognitive improvement in a mouse model of Alzheimer’s type: Possible involvement of BDNF-H2 S-Nrf2 signaling. Fundamental & Clinical Pharmacology, 34(6), 697–707. https://doi.org/10.1111/fcp.12576
- Chen, J., Wang, Z.-Z., Zhang, S., Chu, S.-F., Mou, Z., & Chen, N.-H. (2019). The effects of glucocorticoids on depressive and anxiety-like behaviors, mineralocorticoid receptor-dependent cell proliferation regulates anxiety-like behaviors. Behavioural Brain Research, 362, 288–298. https://doi.org/10.1016/j.bbr.2019.01.026
- Chen, J., Wang, Z., Zhang, S., Zuo, W., & Chen, N. (2016). Does mineralocorticoid receptor play a vital role in the development of depressive disorder? Life Sciences, 152, 76–81. https://doi.org/10.1016/j.lfs.2016.03.022
- Christy, C., Hadoke, P. W. F., Paterson, J. M., Mullins, J. J., Seckl, J. R., & Walker, B. R. (2003). 11beta-hydroxysteroid dehydrogenase type 2 in mouse aorta: Localization and influence on response to glucocorticoids. Hypertension (Dallas, Tex. : 1979), 42(4), 580–587. https://doi.org/10.1161/01.HYP.0000088855.06598.5B
- Cizza, G., & Rother, K. I. (2012). Cortisol binding globulin: More than just a carrier? The Journal of Clinical Endocrinology and Metabolism, 97(1), 77–80. https://doi.org/10.1210/jc.2011-3090
- Cole, T. J., & Young, M. J. (2017). 30 Years Of The Mineralocorticoid receptor: Mineralocorticoid receptor null mice: informing cell-type-specific roles. Journal of Endocrinology, 234(1), T83–T92. https://doi.org/10.1530/JOE-17-0155
- Cornelisse, S., Joëls, M., & Smeets, T. (2011). A randomized trial on mineralocorticoid receptor blockade in men: Effects on stress responses, selective attention, and memory. Neuropsychopharmacology: official Publication of the American College of Neuropsychopharmacology, 36(13), 2720–2728. https://doi.org/10.1038/npp.2011.162
- Couette, B., Fagart, J., Jalaguier, S., Lombes, M., Souque, A., & Rafestin-Oblin, M. E. (1996). Ligand-induced conformational change in the human mineralocorticoid receptor occurs within its hetero-oligomeric structure. Biochemical Journal, 315(2), 421–427. https://doi.org/10.1042/bj3150421
- Coutinho, P., Vega, C., Pojoga, L. H., Rivera, A., Prado, G. N., Yao, T. M., Adler, G., Torres-Grajales, M., Maldonado, E. R., Ramos-Rivera, A., Williams, J. S., Williams, G., & Romero, J. R. (2014). Aldosterone’s rapid, nongenomic effects are mediated by striatin: A modulator of aldosterone’s effect on estrogen action. Endocrinology, 155(6), 2233–2243. https://doi.org/10.1210/en.2013-1834
- de Abreu Feijó de Mello, A., Feijó de Mello, M., Carpenter, L. L., & Price, L. H. (2003). Update on stress and depression: The role of the hypothalamic-pituitary-adrenal (HPA) axis. Revista Brasileira de Psiquiatria (Sao Paulo, Brazil : 1999), 25(4), 231–238. https://doi.org/10.1590/s1516-44462003000400010
- den Boon, F. S., & Sarabdjitsingh, R. A. (2017). Circadian and ultradian patterns of HPA-axis activity in rodents: Significance for brain functionality. Best Practice & Research: Clinical Endocrinology & Metabolism, 31(5), 445–457. https://doi.org/10.1016/j.beem.2017.09.001
- de Kloet, E. R., Fitzsimons, C. P., Datson, N. A., Meijer, O. C., & Vreugdenhil, E. (2009). Glucocorticoid signaling and stress-related limbic susceptibility pathway: About receptors, transcription machinery and microRNA. Brain Research, 1293, 129–141. https://doi.org/10.1016/j.brainres.2009.03.039
- De Nicola, A. F., Meyer, M., Guennoun, R., Schumacher, M., Hunt, H., Belanoff, J., de Kloet, E. R., & Gonzalez Deniselle, M. C. (2020). Insights into the therapeutic potential of glucocorticoid receptor modulators for neurodegenerative diseases. International Journal of Molecular Sciences, 21(6), 2137. https://doi.org/10.3390/ijms21062137
- De Nobrega, A. K., Luz, K. V., & Lyons, L. C. (2020). Resetting the aging clock: Implications for managing age-related diseases. In P. C. Guest (Ed.), Reviews on new drug targets in age-related disorders (pp. 193–265). Springer International Publishing. https://doi.org/10.1016/j.beem.2017.09.001
- DeRijk, R. H., van Leeuwen, N., Klok, M. D., & Zitman, F. G. (2008). Corticosteroid receptor-gene variants: Modulators of the stress-response and implications for mental health. European Journal of Pharmacology, 585(2-3), 492–501. https://doi.org/10.1016/j.ejphar.2008.03.012
- Deuter, C. E., Wingenfeld, K., Schultebraucks, K., Hellmann-Regen, J., Piber, D., & Otte, C. (2017). Effects of mineralocorticoid-receptor stimulation on risk taking behavior in young healthy men and women. Psychoneuroendocrinology, 75, 132–140. https://doi.org/10.1016/j.psyneuen.2016.10.018
- Doetsch, F., Caillé, I., Lim, D. A., García-Verdugo, J. M., & Alvarez-Buylla, A. (1999). Subventricular zone astrocytes are neural stem cells in the adult mammalian brain. Cell, 97(6), 703–716. https://doi.org/10.1016/s0092-8674(00)80783-7
- Edwards, C. R., Stewart, P. M., Burt, D., Brett, L., McIntyre, M. A., Sutanto, W. S., de Kloet, E. R., & Monder, C. (1988). Localisation of 11 beta-hydroxysteroid dehydrogenase—Tissue specific protector of the mineralocorticoid receptor. Lancet (London, England), 2(8618), 986–989. https://doi.org/10.1016/s0140-6736(88)90742-8
- Endedijk, H. M., Nelemans, S. A., Schür, R. R., Boks, M. P. M., van Lier, P., Meeus, W., Branje, S., & Vinkers, C. H. (2020). The role of stress and mineralocorticoid receptor haplotypes in the development of symptoms of depression and anxiety during adolescence. Frontiers in Psychiatry, 11, 367. https://doi.org/10.3389/fpsyt.2020.00367
- Fan, Y. S., Eddy, R. L., Byers, M. G., Haley, L. L., Henry, W. M., Nowak, N. J., & Shows, T. B. (1989). The human mineralocorticoid receptor gene (MLR) is located on chromosome 4 at q31.2. Cytogenetics and Cell Genetics, 52(1-2), 83–84. https://doi.org/10.1159/000132846
- Faresse, N. (2014). Post-translational modifications of the mineralocorticoid receptor: How to dress the receptor according to the circumstances? The Journal of Steroid Biochemistry and Molecular Biology, 143, 334–342. https://doi.org/10.1016/j.jsbmb.2014.04.015
- Faye, C., McGowan, J. C., Denny, C. A., & David, D. J. (2018). Neurobiological mechanisms of stress resilience and implications for the aged population. Current Neuropharmacology, 16(3), 234–270. https://doi.org/10.2174/1570159X15666170818095105
- Fink, G. (2016). Stress: Neuroendocrinology and Neurobiology: Handbook of Stress Series. Academic Press.
