Abstract
Glucocorticoid hormones are essential for health, but overexposure may lead to many detrimental effects, including metabolic, psychiatric, and bone disease. These effects may not only be due to increased overall exposure to glucocorticoids, but also to elevated hormone levels at the time of the physiological circadian trough of glucocorticoid levels. The late Mary Dallman developed a model that allows the differentiation between the effects of overall 24-hour glucocorticoid overexposure and the effects of a lack of circadian rhythmicity. For this, she continuously treated rats with a low dose of corticosterone (or “B”), which leads to a constant hormone level, without 24-hour overexposure using subcutaneously implanted pellets. The data from this “B-flat” model suggest that even modest elevations of glucocorticoid signaling during the time of the normal circadian trough of hormone secretion are a substantial contributor to the negative effects of glucocorticoids on health.
Introduction
In 1996, Mary Dallman embarked on a project to generate a mutant mouse in which the high-affinity receptor for the major stress hormone corticosterone, the mineralocorticoid receptor, would be always active. This project of Mary’s sabbatical in the lab of David Pearce at UCSF did not pan out, yet the project was a well-conceived attempt to answer a basic question on how glucocorticoid (GC) hormones control mammalian physiology. The project attests to Mary’s scientific mind, which was both creative and highly structured, and of course, was in large part dedicated to understanding the effects of GC hormones.
The adrenal GCs are powerful hormones that regulate the activity of every organ in the body. They are essential for the circadian alignment of organs and successful adaptation to stressors, yet overexposure to these hormones increases the risk for many, very different diseases. Appropriate regulation of glucocorticoid levels is therefore essential. Regulation of tissue exposure to GCs takes place in many ways, including hormone binding to plasma proteins (Henley et al., Citation2016), and enzymatic regulation of hormone concentrations in target tissues (Wyrwoll et al., Citation2011). Foremost, the regulation of glucocorticoids amounts to strict control over the hypothalamus pituitary adrenal (HPA) axis. The HPA-axis signaling cascade starts with corticotropin-releasing hormone (CRH) expressing neuroendocrine neurons in the hypothalamic paraventricular nucleus (PVN). These are controlled by a plethora of neuronal inputs (Herman et al., Citation2003). The CRH that is released from the brain acts on the adrenocorticotropic hormone (ACTH)-producing corticotropes in the anterior pituitary, which in turn activates the cells in the adrenal cortex that produce GC hormones. Negative feedback control by GCs takes place at the level of the PVN and the pituitary (Jiang et al., Citation2022; Kim & Iremonger, Citation2019), and possibly in the adrenocortical glucocorticoid producing cells (Walker et al., Citation2015). In addition, there are extra-hypothalamic feedback sites in the brain, such as the hippocampus (Ratka et al., Citation1989).
The adrenals of rats and mice exclusively produce corticosterone, or – as the older generation would say – “Kendall”s substance B’ (Heilman & Kendall, Citation1956). In contrast, the human adrenal cortex secretes predominantly cortisol (“substance F”), leading to a blood B:F ratio of about 1:20. B and F are largely interchangeable, although they differ as substrates for particular transporters. B has superior penetration of the blood-brain barrier, as F is a substrate for the P-glycoprotein (Schinkel et al., Citation1994). B may therefore account for up to 30% of the glucocorticoids in the human brain (Karssen et al., Citation2001). Conversely, B but not F is transported out of adipocytes through ABCC1, and this has been used as an argument for the use of B rather than F in human hormone replacement (Nixon et al., Citation2016).
In this review, we will focus on the importance of one particular aspect of GC control, namely the importance of a daily trough in adrenocortical secretion. The data that we discuss are largely based on a model that uses insights from receptor pharmacology of negative feedback as developed by Mary Dallman (Akana et al., Citation1992). And so as Mary once wrote about “Variations on a theme of B” (Dallman et al., Citation1987), this variation will be on B-flat.
Adaptive and pathogenic glucocorticoid exposure
GCs can turn from essential adaptive coordinators to contributors to disease development in several ways. In the good scenario, in the absence of stress, they follow a circadian pattern made up of shorter ultradian episodes of adrenocortical secretion (Lightman & Conway-Campbell, Citation2010; Upton et al., Citation2023). Hormone levels are low at the end of the active period (i.e. late evening in humans, early morning in rats and mice). Halfway through the resting phase, levels start to rise and peak around awakening. They steadily decline over the active period to reach a trough of low to even undetectable levels. In the presence of acute stressors, there is an additional adaptive activation of the HPA axis. Glucocorticoid concentrations peak 15–20 minutes after stress onset, and return to normal within 2 hours if the stressor has disappeared. In the face of sustained stressors, the HPA-axis is activated more chronically (Dallman et al., Citation1999), but usually still exhibits a circadian pattern (Dallman et al., Citation2000).
There are many ways in which the HPA axis adjusts to long-term activation, and what happens during chronic stress is difficult to fully capture. However, almost all situations in which glucocorticoids are thought to contribute to disease processes involve some form of prolonged exposure. Posttraumatic stress disorder (PTSD) is a possible exception to this, as it may develop also after (repeated) excessive exposure to acute stressors with transiently highly elevated cortisol, but in the chronic disease state possibly even hypocortisolemia (Pace & Heim, Citation2011). Cushing’s Disease, where the HPA axis is often overactive for years, leads to overt pathology involving many organ systems (Limumpornpetch et al., Citation2022). In situations of chronic stress, GC levels are not in the Cushing’s range, but are thought to also increase the risk e.g. metabolic and psychiatric disease. In a more subtle way, a slower recovery of the HPA-axis in acute stressors may be maladaptive, given that over time such responses can lead to a higher integrated exposure to glucocorticoids (Leeuwen et al., Citation2018). Of note, systemic synthetic glucocorticoid use mimics the effects of overexposure to endogenous GCs, depending on the dose and duration. Given that 1–3% of the Western population uses such therapy (Laugesen et al., Citation2019), this poses a health risk for many people.
Thus, “chronic overexposure” to GCs is associated with detrimental effects. The central question that we will address in this review, is the distinction between overall hypercortisolemia (“F- or B-sharp” in this context), and the lack of rhythmicity (“F- or B-flat”) that often also occurs in situations of enhanced HPA-axis activity. Our main message: perhaps it is not so much - or not only - the absolute GC exposure that matters for detrimental effects, but rather an appropriate pause in signaling during the circadian trough. An elegant model that Mary Dallman developed allows us to distinguish, in rodents, the consequences of rhythmicity from those of overexposure by implantation of pellets containing just the right amount of B.
The B-flat model
In itself, the notion of subcutaneous implants for the constant delivery of hormones that we adopted from Akana and Dallman is far from new and goes back to the origins of endocrinology. However, in the ”B-flat” model things are a bit more subtle () (Akana et al., Citation1992). A crucial aspect is that the adrenals can be left intact, and so there are no issues with the loss of adrenalin or aldosterone. This model is based on negative feedback: a moderately elevated B level at the time of the natural trough activates negative feedback on the HPA-axis, and as a consequence, the circadian peak is flattened to the extent that it no longer exists. At the same time, the total exposure to GC (i.e. area under the curve of the 24-hour B levels) is not significantly elevated, and biomarkers for chronic B overexposure such as thymus weight are not, or only modestly, affected. Simply increasing the dose of B in the pellet will lead to elevated 24-hour exposure, and mimic a more Cushing-like state. Thus, the effects of lack of rhythmicity per se and overexposure can be evaluated using highly similar treatments in adrenally intact animals.
Figure 1. The B-flat model. Compared to the physiological situation, there are two distinct ways to achieve a disturbed B-level via subcutaneous implantation of pellets. In a classic overexposure model, hormone levels are high and mimic Cushing’s disease, chronic treatment with synthetic steroids, and perhaps chronic severe stress. The consequences of such a regimen may be due to either overall GC over-exposure or to a lack of circadian rhythmicity. In the B-Flat model, the implanted pellet leads to increased trough levels that suffice to activate negative feedback, likely involving the MR. This leads to reduced adrenocortical output and a flattened rhythm in the absence of overall 24 hour higher exposure to hormones. Created with Biorender.com.
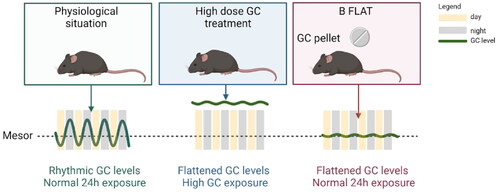
This B-flat model has been used both in mice and rats, and was shown to affect stress reactivity, serotonin receptor expression in the brain, adiposity and thermogenic activity, and osteoporosis (Akana et al., Citation1992; Kroon et al., Citation2021; Meijer et al., Citation1997; Sarabdjitsingh et al., Citation2010; Schilperoort et al., Citation2021; Tholen et al., Citation2022). Likely there are many other organ systems and processes that are adversely affected by a lack of GC rhythm (or lack of a GC trough), rather than overall GC concentrations over the day or elevations at the time of the circadian peak.