- Finsterwald, C., & Alberini, C. M. (2014). Stress and glucocorticoid receptor-dependent mechanisms in long-term memory: From adaptive responses to psychopathologies. Neurobiology of Learning and Memory, 112, 17–29. https://doi.org/10.1016/j.nlm.2013.09.017
- Fischer, K., Kelly, S. M., Watt, K., Price, N. C., & McEwan, I. J. (2010). Conformation of the mineralocorticoid receptor n-terminal domain: Evidence for induced and stable structure. Molecular Endocrinology (Baltimore, Md.), 24(10), 1935–1948. https://doi.org/10.1210/me.2010-0005
- Fischer, A. K., von Rosenstiel, P., Fuchs, E., Goula, D., Almeida, O. F. X., & Czéh, B. (2002). The prototypic mineralocorticoid receptor agonist aldosterone influences neurogenesis in the dentate gyrus of the adrenalectomized rat. Brain Research, 947(2), 290–293. https://doi.org/10.1016/s0006-8993(02)03042-1
- Fox, A. S., Oler, J. A., Birn, R. M., Shackman, A. J., Alexander, A. L., & Kalin, N. H. (2018). Functional connectivity within the primate extended amygdala is heritable and associated with early-life anxious temperament. The Journal of Neuroscience : The Official Journal of the Society for Neuroscience, 38(35), 7611–7621. https://doi.org/10.1523/JNEUROSCI.0102-18.2018
- Fru, K. N., VandeVoort, C. A., & Chaffin, C. L. (2006). Mineralocorticoid synthesis during the periovulatory interval in macaques. Biology of Reproduction, 75(4), 568–574. https://doi.org/10.1095/biolreprod.106.053470
- Fujioka, A., Fujioka, T., Ishida, Y., Maekawa, T., & Nakamura, S. (2006). Differential effects of prenatal stress on the morphological maturation of hippocampal neurons. Neuroscience, 141(2), 907–915. https://doi.org/10.1016/j.neuroscience.2006.04.046
- Fujioka, T., Fujioka, A., Tan, N., Chowdhury, G. M., Mouri, H., Sakata, Y., & Nakamura, S. (2001). Mild prenatal stress enhances learning performance in the non-adopted rat offspring. Neuroscience, 103(2), 301–307. https://doi.org/10.1016/s0306-4522(00)00582-0
- Fujioka, T., Sakata, Y., Yamaguchi, K., Shibasaki, T., Kato, H., & Nakamura, S. (1999). The effects of prenatal stress on the development of hypothalamic paraventricular neurons in fetal rats. Neuroscience, 92(3), 1079–1088. https://doi.org/10.1016/s0306-4522(99)00073-1
- Fuller, P. J. (2015). Novel interactions of the mineralocorticoid receptor. Molecular and Cellular Endocrinology, 408, 33–37. https://doi.org/10.1016/j.mce.2015.01.027
- Fuller, P. J., & Verity, K. (1990). Mineralocorticoid receptor gene expression in the gastrointestinal tract: Distribution and ontogeny. Journal of Steroid Biochemistry, 36(4), 263–267. https://doi.org/10.1016/0022-4731(90)90215-e
- Fuller, P. J., Yao, Y., Yang, J., & Young, M. J. (2012). Mechanisms of ligand specificity of the mineralocorticoid receptor. The Journal of Endocrinology, 213(1), 15–24. https://doi.org/10.1530/JOE-11-0372
- Fuller, P. J., & Young, M. J. (2005). Mechanisms of mineralocorticoid action. Hypertension (Dallas, Tex. : 1979), 46(6), 1227–1235. https://doi.org/10.1161/01.HYP.0000193502.77417.17
- Funder, J. W. (1991). Steroids, receptors, and response elements: The limits of signal specificity. Recent Progress in Hormone Research, 47, 191–207. discussion 207-210. https://doi.org/10.1016/b978-0-12-571147-0.50010-x
- Funder, J. W., Pearce, P. T., Smith, R., & Smith, A. I. (1988). Mineralocorticoid action: Target tissue specificity is enzyme, not receptor, mediated. Science (New York, N.Y.), 242(4878), 583–585. https://doi.org/10.1126/science.2845584
- Gadasheva, Y., Nolze, A., & Grossmann, C. (2021). Posttranslational modifications of the mineralocorticoid receptor and cardiovascular aging. Frontiers in Molecular Biosciences, 8, 667990. https://doi.org/10.3389/fmolb.2021.667990
- Gaffey, A. E., Bergeman, C. S., Clark, L. A., & Wirth, M. M. (2016). Aging and the HPA axis: Stress and resilience in older adults. Neuroscience and Biobehavioral Reviews, 68, 928–945. https://doi.org/10.1016/j.neubiorev.2016.05.036
- Gasparini, S., Resch, J. M., Narayan, S. V., Peltekian, L., Iverson, G. N., Karthik, S., & Geerling, J. C. (2019). Aldosterone-sensitive HSD2 neurons in mice. Brain Structure & Function, 224(1), 387–417. https://doi.org/10.1007/s00429-018-1778-y
- Gass, P., Kretz, O., Wolfer, D. P., Berger, S., Tronche, F., Reichardt, H. M., Kellendonk, C., Lipp, H.-P., Schmid, W., & Schütz, G. (2000). Genetic disruption of mineralocorticoid receptor leads to impaired neurogenesis and granule cell degeneration in the hippocampus of adult mice. EMBO Reports, 1(5), 447–451. https://doi.org/10.1093/embo-reports/kvd088
- GBD 2019 Mental Disorders Collaborators. (2022). Global, regional, and national burden of 12 mental disorders in 204 countries and territories, 1990–2019: A systematic analysis for the Global Burden of Disease Study 2019. The Lancet. Psychiatry, 9(2), 137–150. https://doi.org/10.1016/S2215-0366(21)00395-3
- Geerling, J. C., Kawata, M., & Loewy, A. D. (2006). Aldosterone-sensitive neurons in the rat central nervous system. The Journal of Comparative Neurology, 494(3), 515–527. https://doi.org/10.1002/cne.20808
- Gesing, A., Bilang-Bleuel, A., Droste, S. K., Linthorst, A. C., Holsboer, F., & Reul, J. M. (2001). Psychological stress increases hippocampal mineralocorticoid receptor levels: Involvement of corticotropin-releasing hormone. The Journal of Neuroscience : The Official Journal of the Society for Neuroscience, 21(13), 4822–4829. https://doi.org/10.1523/JNEUROSCI.21-13-04822.2001
- Gomez-Sanchez, E. (2014). Brain mineralocorticoid receptors in cognition and cardiovascular homeostasis. Steroids, 91, 20–31. https://doi.org/10.1016/j.steroids.2014.08.014
- Gomez-Sanchez, E., & Gomez-Sanchez, C. E. (2014). The multifaceted mineralocorticoid receptor. In R. Terjung (Ed.), Comprehensive Physiology (1st ed., pp. 965–994). Wiley. https://doi.org/10.1002/cphy
- Goncharova, N. D. (2020). The HPA axis under stress and aging: individual vulnerability is associated with behavioral patterns and exposure time. BioEssays, 42(9), 2000007. https://doi.org/10.1002/bies.202000007
- Goncharova, N. D., & Oganyan, T. E. (2018). Age-related differences in stress responsiveness of the hypothalamic-pituitary-adrenal axis of nonhuman primates with various types of adaptive behavior. General and Comparative Endocrinology, 258, 163–172. https://doi.org/10.1016/j.ygcen.2017.08.007
- Gorini, S., Kim, S. K., Infante, M., Mammi, C., La Vignera, S., Fabbri, A., Jaffe, I. Z., & Caprio, M. (2019). Role of Aldosterone and Mineralocorticoid Receptor in Cardiovascular Aging. Frontiers in Endocrinology, 10, 584. https://doi.org/10.3389/fendo.2019.00584
- Groeneweg, F. L., Karst, H., de Kloet, E. R., & Joëls, M. (2012). Mineralocorticoid and glucocorticoid receptors at the neuronal membrane, regulators of nongenomic corticosteroid signalling. Molecular and Cellular Endocrinology, 350(2), 299–309. https://doi.org/10.1016/j.mce.2011.06.020
- Grossmann, C., Almeida-Prieto, B., Nolze, A., & Alvarez de la Rosa, D. (2022). Structural and molecular determinants of mineralocorticoid receptor signalling. British Journal of Pharmacology, 179(13), 3103–3118. https://doi.org/10.1111/bph.15746
- Grottoli, S., Giordano, R., Maccagno, B., Pellegrino, M., Ghigo, E., & Arvat, E. (2002). The stimulatory effect of canrenoate, a mineralocorticoid antagonist, on the activity of the hypothalamus-pituitary-adrenal axis is abolished by alprazolam, a benzodiazepine, in humans. The Journal of Clinical Endocrinology and Metabolism, 87(10), 4616–4620. https://doi.org/10.1210/jc.2002-020331
- Gulyaeva, N. V. (2021). Glucocorticoid Regulation of the GlutamatergicSynapse: Mechanisms of Stress-Dependent Neuroplasticity. Journal of Evolutionary Biochemistry and Physiology, 57(3), 564–576. https://doi.org/10.1134/S0022093021030091
- Gupta, A. (2019). Role of stress and hormones of the Hypothalamic-Pituitary-Adrenal (HPA) axis in aging. In P. C. Rath (Ed.), Models, molecules and mechanisms in biogerontology: physiological abnormalities, diseases and interventions (pp. 241–263). Springer. https://doi.org/10.1007/978-981-13-3585-3
- Haleem, D. J., & Gul, S. (2020). Circulating leptin, cortisol and gender differences associated with anorexia or obesity in depression. The World Journal of Biological Psychiatry: The Official Journal of the World Federation of Societies of Biological Psychiatry, 21(3), 195–202. https://doi.org/10.1080/15622975.2019.1648870
- Hassan, A. H., von Rosenstiel, P., Patchev, V. K., Holsboer, F., & Almeida, O. F. (1996). Exacerbation of apoptosis in the dentate gyrus of the aged rat by dexamethasone and the protective role of corticosterone. Experimental Neurology, 140(1), 43–52. https://doi.org/10.1006/exnr.1996.0113
- Hayashi, G., & Cortopassi, G. (2015). Oxidative stress in inherited mitochondrial diseases. Free Radical Biology & Medicine, 88(Pt A), 10–17. https://doi.org/10.1016/j.freeradbiomed.2015.05.039
- Heim, C., Newport, D. J., Heit, S., Graham, Y. P., Wilcox, M., Bonsall, R., Miller, A. H., & Nemeroff, C. B. (2000). Pituitary-adrenal and autonomic responses to stress in women after sexual and physical abuse in childhood. JAMA, 284(5), 592–597. https://doi.org/10.1001/jama.284.5.592
- Herman, J. P., McKlveen, J. M., Ghosal, S., Kopp, B., Wulsin, A., Makinson, R., Scheimann, J., & Myers, B. (2016). Regulation of the hypothalamic-pituitary-adrenocortical stress response. Comprehensive Physiology, 6(2), 603–621. https://doi.org/10.1016/0006-8993(95)00152-g
- Herman, J. P., & Watson, S. J. (1995). Stress regulation of mineralocorticoid receptor heteronuclear RNA in rat hippocampus. Brain Research, 677(2), 243–249. https://doi.org/10.1016/0006-8993(95)00152-g
- Hermidorff, M. M., de Assis, L. V. M., & Isoldi, M. C. (2017). Genomic and rapid effects of aldosterone: What we know and do not know thus far. Heart Failure Reviews, 22(1), 65–89. https://doi.org/10.1007/s10741-016-9591-2
- Hlavacova, N., & Jezova, D. (2008). Chronic treatment with the mineralocorticoid hormone aldosterone results in increased anxiety-like behavior. Hormones and Behavior, 54(1), 90–97. https://doi.org/10.1016/j.yhbeh.2008.02.004
- Hlavacova, N., Wes, P. D., Ondrejcakova, M., Flynn, M. E., Poundstone, P. K., Babic, S., Murck, H., & Jezova, D. (2012). Subchronic treatment with aldosterone induces depression-like behaviours and gene expression changes relevant to major depressive disorder. The International Journal of Neuropsychopharmacology, 15(2), 247–265. https://doi.org/10.1017/S1461145711000368
- Hood, S., & Amir, S. (2017). The aging clock: Circadian rhythms and later life. The Journal of Clinical Investigation, 127(2), 437–446. https://doi.org/10.1172/JCI90328
- Hudson, W. H., Youn, C., & Ortlund, E. A. (2014). Crystal structure of the mineralocorticoid receptor DNA binding domain in complex with DNA. PloS One, 9(9), e107000. https://doi.org/10.1371/journal.pone.0107000
- Izakova, L., Hlavacova, N., Segeda, V., Kapsdorfer, D., Morovicsova, E., & Jezova, D. (2020). Salivary aldosterone, cortisol, and their morning to evening slopes in patients with depressive disorder and healthy subjects: acute episode and follow-up 6 months after reaching remission. Neuroendocrinology, 110(11-12), 1001–1009. https://doi.org/10.1159/000505921
- Joëls, M. (2001). Corticosteroid actions in the hippocampus: Corticosteroid actions in the hippocampus. Journal of Neuroendocrinology, 13(8), 657–669. https://doi.org/10.1046/j.1365-2826.2001.00688.x
- Joëls, M. (2008). Functional actions of corticosteroids in the hippocampus. European Journal of Pharmacology, 583(2-3), 312–321. https://doi.org/10.1016/j.ejphar.2007.11.064
- Joëls, M., Sarabdjitsingh, R. A., & Karst, H. (2012). Unraveling the time domains of corticosteroid hormone influences on brain activity: Rapid, slow, and chronic modes. Pharmacological Reviews, 64(4), 901–938. https://doi.org/10.1124/pr.112.005892
- Jurkowski, M. P., Bettio, L., K Woo, E., Patten, A., Yau, S.-Y., & Gil-Mohapel, J. (2020). Beyond the hippocampus and the SVZ: adult neurogenesis throughout the brain. Frontiers in Cellular Neuroscience, 14, 576444. https://doi.org/10.3389/fncel.2020.576444
- Juruena, M. F., Bocharova, M., Agustini, B., & Young, A. H. (2018). Atypical depression and non-atypical depression: Is HPA axis function a biomarker? A systematic review. Journal of Affective Disorders, 233, 45–67. https://doi.org/10.1016/j.jad.2017.09.052
- Juruena, M. F., Gama, C. S., Berk, M., & Belmonte-de-Abreu, P. S. (2009). Improved stress response in bipolar affective disorder with adjunctive spironolactone (mineralocorticoid receptor antagonist): Case series. Journal of Psychopharmacology (Oxford, England), 23(8), 985–987. https://doi.org/10.1177/0269881108092121
- Karandrea, D., Kittas, C., & Kitraki, E. (2000). Contribution of sex and cellular context in the regulation of brain corticosteroid receptors following restraint stress. Neuroendocrinology, 71(6), 343–353. https://doi.org/10.1159/000054555
- Karst, H., Berger, S., Turiault, M., Tronche, F., Schütz, G., & Joëls, M. (2005). Mineralocorticoid receptors are indispensable for nongenomic modulation of hippocampal glutamate transmission by corticosterone. Proceedings of the National Academy of Sciences of the United States of America, 102(52), 19204–19207. https://doi.org/10.1073/pnas.0507572102
- Karst, H., den Boon, F. S., Vervoort, N., Adrian, M., Kapitein, L. C., & Joëls, M. (2022). Non-genomic steroid signaling through the mineralocorticoid receptor: Involvement of a membrane-associated receptor? Molecular and Cellular Endocrinology, 541, 111501. https://doi.org/10.1016/j.mce.2021.111501
- Keller, J., Gomez, R., Williams, G., Lembke, A., Lazzeroni, L., Murphy, G. M., & Schatzberg, A. F. (2017). HPA axis in major depression: cortisol, clinical symptomatology, and genetic variation predict cognition. Molecular Psychiatry, 22(4), 527–536. https://doi.org/10.1038/mp.2016.120
- Keller-Wood, M. E., & Dallman, M. F. (1984). Corticosteroid inhibition of ACTH secretion. Endocrine Reviews, 5(1), 1–24. https://doi.org/10.1210/edrv-5-1-1
- Kellner, M., Baker, D. G., Yassouridis, A., Bettinger, S., Otte, C., Naber, D., & Wiedemann, K. (2002). Mineralocorticoid receptor function in patients with posttraumatic stress disorder. The American Journal of Psychiatry, 159(11), 1938–1940. https://doi.org/10.1176/appi.ajp.159.11.1938
- Kellner, M., Otte, C., Yassouridis, A., Schick, M., Jahn, H., & Wiedemann, K. (2004). Overnight metyrapone and combined dexamethasone/metyrapone tests in post-traumatic stress disorder: Preliminary findings. European Neuropsychopharmacology : The Journal of the European College of Neuropsychopharmacology, 14(4), 337–339. https://doi.org/10.1016/j.euroneuro.2003.11.002
- Kellner, M., & Wiedemann, K. (2008). Mineralocorticoid receptors in brain, in health and disease: Possibilities for new pharmacotherapy. European Journal of Pharmacology, 583(2-3), 372–378. https://doi.org/10.1016/j.ejphar.2007.07.072
- Kempermann, G., Song, H., & Gage, F. H. (2015). Neurogenesis in the Adult Hippocampus. Cold Spring Harbor Perspectives in Biology, 7(9), a018812. https://doi.org/10.1101/cshperspect.a018812
- Kennedy, C. L. M., Carter, S. D., Mifsud, K. R., & Reul, J. M. H. M. (2020). Unexpected effects of metyrapone on corticosteroid receptor interaction with the genome and subsequent gene transcription in the hippocampus of male rats. Journal of Neuroendocrinology, 32(2), e12820. https://doi.org/10.1111/jne.12820
- Kitraki, E., Kremmyda, O., Youlatos, D., Alexis, M. N., & Kittas, C. (2004). Gender-dependent alterations in corticosteroid receptor status and spatial performance following 21 days of restraint stress. Neuroscience, 125(1), 47–55. https://doi.org/10.1016/j.neuroscience.2003.12.024
- Kline, S. A., & Mega, M. S. (2020). Stress-Induced neurodegeneration: the potential for coping as neuroprotective therapy. American Journal of Alzheimer’s Disease and Other Dementias, 35, 1533317520960873. https://doi.org/10.1177/1533317520960873
- Kloet, E. R. D., Vreugdenhil, E., Oitzl, M. S., & Ls, M. J. (1998). Brain corticosteroid receptor balance in health and disease. Brain Corticosteroid Receptors, 19(3), 33.