How does the model work?
Given that negative feedback is primarily conceptualized as the normalization of stimulated hormone levels, how would minor elevations activate a negative feedback mechanism? Here, it becomes relevant that B and F act via two receptor types, the high-affinity mineralocorticoid receptor (MR) and the lower-affinity glucocorticoid receptor (GR). The GR is expressed almost ubiquitously, including the major negative feedback sites of the HPA-axis: the neuroendocrine CRH neurons in the PVN and the ACTH-producing corticotropes in the pituitary. It is the GR that is thought to be the major receptor in the context of stress-activated HPA-axis activity (Ratka et al., Citation1989; Viho et al., Citation2022).
The MR in the brain is mainly a receptor for B and F rather than for the mineralocorticoid aldosterone and is much more restricted in its expression than the GR. The MR is expressed at very high levels in the hippocampus, and here it mediates a negative feedback system on the HPA-axis (Bradbury et al., Citation1991; Bradbury:1991vq; Reul et al., Citation1987; van Haarst et al., Citation1997). This MR-mediated signal was originally conceptualized to be “tonic” (that is: continuously active) throughout the 24-hour cycle (Reul et al., Citation1987). This was contested by Dallman, e.g. based on observations that trough B levels in rats are similar to those found in adrenalectomized animals. This may have been the reason why Dallman embarked on the attempt to generate a mouse line with a constitutively active MR, some 4 years after the B-flat model was published. Microdialysis that was done in rats later suggested that extended periods of undetectable B may indeed occur during the inactive period of the day, but in a strain/sex/state dependent manner (Windle et al., Citation2001). In the human brain, MR occupancy may be even lower than in the rodent, given that low concentrations of F do not have unhampered access to the brain (Karssen et al., Citation2001). Be this as may, Akana and Dallman hypothesized that enhanced B concentrations at the moment of the circadian trough would activate hippocampal MR-mediated feedback, and that this would account for the compensatory lowering of the circadian peak of B in rats.
B-flat: MR and GR overactivation
In the 1992 paper, Akana et al used 3 different doses of B-replacement in rats. The higher dose of 80 mg B per subcutaneous pellet clearly led to many GR-mediated changes, including increased insulin levels and reduced body weight gain. With 20 mg and 40 mg pellets the effects they measured mainly concerned “static” HPA-axis parameters such as adrenal weights and pituitary ACTH content, but also the corticosterone response to restraint stress was found to be convincingly reduced. These may all be effects of higher MR signaling. Indeed, thymus weight, which is a highly sensitive biomarker for GC excess acting via GR, was not or hardly affected by 40 mg pellets in rats (Akana et al., Citation1992; Sarabdjitsingh et al., Citation2010), or in the slightly lower dose of 35 mg (Meijer et al., Citation1997). However, it is formally difficult to prove that the 24-hour exposure to corticosterone is really not higher in this model, in particular because free corticosterone concentrations have not been determined. Nevertheless, any overexposure is very modest in relation to classical chronic corticosterone treatment models. This also goes for mice, where optimal doses to flatten the rhythm without 24-hour overexposure seem to be 2.5 mg and 7 mg corticosterone in males and females, respectively (Kroon et al., Citation2021; Schilperoort et al., Citation2021).
Although the B-flat model was based on MR-mediated feedback (Akana et al., Citation1992; Dallman et al., Citation1989), and later interpreted (Dallman et al., Citation2000; Meijer et al., Citation1997) as reflecting mainly enhanced MR rather than GR activation, this notion goes formally unproven. Overactivation of MR without overactivation of GR makes sense from a receptor occupancy point of view, as there is a ten-fold difference in affinity between the receptors (Reul et al., Citation1987). However, the potency of GR-mediated effects depends very much on the process. This is recapitulated at the transcriptional level, where the concentration-response curves of GR-dependent gene expression differ substantially per gene (Reddy et al., Citation2009). This is most extreme for the period 1 (PER1) gene (Reddy et al., Citation2012), and it in fact makes sense that circadian alignment should involve different transcriptional targets (like PER1) than a strong stress response (Meijer, Citation2006). Thus, GR-mediated effects occur at different hormone concentrations. Interestingly, this gene-specific potency of GR is also ligand-dependent, as evidenced by partial agonism properties of the classical GR antagonist RU486 (Havel et al., Citation1996). We found a reversed order of potency at a small set of genes for RU486 and the synthetic agonist dexamethasone (Monczor et al., Citation2019). The latter finding corroborates the notion that gene-specific GR potency is not only caused by intrinsic differences in affinity of the GR binding sites at the DNA (Polman et al., Citation2013), but also involves interactions with other transcription factors and/or transcriptional coregulators (Reddy et al., Citation2012).