- Klok, M. D., Alt, S. R., Irurzun Lafitte, A. J. M., Turner, J. D., Lakke, E. A. J. F., Huitinga, I., Muller, C. P., Zitman, F. G., Ronald de Kloet, E., & DeRijk, R. H. (2011a). Decreased expression of mineralocorticoid receptor mRNA and its splice variants in postmortem brain regions of patients with major depressive disorder. Journal of Psychiatric Research, 45(7), 871–878. https://doi.org/10.1016/j.jpsychires.2010.12.002
- Klok, M. D., Giltay, E. J., Van der Does, A. J. W., Geleijnse, J. M., Antypa, N., Penninx, B. W. J. H., de Geus, E. J. C., Willemsen, G., Boomsma, D. I., van Leeuwen, N., Zitman, F. G., de Kloet, E. R., & DeRijk, R. H. (2011b). A common and functional mineralocorticoid receptor haplotype enhances optimism and protects against depression in females. Translational Psychiatry, 1(12), e62. https://doi.org/10.1038/tp.2011.59
- Knutti, D., Kaul, A., & Kralli, A. (2000). A tissue-specific coactivator of steroid receptors, identified in a functional genetic screen. Molecular and Cellular Biology, 20(7), 2411–2422. https://doi.org/10.1128/MCB.20.7.2411-2422.2000
- Koning, A.-S C. A. M., Buurstede, J. C., van Weert, L. T. C. M., & Meijer, O. C. (2019). Glucocorticoid and mineralocorticoid receptors in the brain: a transcriptional perspective. Journal of the Endocrine Society, 3(10), 1917–1930. https://doi.org/10.1210/js.2019-00158
- Kuningas, M., de Rijk, R. H., Westendorp, R. G. J., Jolles, J., Slagboom, P. E., & van Heemst, D. (2007). Mental performance in old age dependent on cortisol and genetic variance in the mineralocorticoid and glucocorticoid receptors. Neuropsychopharmacology : official Publication of the American College of Neuropsychopharmacology, 32(6), 1295–1301. https://doi.org/10.1038/sj.npp.1301260
- Kwak, S. P., Patel, P. D., Thompson, R. C., Akil, H., & Watson, S. J. (1993). 5’-Heterogeneity of the mineralocorticoid receptor messenger ribonucleic acid: Differential expression and regulation of splice variants within the rat hippocampus. Endocrinology, 133(5), 2344–2350. https://doi.org/10.1210/endo.133.5.8404687
- Lai, M., Horsburgh, K., Bae, S.-E., Carter, R. N., Stenvers, D. J., Fowler, J. H., Yau, J. L., Gomez-Sanchez, C. E., Holmes, M. C., Kenyon, C. J., Seckl, J. R., & Macleod, M. R. (2007). Forebrain mineralocorticoid receptor overexpression enhances memory, reduces anxiety and attenuates neuronal loss in cerebral ischaemia: Increased mineralocorticoid receptor is protective. The European Journal of Neuroscience, 25(6), 1832–1842. https://doi.org/10.1111/j.1460-9568.2007.05427.x
- Lainscak, M., Pelliccia, F., Rosano, G., Vitale, C., Schiariti, M., Greco, C., Speziale, G., & Gaudio, C. (2015). Safety profile of mineralocorticoid receptor antagonists: Spironolactone and eplerenone. International Journal of Cardiology, 200, 25–29. https://doi.org/10.1016/j.ijcard.2015.05.127
- Le Moëllic, C., Ouvrard-Pascaud, A., Capurro, C., Cluzeaud, F., Fay, M., Jaisser, F., Farman, N., & Blot-Chabaud, M. (2004). Early nongenomic events in aldosterone action in renal collecting duct cells: PKCalpha activation, mineralocorticoid receptor phosphorylation, and cross-talk with the genomic response. Journal of the American Society of Nephrology: JASN, 15(5), 1145–1160.
- Liu, Z., Wong, J., Tsai, S. Y., Tsai, M. J., & O’Malley, B. W. (1999). Steroid receptor coactivator-1 (SRC-1) enhances ligand-dependent and receptor-dependent cell-free transcription of chromatin. Proceedings of the National Academy of Sciences of the United States of America, 96(17), 9485–9490. https://doi.org/10.1073/pnas.96.17.9485
- Lombès, M., Binart, N., Delahaye, F., Baulieu, E. E., & Rafestin-Oblin, M. E. (1994). Differential intracellular localization of human mineralocorticosteroid receptor on binding of agonists and antagonists. Biochemical Journal, 302(1), 191–197. https://doi.org/10.1042/bj3020191
- Lupien, S. J., Juster, R.-P., Raymond, C., & Marin, M.-F. (2018). The effects of chronic stress on the human brain: From neurotoxicity, to vulnerability, to opportunity. Frontiers in Neuroendocrinology, 49, 91–105. https://doi.org/10.1016/j.yfrne.2018.02.001
- Lupien, S. J., & McEwen, B. S. (1997). The acute effects of corticosteroids on cognition: Integration of animal and human model studies. Brain Research. Brain Research Reviews, 24(1), 1–27. https://doi.org/10.1016/s0165-0173(97)00004-0
- Mangelsdorf, D. J., Thummel, C., Beato, M., Herrlich, P., Schütz, G., Umesono, K., Blumberg, B., Kastner, P., Mark, M., Chambon, P., & Evans, R. M. (1995). The Nuclear Receptor Superfamily: The Second Decade. Cell, 83(6), 835–839. https://doi.org/10.1016/0092-8674(95)90199-x
- Marver, D., Stewart, J., Funder, J. W., Feldman, D., & Edelman, I. S. (1974). Renal Aldosterone Receptors: Studies with [3H]Aldosterone and the Anti-Mineralocorticoid [3H]Spirolactone (SC-26304). Proceedings of the National Academy of Sciences of the United States of America, 71(4), 1431–1435. https://doi.org/10.1073/pnas.71.4.1431
- Marzolla, V., Armani, A., Feraco, A., De Martino, M. U., Fabbri, A., Rosano, G., & Caprio, M. (2014). Mineralocorticoid receptor in adipocytes and macrophages: A promising target to fight metabolic syndrome. Steroids, 91, 46–53. https://doi.org/10.1016/j.steroids.2014.05.001
- McEown, K., & Treit, D. (2011). Mineralocorticoid receptors in the medial prefrontal cortex and hippocampus mediate rats’ unconditioned fear behaviour. Hormones and Behavior, 60(5), 581–588. https://doi.org/10.1016/j.yhbeh.2011.08.007
- McKenna, N. J., Lanz, R. B., & O’Malley, B. W. (1999). Nuclear receptor coregulators: Cellular and molecular biology. Endocrine Reviews, 20(3), 321–344. https://doi.org/10.1210/edrv.20.3.0366
- Medina, A., Seasholtz, A. F., Sharma, V., Burke, S., Bunney, W., Myers, R. M., Schatzberg, A., Akil, H., & Watson, S. J. (2013). Glucocorticoid and Mineralocorticoid Receptor Expression in the Human Hippocampus in Major Depressive Disorder. Journal of Psychiatric Research, 47(3), 307–314. https://doi.org/10.1016/j.jpsychires.2012.11.002
- Meijer, O. C., Koorneef, L. L., & Kroon, J. (2018). Glucocorticoid receptor modulators. Annales D’endocrinologie, 79(3), 107–111. https://doi.org/10.1016/j.ando.2018.03.004
- Meijer, O. C., Steenbergen, P. J., & de Kloet, E. R. (2000). Differential expression and regional distribution of steroid receptor coactivators SRC-1 and SRC-2 in brain and pituitary*. Endocrinology, 141(6), 2192–2199. https://doi.org/10.1210/endo.141.6.7489
- Meltzer, H. (1989). Serotonergic dysfunction in depression. British Journal of Psychiatry, 155(S8), 25–31. https://doi.org/10.1192/S0007125000291733
- Meltzer, H. Y. (1990). Role of serotonin in depressiona. Annals of the New York Academy of Sciences, 600(1), 486–499. https://doi.org/10.1111/j.1749-6632.1990.tb16904.x
- Mifsud, K. R., & Reul, J. M. H. M. (2018). Mineralocorticoid and glucocorticoid receptor-mediated control of genomic responses to stress in the brain. Stress (Amsterdam, Netherlands), 21(5), 389–402. https://doi.org/10.1080/10253890.2018.1456526
- Miller, M. W., & Sadeh, N. (2014). Traumatic stress, oxidative stress and post-traumatic stress disorder: Neurodegeneration and the accelerated-aging hypothesis. Molecular Psychiatry, 19(11), 1156–1162. https://doi.org/10.1038/mp.2014.111
- Mitra, R., Ferguson, D., & Sapolsky, R. M. (2009). Mineralocorticoid receptor overexpression in basolateral amygdala reduces corticosterone secretion and anxiety. Biological Psychiatry, 66(7), 686–690. https://doi.org/10.1016/j.biopsych.2009.04.016
- Montaron, M. F., Piazza, P. V., Aurousseau, C., Urani, A., Le Moal, M., & Abrous, D. N. (2003). Implication of corticosteroid receptors in the regulation of hippocampal structural plasticity. The European Journal of Neuroscience, 18(11), 3105–3111. https://doi.org/10.1111/j.1460-9568.2003.03048.x
- Morrison, N., Harrap, S., Arriza, J., Boyd, E., & Connor, J. M. (1990). Regional chromosomal assignment of the human mineralocorticoid receptor gene to 4q31.1. Human Genetics, 85(1), 130–132. https://doi.org/10.1007/BF00276340
- Motivala, A., & Pitt, B. (2007). Drospirenone for oral contraception and hormone replacement therapy: Are its cardiovascular risks and benefits the same as other progestogens? Drugs, 67(5), 647–655. https://doi.org/10.2165/00003495-200767050-00001
- Muhtz, C., Zyriax, B.-C., Bondy, B., Windler, E., & Otte, C. (2011). Association of a common mineralocorticoid receptor gene polymorphism with salivary cortisol in healthy adults. Psychoneuroendocrinology, 36(2), 298–301. https://doi.org/10.1016/j.psyneuen.2010.08.003
- Murck, H., Adolf, C., Schneider, A., Schlageter, L., Heinrich, D., Ritzel, K., Sturm, L., Quinkler, M., Beuschlein, F., Reincke, M., & Künzel, H. (2021). Differential effects of reduced mineralocorticoid receptor activation by unilateral adrenalectomy vs mineralocorticoid antagonist treatment in patients with primary aldosteronism—Implications for depression and anxiety. Journal of Psychiatric Research, 137, 376–382. https://doi.org/10.1016/j.jpsychires.2021.02.064
- Murck, H., Büttner, M., Kircher, T., & Konrad, C. (2014). Genetic, molecular and clinical determinants for the involvement of aldosterone and its receptors in major depression. Nephron. Physiology, 128(1-2), 17–25. https://doi.org/10.1159/000368265
- Myers, B., McKlveen, J. M., & Herman, J. P. (2014). Glucocorticoid actions on synapses, circuits, and behavior: Implications for the energetics of stress. Frontiers in Neuroendocrinology, 35(2), 180–196. https://doi.org/10.1016/j.yfrne.2013.12.003
- Nagata, Y., Goto, T., & Miyamoto, H. (2019). The role of mineralocorticoid receptor signaling in genitourinary cancers. Nuclear Receptor Research, 6, https://doi.org/10.32527/2019/101410
- Nakamura, T., Girerd, S., Jaisser, F., & Barrera-Chimal, J. (2022). Nonepithelial mineralocorticoid receptor activation as a determinant of kidney disease. Kidney International Supplements, 12(1), 12–18. https://doi.org/10.1016/j.kisu.2021.11.004
- Neylan, T. C., Lenoci, M., Maglione, M. L., Rosenlicht, N. Z., Metzler, T. J., Otte, C., Schoenfeld, F. B., Yehuda, R., & Marmar, C. R. (2003). Delta sleep response to metyrapone in post-traumatic stress disorder. Neuropsychopharmacology: official Publication of the American College of Neuropsychopharmacology, 28(9), 1666–1676. https://doi.org/10.1038/sj.npp.1300215
- Nicolaides, N. C., Kino, T., Roberts, M. L., Katsantoni, E., Sertedaki, A., Moutsatsou, P., Psarra, A.-M G., Chrousos, G. P., & Charmandari, E. (2017). The role of S-palmitoylation of the Human Glucocorticoid Receptor (hGR) in mediating the nongenomic glucocorticoid actions. Journal of Molecular Biochemistry, 6(1), 3–12. https://www.ncbi.nlm.nih.gov/pmc/articles/PMC5538142/
- Odermatt, A., & Atanasov, A. G. (2009). Mineralocorticoid receptors: Emerging complexity and functional diversity. Steroids, 74(2), 163–171. https://doi.org/10.1016/j.steroids.2008.10.010
- Odermatt, A., & Kratschmar, D. V. (2012). Tissue-specific modulation of mineralocorticoid receptor function by 11β-hydroxysteroid dehydrogenases: An overview. Molecular and Cellular Endocrinology, 350(2), 168–186. https://doi.org/10.1016/j.mce.2011.07.020
- Oh, H.-J., Song, M., Kim, Y. K., Bae, J. R., Cha, S.-Y., Bae, J. Y., Kim, Y., You, M., Lee, Y., Shim, J., & Maeng, S. (2018). Age-related decrease in stress responsiveness and proactive coping in male mice. Frontiers in Aging Neuroscience, 10, 128. https://doi.org/10.3389/fnagi.2018.00128
- Oitzl, M. S., & de Kloet, E. R. (1992). Selective corticosteroid antagonists modulate specific aspects of spatial orientation learning. Behavioral Neuroscience, 106(1), 62–71. https://doi.org/10.1037//0735-7044.106.1.62
- Oitzl, M. S., van Haarst, A. D., Sutanto, W., & de Kloet, E. R. (1995). Corticosterone, brain mineralocorticoid receptors (MRs) and the activity of the hypothalamic-pituitary-adrenal (HPA) axis: The Lewis rat as an example of increased central MR capacity and a hyporesponsive HPA axis. Psychoneuroendocrinology, 20(6), 655–675. https://doi.org/10.1016/0306-4530(95)00003-7
- Olijslagers, J. E., de Kloet, E. R., Elgersma, Y., van Woerden, G. M., Joëls, M., & Karst, H. (2008). Rapid changes in hippocampal CA1 pyramidal cell function via pre- as well as postsynaptic membrane mineralocorticoid receptors. The European Journal of Neuroscience, 27(10), 2542–2550. https://doi.org/10.1111/j.1460-9568.2008.06220.x
- Ong, G. S. Y., & Young, M. J. (2017). Mineralocorticoid regulation of cell function: The role of rapid signalling and gene transcription pathways. Journal of Molecular Endocrinology, 58(1), R33–R57. https://doi.org/10.1530/JME-15-0318
- Oster, H., Challet, E., Ott, V., Arvat, E., de Kloet, E. R., Dijk, D.-J., Lightman, S., Vgontzas, A., & Van Cauter, E. (2017). The functional and clinical significance of the 24-hour rhythm of circulating glucocorticoids. Endocrine Reviews, 38(1), 3–45. https://doi.org/10.1210/er.2015-1080
- Otte, C., Hinkelmann, K., Moritz, S., Yassouridis, A., Jahn, H., Wiedemann, K., & Kellner, M. (2010). Modulation of the mineralocorticoid receptor as add-on treatment in depression: A randomized, double-blind, placebo-controlled proof-of-concept study. Journal of Psychiatric Research, 44(6), 339–346. https://doi.org/10.1016/j.jpsychires.2009.10.006
- Otte, C., Muhtz, C., Daneshkhah, S., Yassouridis, A., Kiefer, F., Wiedemann, K., & Kellner, M. (2006). Mineralocorticoid receptor function in posttraumatic stress disorder after pretreatment with metyrapone. Biological Psychiatry, 60(7), 784–787. https://doi.org/10.1016/j.biopsych.2006.01.014
- Otte, C., Wingenfeld, K., Kuehl, L. K., Richter, S., Regen, F., Piber, D., & Hinkelmann, K. (2015). Cognitive function in older adults with major depression: Effects of mineralocorticoid receptor stimulation. Journal of Psychiatric Research, 69, 120–125. https://doi.org/10.1016/j.jpsychires.2015.08.001