One indirect argument for GR-mediated effects at “daily average” corticosterone levels comes from a later study by Akana and Dallman (Akana & Dallman, Citation1997). In a study in adrenalectomized male rats that received corticosterone pellets, a minimum plasma concentration of 2.3 µg/dl (or 23 ng/ml) was needed for animals to survive at 4 degrees centigrade. The survival likely reflects GR-mediated effects on energy recruitment, and this dose is well below the daily average of about 40-50 ng/ml in the plasma of male rats. Another argument for GR activation comes from clear effects of the B-flat regimen on direct transcriptional MR/GR targets in tissues that simply do not have a lot of MR expression.
An attractive mechanism for the substantial impact of flat B is that GR sensitivity may vary through the day: higher tissues responses at the moment of the normal circadian trough may account for more (detrimental) effects. One notion is that GR interacts with transcription factors that are part of the molecular circadian clock that is present in every cell (Cederroth et al., Citation2019). Specifically, GR interactions with the BMAL1/CLOCK complex have been reported to reduce binding affinity of GR for the DNA. This regulatory mechanism has been suggested to lead to an increased GR sensitivity at the moment of the natural trough of hormone concentrations (Nader et al., Citation2010). This notion predicts a particularly strong effect of acute GC exposure at the time of the circadian trough. However, the interaction between GR and the molecular clock goes in two directions: the molecular clock components may affect GR function, but GR also affects the molecular clock. This includes GRE-mediated transcriptional regulation of the Per1 gene (Balsalobre et al., Citation2000; So et al., Citation2009), but also regulation of many other genes, as evidence by prednisolone-induced effects in the rat hippocampus (Birnie et al., Citation2023). In the B-flat model the circadian rhythm of Bmal1 and Clock gene expression was found to be strongly attenuated in bone. Indeed, the rhythmic expression of many genes was attenuated under these conditions – refuting the notion that a constant exposure of B should lead to peaks of gene expression at given moments following an enhanced GR sensitivity (Schilperoort et al., Citation2021).
Alternatively, there is good evidence that the GR accessibility of the chromatin differs over the 24-hour cycle. The accessory clock protein REV-ERBα was shown to recruit GR, and to allow binding to specific loci on the DNA in the context of liver metabolism. Thus, out of phase exposure is not merely enhancing the extent of gene regulation, but rather may involve regulation of a different set of target genes (Caratti et al., Citation2018).
When does this matter?
The adverse effects of flat B that have been observed in mouse and rat studies as outlined below are substantial, and perhaps only a fraction of everything that is happening. The crucial aspect of this model is likely the absence of a trough in GR signaling, although enhanced tonic MR activation may also have effects via e.g. sympathetic outflow (Kroon et al., Citation2021). The consequences of GC signaling at the wrong moment of the day were also convincingly shown in a study on prenatal treatment of mice with GCs. In this study, pregnant mice were treated with a high dose of B, either at the moment of the natural peak or at the moment of the trough. Effects in the offspring were only observed in the out-of-phase group, and consisted of a changed emotional reactivity, including higher anxiety levels (Astiz et al., Citation2020). Likewise, in relation to bone disease and fat tissue, daily treatment with of mice with a very high dose of B at the moment of the endogenous peak was without consequence (Schilperoort et al., Citation2021; Tholen et al., Citation2022). With respect to disease models, Mary also made the point that the daily trough in B levels is absent in streptozotocin-treated rats, which develop type 1 diabetes (Dallman et al., Citation2000).
Clinical records of children born preterm suggested that the developmental consequences of out-of-phase glucocorticoids also affect humans (Astiz et al., Citation2020). Flattened rhythms of F also occur in humans. In fact, a study by Mary Dallman et al showed that chronically stressed human subjects had a smaller slope of the daily rhythm in F, in association with more visceral obesity (Rosmond et al., Citation1998). A meta-analysis over 80 published papers concluded that flattened F levels indeed were associated with a range of poor health outcomes, including but not limited to obesity, depression, inflammatory disease and cancer (Adam et al., Citation2017). The detection of the lack of a circadian trough in clinical populations should be greatly advanced by the recently developed ambulatory continuous subcutaneous sampling method that allows the determination of up to 72 hours of tissue concentrations of cortisol (and other steroids) (Upton et al., Citation2023).
Apart from chronic stress, also the use of synthetic GC may interfere with the diurnal rhythmicity of GR signaling. It is well known that these drugs activate negative feedback and lead to suppression of endogenous cortisol production, even when inhaled (Kachroo et al., Citation2022). Due to the fact that most drugs used have a long biological half-life despite a short pharmacological half-life, there is a continuous GR activation depending on the end organ (Buttgereit, Citation2021; Franco et al., Citation2019). The blunted HPA-axis hampers the orchestration of circadian rhythmicity in peripheral tissues by the key regulators B and F. This means that even in low dosages various formulations of synthetic glucocorticoids disturb the delicate process of aligned rhythmicity, which might be the explanation for the systemic effects of sub-physiological dosages of pharmacological treatment (Mckenzie et al., Citation2000; Van Staa, et al., Citation2005). Furthermore, only disruption of the circadian clock itself, e.g. miss-timed light exposure, disturbs the endogenous circadian GC rhythm and leads to systemic effects mimicking GC overexposure (Schilperoort et al., Citation2020). It is unknown whether therapeutic effects and side effects are equally sensitive to GC-signaling during the time of the circadian trough. It therefore may be worthwhile to develop strategies to minimize GC side effects by preventing misaligned activation of the GR.
Conclusions
From the B-flat model, it has become clear that even modestly elevated GC signaling at the “wrong” time of the day can contribute to the adverse effects of these hormones. The B-flat model is (sub) chronic, but additional work has shown that responses to acute GC treatment differ substantially depending on the time of day, with a worse health impact during the time of the normal circadian trough. These insights offer the perspective of timing-based strategies to diminish the negative impact of GCs on health.
Afterword
It is unclear whether the constitutively active MR mouse that Mary Dallman and David Pearce tried to generate in 1996 would have worked. The concept was based on a truncated receptor, that is constitutively active in luciferase assays, but that lacks one of the two activation functions of the MR (that is: AF-1) (Pearce & Yamamoto, Citation1993). Ten years later, a forebrain MR overexpression mouse was reported that did not have an obvious HPA axis phenotype (Lai et al., Citation2007). However, given that the corticosterone trough still existed, this model does not fully recapitulate Dallman’s original question. Perhaps this story reflects the fact that Mary Dallman knew to ask the proper questions and often was ahead of her time in realizing what we do and what we do not know about the regulation and effects of glucocorticoids. It is with gratitude that we dedicate this review to her memory.
Disclosure statement
No potential conflict of interest was reported by the author(s).
Additional information
Funding
References
- Adam, E. K., Quinn, M. E., Tavernier, R., McQuillan, M. T., Dahlke, K. A., & Gilbert, K. E. (2017). Diurnal cortisol slopes and mental and physical health outcomes: A systematic review and meta-analysis. Psychoneuroendocrinology, 83, 1–7. https://doi.org/10.1016/j.psyneuen.2017.05.018
- Akana, S. F., & Dallman, M. F. (1997). Chronic cold in adrenalectomized, corticosterone (B)-treated rats: facilitated corticotropin responses to acute restraint emerge as B increases. Endocrinology, 138(8), 3249–3258. https://doi.org/10.1210/endo.138.8.5291
- Akana, S. F., Scribner, K. A., Bradbury, M. J., Strack, A. M., Walker, C. D., & Dallman, M. F. (1992). Feedback sensitivity of the rat hypothalamo-pituitary-adrenal axis and its capacity to adjust to exogenous corticosterone. Endocrinology, 131(2), 585–594. https://doi.org/10.1210/endo.131.2.1322275