- Otte, C., Wingenfeld, K., Kuehl, L. K., Kaczmarczyk, M., Richter, S., Quante, A., Regen, F., Bajbouj, M., Zimmermann-Viehoff, F., Wiedemann, K., & Hinkelmann, K. (2015). Mineralocorticoid receptor stimulation improves cognitive function and decreases cortisol secretion in depressed patients and healthy individuals. Neuropsychopharmacology, 40(2), 386–393. https://doi.org/10.1038/npp.2014.181
- Ozacmak, H. S., Ozacmak, V. H., Barut, F., Araslı, M., & Ucan, B. H. (2014). Pretreatment with mineralocorticoid receptor blocker reduces intestinal injury induced by ischemia and reperfusion: Involvement of inhibition of inflammatory response, oxidative stress, nuclear factor κB, and inducible nitric oxide synthase. The Journal of Surgical Research, 191(2), 350–361. https://doi.org/10.1016/j.jss.2014.04.040
- Parker, B. M., Wertz, S. L., Pollard, C. M., Desimine, V. L., Maning, J., McCrink, K. A., & Lymperopoulos, A. (2018). Novel insights into the crosstalk between mineralocorticoid receptor and G protein-coupled receptors in heart adverse remodeling and disease. International Journal of Molecular Sciences, 19(12), 3764. https://doi.org/10.3390/ijms19123764
- Pascual-Le Tallec, L., & Lombès, M. (2005). The mineralocorticoid receptor: A journey exploring its diversity and specificity of action. Molecular Endocrinology (Baltimore, Md.), 19(9), 2211–2221. https://doi.org/10.1210/me.2005-0089
- Patel, P. D., Lopez, J. F., Lyons, D. M., Burke, S., Wallace, M., & Schatzberg, A. F. (2000). Glucocorticoid and mineralocorticoid receptor mRNA expression in squirrel monkey brain. Journal of Psychiatric Research, 34(6), 383–392. https://doi.org/10.1016/s0022-3956(00)00035-2
- Patel, P. D., Sherman, T. G., Goldman, D. J., & Watson, S. J. (1989). Molecular cloning of a mineralocorticoid (type I) receptor complementary DNA from rat hippocampus. Molecular Endocrinology (Baltimore, Md.), 3(11), 1877–1885. https://doi.org/10.1210/mend-3-11-1877
- Paul, S. N., Wingenfeld, K., Otte, C., & Meijer, O. C. (2022). Brain mineralocorticoid receptor in health and disease: From molecular signalling to cognitive and emotional function. British Journal of Pharmacology, 179(13), 3205–3219. https://doi.org/10.1111/bph.15835
- Penning, T. M. (2003). Hydroxysteroid dehydrogenases and pre-receptor regulation of steroid hormone action. Human Reproduction Update, 9(3), 193–205. https://doi.org/10.1093/humupd/dmg022
- Penning, T. M., Jin, Y., Rizner, T. L., & Bauman, D. R. (2008). Pre-receptor regulation of the androgen receptor. Molecular and Cellular Endocrinology, 281(1-2), 1–8. https://doi.org/10.1016/j.mce.2007.10.008
- Ponti, G., Obernier, K., & Alvarez-Buylla, A. (2013). Lineage progression from stem cells to new neurons in the adult brain ventricular-subventricular zone. Cell Cycle (Georgetown, Tex.), 12(11), 1649–1650. https://doi.org/10.4161/cc.24984
- Popoli, M., Yan, Z., McEwen, B. S., & Sanacora, G. (2011). The stressed synapse: The impact of stress and glucocorticoids on glutamate transmission. Nature Reviews. Neuroscience, 13(1), 22–37. https://doi.org/10.1038/nrn3138
- Qi, X.-R., Kamphuis, W., Wang, S., Wang, Q., Lucassen, P. J., Zhou, J.-N., & Swaab, D. F. (2013). Aberrant stress hormone receptor balance in the human prefrontal cortex and hypothalamic paraventricular nucleus of depressed patients. Psychoneuroendocrinology, 38(6), 863–870. https://doi.org/10.1016/j.psyneuen.2012.09.014
- Qing, L., Liu, L., Zhou, L., Zhang, F., Gao, C., Hu, L., & Nie, S. (2020). Sex-dependent association of mineralocorticoid receptor gene (NR3C2) DNA methylation and schizophrenia. Psychiatry Research, 292, 113318. https://doi.org/10.1016/j.psychres.2020.113318
- Qiu, S., Champagne, D. L., Peters, M., Catania, E. H., Weeber, E. J., Levitt, P., & Pimenta, A. F. (2010). Loss of limbic system-associated membrane protein leads to reduced hippocampal mineralocorticoid receptor expression, impaired synaptic plasticity, and spatial memory deficit. Biological Psychiatry, 68(2), 197–204. https://doi.org/10.1016/j.biopsych.2010.02.013
- Quevedo, J., Carvalho, A. F., & Zarate, C. A. (2019). Neurobiology of Depression: Road to Novel Therapeutics. Academic Press.
- Quinkler, M., Diederich, S., Bähr, V., & Oelkers, W. (2004). The role of progesterone metabolism and androgen synthesis in renal blood pressure regulation. Hormone and Metabolic Research = Hormon- Und Stoffwechselforschung = Hormones et Metabolisme, 36(6), 381–386. https://doi.org/10.1055/s-2004-814572
- Rao, R., & Androulakis, I. P. (2019). The physiological significance of the circadian dynamics of the HPA axis: Interplay between circadian rhythms, allostasis and stress resilience. Hormones and Behavior, 110, 77–89. https://doi.org/10.1016/j.yhbeh.2019.02.018
- Ravid, J. D., & Laffin, L. J. (2022). Effects of finerenone, a novel nonsteroidal mineralocorticoid receptor antagonist, on cardiovascular disease, chronic kidney disease, and blood pressure. Current Cardiology Reports, 24(10), 1251–1259. https://doi.org/10.1007/s11886-022-01750-0
- Reul, J. M. H. M., & de Kloet, E. R. (1985). Two receptor systems for corticosterone in rat brain: microdistribution and differential occupation. Endocrinology, 117(6), 2505–2511. https://doi.org/10.1210/endo-117-6-2505
- Reul, J. M. H. M., Gesing, A., Droste, S., Stec, I. S. M., Weber, A., Bachmann, C., Bilang-Bleuel, A., Holsboer, F., & Linthorst, A. C. E. (2000). The brain mineralocorticoid receptor: Greedy for ligand, mysterious in function. European Journal of Pharmacology, 405(1-3), 235–249. https://doi.org/10.1016/s0014-2999(00)00677-4
- Rivers, C., Flynn, A., Qian, X., Matthews, L., Lightman, S., Ray, D., & Norman, M. (2009). Characterization of conserved tandem donor sites and intronic motifs required for alternative splicing in corticosteroid receptor genes. Endocrinology, 150(11), 4958–4967. https://doi.org/10.1210/en.2009-0346
- Robertson, N. M., Schulman, G., Karnik, S., Alnemri, E., & Litwack, G. (1993). Demonstration of nuclear translocation of the mineralocorticoid receptor (MR) using an anti-MR antibody and confocal laser scanning microscopy. Molecular Endocrinology (Baltimore, Md.), 7(9), 1226–1239. https://doi.org/10.1210/mend.7.9.8247024
- Rodriguez, J. J., Montaron, M. F., Petry, K. G., Aurousseau, C., Marinelli, M., Premier, S., Rougon, G., Moal, M. L., & Abrous, D. N. (1998). Complex regulation of the expression of the polysialylated form of the neuronal cell adhesion molecule by glucocorticoids in the rat hippocampus. The European Journal of Neuroscience, 10(9), 2994–3006. https://doi.org/10.1046/j.1460-9568.1998.00316.x
- Roozendaal, B., Bohus, B., & McGaugh, J. L. (1996). Dose-dependent suppression of adrenocortical activity with metyrapone: Effects on emotion and memory. Psychoneuroendocrinology, 21(8), 681–693. https://doi.org/10.1016/s0306-4530(96)00028-5
- Roozendaal, B., McReynolds, J. R., Van der Zee, E. A., Lee, S., McGaugh, J. L., & McIntyre, C. K. (2009). Glucocorticoid effects on memory consolidation depend on functional interactions between the medial prefrontal cortex and basolateral amygdala. The Journal of Neuroscience : The Official Journal of the Society for Neuroscience, 29(45), 14299–14308. https://doi.org/10.1523/JNEUROSCI.3626-09.2009
- Rozeboom, A. M., Akil, H., & Seasholtz, A. F. (2007). Mineralocorticoid receptor overexpression in forebrain decreases anxiety-like behavior and alters the stress response in mice. Proceedings of the National Academy of Sciences of the United States of America, 104(11), 4688–4693. https://doi.org/10.1073/pnas.0606067104
- Ruhs, S., Nolze, A., Hübschmann, R., & Grossmann, C. (2017). 30 Years Of The Mineralocorticoid Receptor: Nongenomic effects via the mineralocorticoid receptor. Journal of Endocrinology, 234(1), T107–T124. https://doi.org/10.1530/JOE-16-0659
- Sajadi, A. A., Samaei, S. A., & Rashidy-Pour, A. (2006). Intra-hippocampal microinjections of anisomycin did not block glucocorticoid-induced impairment of memory retrieval in rats: An evidence for non-genomic effects of glucocorticoids. Behavioural Brain Research, 173(1), 158–162. https://doi.org/10.1016/j.bbr.2006.06.018
- Šalamon Arčan, I., Kouter, K., & Videtič Paska, A. (2022). Depressive disorder and antidepressants from an epigenetic point of view. World Journal of Psychiatry, 12(9), 1150–1168. https://doi.org/10.5498/wjp.v12.i9.1150
- Sarabdjitsingh, R. A., Joëls, M., & de Kloet, E. R. (2012). Glucocorticoid pulsatility and rapid corticosteroid actions in the central stress response. Physiology & Behavior, 106(1), 73–80. https://doi.org/10.1016/j.physbeh.2011.09.017
- Schumacher, M. (1990). Rapid membrane effects of steroid hormones: An emerging concept in neuroendocrinology. Trends in Neurosciences, 13(9), 359–362. https://doi.org/10.1016/0166-2236(90)90016-4
- Schwabe, L., Tegenthoff, M., Höffken, O., & Wolf, O. T. (2013). Mineralocorticoid receptor blockade prevents stress-induced modulation of multiple memory systems in the human brain. Biological Psychiatry, 74(11), 801–808. https://doi.org/10.1016/j.biopsych.2013.06.001
- Shea, A., Walsh, C., MacMillan, H., & Steiner, M. (2005). Child maltreatment and HPA axis dysregulation: Relationship to major depressive disorder and post traumatic stress disorder in females. Psychoneuroendocrinology, 30(2), 162–178. https://doi.org/10.1016/j.psyneuen.2004.07.001
- Shekhtman, E., Geerling, J. C., & Loewy, A. D. (2007). Aldosterone-sensitive neurons of the nucleus of the solitary tract: Multisynaptic pathway to the nucleus accumbens. The Journal of Comparative Neurology, 501(2), 274–289. https://doi.org/10.1002/cne.21245
- Sheppard, K. E. (2002). Nuclear receptors. II. Intestinal corticosteroid receptors. American Journal of Physiology. Gastrointestinal and Liver Physiology, 282(5), G742–746. https://doi.org/10.1152/ajpgi.00531.2001
- Smythe, J. W., Murphy, D., Timothy, C., & Costall, B. (1997). Hippocampal Mineralocorticoid, but Not Glucocorticoid, Receptors Modulate Anxiety-Like Behavior in Rats. Pharmacology, Biochemistry, and Behavior, 56(3), 507–513. https://doi.org/10.1016/s0091-3057(96)00244-4
- Sonino, N., Tomba, E., Genesia, M. L., Bertello, C., Mulatero, P., Veglio, F., Fava, G. A., & Fallo, F. (2011). Psychological assessment of primary aldosteronism: A controlled study. The Journal of Clinical Endocrinology and Metabolism, 96(6), E878–883. https://doi.org/10.1210/jc.2010-2723
- Sousa, N., Paula-Barbosa, M. M., & Almeida, O. F. (1999). Ligand and subfield specificity of corticoid-induced neuronal loss in the rat hippocampal formation. Neuroscience, 89(4), 1079–1087. https://doi.org/10.1016/s0306-4522(98)00311-x
- Spijker, A. T., & van Rossum, E. F. C. (2009). Glucocorticoid receptor polymorphisms in major depression. Focus on glucocorticoid sensitivity and neurocognitive functioning. Annals of the New York Academy of Sciences, 1179, 199–215. https://doi.org/10.1111/j.1749-6632.2009.04985.x
- Syed, S. B., & Qureshi, M. A. (2012). Association of aldosterone and cortisol with cardiovascular risk factors in prehypertension stage. International Journal of Hypertension, 2012, 906327. https://doi.org/10.1155/2012/906327
- ter Horst, J. P., Carobrez, A. P., van der Mark, M. H., de Kloet, E. R., & Oitzl, M. S. (2012). Sex differences in fear memory and extinction of mice with forebrain-specific disruption of the mineralocorticoid receptor. The European Journal of Neuroscience, 36(8), 3096–3102. https://doi.org/10.1111/j.1460-9568.2012.08237.x
- ter Horst, J. P., van der Mark, M. H., Arp, M., Berger, S., de Kloet, E. R., & Oitzl, M. S. (2012). Stress or no stress: Mineralocorticoid receptors in the forebrain regulate behavioral adaptation. Neurobiology of Learning and Memory, 98(1), 33–40. https://doi.org/10.1016/j.nlm.2012.04.006
- Torchia, J., Glass, C., & Rosenfeld, M. G. (1998). Co-activators and co-repressors in the integration of transcriptional responses. Current Opinion in Cell Biology, 10(3), 373–383. https://doi.org/10.1016/s0955-0674(98)80014-8
- van der Heijden, C. D. C. C., Bode, M., Riksen, N. P., & Wenzel, U. O. (2022). The role of the mineralocorticoid receptor in immune cells in cardiovascular disease. British Journal of Pharmacology, 179(13), 3135–3151. https://doi.org/10.1111/bph.15782
- van Haarst, A. D., Oitzl, M. S., & de Kloet, E. R. (1997). Facilitation of feedback inhibition through blockade of glucocorticoid receptors in the hippocampus. Neurochemical Research, 22(11), 1323–1328. https://doi.org/10.1023/a:1022010904600
- van Leeuwen, N., Kumsta, R., Entringer, S., de Kloet, E. R., Zitman, F. G., DeRijk, R. H., & Wüst, S. (2010). Functional mineralocorticoid receptor (MR) gene variation influences the cortisol awakening response after dexamethasone. Psychoneuroendocrinology, 35(3), 339–349. https://doi.org/10.1016/j.psyneuen.2009.07.006
- Viengchareun, S., Le Menuet, D., Martinerie, L., Munier, M., Tallec, L. P.-L., & Lombès, M. (2007). The Mineralocorticoid Receptor: Insights into its Molecular and (Patho)Physiological Biology. Nuclear Receptor Signaling, 5(1), nrs.05012. https://doi.org/10.1621/nrs.05012
- Viho, E. M. G., Buurstede, J. C., Berkhout, J. B., Mahfouz, A., & Meijer, O. C. (2022). Cell type specificity of glucocorticoid signaling in the adult mouse hippocampus. Journal of Neuroendocrinology, 34(2), e13072. https://doi.org/10.1111/jne.13072
- Vinkers, C. H., Joëls, M., Milaneschi, Y., Gerritsen, L., Kahn, R. S., Penninx, B. W. J. H., & Boks, M. P. M. (2015). Mineralocorticoid receptor haplotypes sex-dependently moderate depression susceptibility following childhood maltreatment. Psychoneuroendocrinology, 54, 90–102. https://doi.org/10.1016/j.psyneuen.2015.01.018
- Vogel, S., Klumpers, F., Schröder, T. N., Oplaat, K. T., Krugers, H. J., Oitzl, M. S., Joëls, M., Doeller, C. F., & Fernández, G. (2017). Stress induces a shift towards striatum-dependent stimulus-response learning via the mineralocorticoid receptor. Neuropsychopharmacology : official Publication of the American College of Neuropsychopharmacology, 42(6), 1262–1271. https://doi.org/10.1038/npp.2016.262
- Wang, C.-H., Hung, P.-W., Chiang, C.-W., Lombès, M., Chen, C.-H., Lee, K.-H., Lo, Y.-C., Wu, M.-H., Chang, W.-C., & Lin, D.-Y. (2019). Identification of two independent SUMO-interacting motifs in Fas-associated factor 1 (FAF1): Implications for mineralocorticoid receptor (MR)-mediated transcriptional regulation. Biochimica et Biophysica Acta. Molecular Cell Research, 1866(8), 1282–1297. https://doi.org/10.1016/j.bbamcr.2019.03.014
- Wang, Q., Anzick, S., Richter, W. F., Meltzer, P., & Simons, S. S. (2004). Modulation of transcriptional sensitivity of mineralocorticoid and estrogen receptors. The Journal of Steroid Biochemistry and Molecular Biology, 91(4-5), 197–210. https://doi.org/10.1016/j.jsbmb.2004.04.007
- Watzka, M., Beyenburg, S., Blümcke, I., Elger, C. E., Bidlingmaier, F., & Stoffel-Wagner, B. (2000). Expression of mineralocorticoid and glucocorticoid receptor mRNA in the human hippocampus. Neuroscience Letters, 290(2), 121–124. https://doi.org/10.1016/s0304-3940(00)01325-2
- Weber, M., Wehling, M., & Lösel, R. (2008). Proteins interact with the cytosolic mineralocorticoid receptor depending on the ligand. American Journal of Physiology. Heart and Circulatory Physiology, 295(1), H361–365. https://doi.org/10.1152/ajpheart.00825.2007
- Wehling, M. (2005). Effects of Aldosterone and Mineralocorticoid Receptor Blockade on Intracellular Electrolytes. Heart Failure Reviews, 10(1), 39–46. https://doi.org/10.1007/s10741-005-2347-z
- Wehr, M. C., Hinrichs, W., Brzózka, M. M., Unterbarnscheidt, T., Herholt, A., Wintgens, J. P., Papiol, S., Soto-Bernardini, M. C., Kravchenko, M., Zhang, M., Nave, K., Wichert, S. P., Falkai, P., Zhang, W., Schwab, M. H., & Rossner, M. J. (2017). Spironolactone is an antagonist of NRG1-ERBB4 signaling and schizophrenia-relevant endophenotypes in mice. EMBO Molecular Medicine, 9(10), 1448–1462. https://doi.org/10.15252/emmm.201707691
- Weikum, E. R., Liu, X., & Ortlund, E. A. (2018). The nuclear receptor superfamily: A structural perspective. Protein Science : a Publication of the Protein Society, 27(11), 1876–1892. https://doi.org/10.1002/pro.3496
- Wickert, L., Watzka, M., Bolkenius, U., Bidlingmaier, F., & Ludwig, M. (1998). Mineralocorticoid receptor splice variants in different human tissues. European Journal of Endocrinology, 138(6), 702–704. https://doi.org/10.1530/eje.0.1380702
- Wilson, P., Morgan, J., Funder, J. W., Fuller, P. J., & Young, M. J. (2009). Mediators of mineralocorticoid receptor-induced profibrotic inflammatory responses in the heart. Clinical Science (London, England : 1979), 116(9), 731–739. https://doi.org/10.1042/CS20080247
- Wingenfeld, K., Kuehl, L. K., Janke, K., Hinkelmann, K., Dziobek, I., Fleischer, J., Otte, C., & Roepke, S. (2014). Enhanced emotional empathy after mineralocorticoid receptor stimulation in women with borderline personality disorder and healthy women. Neuropsychopharmacology : official Publication of the American College of Neuropsychopharmacology, 39(8), 1799–1804. https://doi.org/10.1038/npp.2014.36
- Wingenfeld, K., & Otte, C. (2019). Mineralocorticoid receptor function and cognition in health and disease. Psychoneuroendocrinology, 105, 25–35. https://doi.org/10.1016/j.psyneuen.2018.09.010
- Wingenfeld, K., & Wolf, O. T. (2015). Effects of cortisol on cognition in major depressive disorder, posttraumatic stress disorder and borderline personality disorder—2014 Curt Richter Award Winner. Psychoneuroendocrinology, 51, 282–295. https://doi.org/10.1016/j.psyneuen.2014.10.009
- Wu, T.-C., Chen, H.-T., Chang, H.-Y., Yang, C.-Y., Hsiao, M.-C., Cheng, M.-L., & Chen, J.-C. (2013). Mineralocorticoid receptor antagonist spironolactone prevents chronic corticosterone induced depression-like behavior. Psychoneuroendocrinology, 38(6), 871–883. https://doi.org/10.1016/j.psyneuen.2012.09.011
- Xing, G.-Q., Russell, S., Webster, M. J., & Post, R. M. (2004). Decreased expression of mineralocorticoid receptor mRNA in the prefrontal cortex in schizophrenia and bipolar disorder. The International Journal of Neuropsychopharmacology, 7(2), 143–153. https://doi.org/10.1017/S1461145703004000
- Yang, J., & Young, M. J. (2009). The mineralocorticoid receptor and its coregulators. Journal of Molecular Endocrinology, 43(2), 53–64. https://doi.org/10.1677/JME-09-0031
- Yau, J. L., Noble, J., & Seckl, J. R. (1999). Continuous blockade of brain mineralocorticoid receptors impairs spatial learning in rats. Neuroscience Letters, 277(1), 45–48. https://doi.org/10.1016/s0304-3940(99)00858-7
- Yiallouris, A., Tsioutis, C., Agapidaki, E., Zafeiri, M., Agouridis, A. P., Ntourakis, D., & Johnson, E. O. (2019). Adrenal aging and its implications on stress responsiveness in humans. Frontiers in Endocrinology, 10, 54. https://doi.org/10.3389/fendo.2019.00054
- Yokota, K., Shibata, H., Kurihara, I., Kobayashi, S., Suda, N., Murai-Takeda, A., Saito, I., Kitagawa, H., Kato, S., Saruta, T., & Itoh, H. (2007). Coactivation of the N-terminal transactivation of mineralocorticoid receptor by Ubc9. The Journal of Biological Chemistry, 282(3), 1998–2010. https://doi.org/10.1074/jbc.M607741200
- Young, E. A., Haskett, R. F., Grunhaus, L., Pande, A., Weinberg, V. M., Watson, S. J., & Akil, H. (1994). Increased evening activation of the hypothalamic-pituitary-adrenal axis in depressed patients. Archives of General Psychiatry, 51(9), 701–707. https://doi.org/10.1001/archpsyc.1994.03950090033005
- Zajkowska, Z., Gullett, N., Walsh, A., Zonca, V., Pedersen, G. A., Souza, L., Kieling, C., Fisher, H. L., Kohrt, B. A., & Mondelli, V. (2022). Cortisol and development of depression in adolescence and young adulthood – a systematic review and meta-analysis. Psychoneuroendocrinology, 136, 105625. https://doi.org/10.1016/j.psyneuen.2021.105625
- Zandifar, A., Badrfam, R., Sanjari Moghaddam, H., & Akhondzadeh, S. (2022). Efficacy of spironolactone as an adjunctive therapy to risperidone to improve symptoms of schizophrenia: a double-blind, randomized, placebo-controlled, clinical trial. Iranian Journal of Psychiatry, 17(1), 14–23. https://doi.org/10.18502/ijps.v17i1.8045
- Zennaro, M.-C., Keightley, M.-C., Kotelevtsev, Y., Conway, G. S., Soubrier, F., & Fuller, P. J. (1995). Human mineralocorticoid receptor genomic structure and identification of expressed isoforms. The Journal of Biological Chemistry, 270(36), 21016–21020. https://doi.org/10.1074/jbc.270.36.21016
- Zhe, D., Fang, H., & Yuxiu, S. (2008). Expressions of hippocampal Mineralocorticoid Receptor (MR) and Glucocorticoid Receptor (GR) in the single-prolonged stress-rats. Acta Histochemica et Cytochemica, 41(4), 89–95. https://doi.org/10.1267/ahc.08013
- Zhou, M., Bakker, E. H. M., Velzing, E. H., Berger, S., Oitzl, M., Joëls, M., & Krugers, H. J. (2010). Both mineralocorticoid and glucocorticoid receptors regulate emotional memory in mice. Neurobiology of Learning and Memory, 94(4), 530–537. https://doi.org/10.1016/j.nlm.2010.09.005
- Zhou, M., Kindt, M., Joëls, M., & Krugers, H. J. (2011). Blocking mineralocorticoid receptors prior to retrieval reduces contextual fear memory in mice. PloS One, 6(10), e26220. https://doi.org/10.1371/journal.pone.0026220