- Astiz, M., Heyde, I., Fortmann, M. I., Bossung, V., Roll, C., Stein, A., Grüttner, B., Göpel, W., Härtel, C., Obleser, J., & Oster, H. (2020). The circadian phase of antenatal glucocorticoid treatment affects the risk of behavioral disorders. Nature Communications, 11(1), 3593. https://doi.org/10.1038/s41467-020-17429-5
- Balsalobre, A., Brown, S. A., Marcacci, L., Tronche, F., Kellendonk, C., Reichardt, H. M., Schütz, G., & Schibler, U. (2000). Resetting of circadian time in peripheral tissues by glucocorticoid signaling. Science, 289(5488), 2344–2347. https://doi.org/10.1126/science.289.5488.2344
- Birnie, M. T., Claydon, M. D. B., Troy, O., Flynn, B. P., Yoshimura, M., Kershaw, Y. M., Zhao, Z., Demski-Allen, R. C. R., Barker, G. R. I., Warburton, E. C., Bortolotto, Z. A., Lightman, S. L., & Conway-Campbell, B. L. (2023). Circadian regulation of hippocampal function is disrupted with corticosteroid treatment. Proceedings of the National Academy of Sciences of the United States of America, 120(15), e2211996120. https://doi.org/10.1073/pnas.2211996120
- Bradbury, M. J., Akana, S. F., Cascio, C. S., Levin, N., Jacobson, L., & Dallman, M. F. (1991). Regulation of basal ACTH secretion by corticosterone is mediated by both type I (MR) and type II (GR) receptors in rat brain. The Journal of Steroid Biochemistry and Molecular Biology, 40(1-3), 133–142. https://doi.org/10.1016/0960-0760(91)90176-6
- Buttgereit, F. (2021). Glucocorticoids: surprising new findings on their mechanisms of actions. Annals of the Rheumatic Diseases, 80(2), 137–139. https://doi.org/10.1136/annrheumdis-2020-218798
- Caratti, G., Iqbal, M., Hunter, L., Kim, D., Wang, P., Vonslow, R. M., Begley, N., Tetley, A. J., Woodburn, J. L., Pariollaud, M., Maidstone, R., Donaldson, I. J., Zhang, Z., Ince, L. M., Kitchen, G., Baxter, M., Poolman, T. M., Daniels, D. A., Stirling, D. R., … Ray, D. W. (2018). REVERBa couples the circadian clock to hepatic glucocorticoid action. The Journal of Clinical Investigation, 128(10), 4454–4471. https://doi.org/10.1172/JCI96138
- Cederroth, C. R., Albrecht, U., Bass, J., Brown, S. A., Dyhrfjeld-Johnsen, J., Gachon, F., Green, C. B., Hastings, M. H., Helfrich-Förster, C., Hogenesch, J. B., Lévi, F., Loudon, A., Lundkvist, G. B., Meijer, J. H., Rosbash, M., Takahashi, J. S., Young, M., & Canlon, B. (2019). Medicine in the fourth dimension. Cell Metabolism, 30(2), 238–250. https://doi.org/10.1016/j.cmet.2019.06.019
- Dallman, M. F., Akana, S. F., Bhatnagar, S., Bell, M. E., & Strack, A. M. (2000). Bottomed out: metabolic significance of the circadian trough in glucocorticoid concentrations. International Journal of Obesity and Related Metabolic Disorders: journal of the International Association for the Study of Obesity, 24 Suppl 2(S2), S40–S46. https://doi.org/10.1038/sj.ijo.0801276
- Dallman, M. F., Akana, S. F., Bhatnagar, S., Bell, M. E., Choi, S., Chu, A., Horsley, C., Levin, N., Meijer, O., Soriano, L. R., Strack, A. M., & Viau, V. (1999). Starvation: Early signals, sensors, and sequelae. Endocrinology, 140(9), 4015–4023. https://doi.org/10.1210/endo.140.9.7001
- Dallman, M. F., Akana, S. F., Cascio, C. S., Darlington, D. N., Jacobson, L., & Levin, N. (1987). Regulation of ACTH secretion: Variations on a theme of B. Recent Progress in Hormone Research, 43, 113–173. https://doi.org/10.1016/b978-0-12-571143-2.50010-1
- Dallman, M. F., Levin, N., Cascio, C. S., Akana, S. F., Jacobson, L., & Kuhn, R. W. (1989). Pharmacological evidence that the inhibition of diurnal adrenocorticotropin secretion by corticosteroids is mediated via type I corticosterone-preferring receptors. Endocrinology, 124(6), 2844–2850. https://doi.org/10.1210/endo-124-6-2844
- Franco, L. M., Gadkari, M., Howe, K. N., Sun, J., Kardava, L., Kumar, P., Kumari, S., Hu, Z., Fraser, I. D. C., Moir, S., Tsang, J. S., & Germain, R. N. (2019). Glucocorticoids: Surprising new findings on their mechanisms of actions. The Journal of Experimental Medicine, 216(2), 384–406. https://doi.org/10.1084/jem.20180595
- Havel, P. J., Busch, B. L., Curry, D. L., Johnson, P. R., Dallman, M. F., & Stern, J. S. (1996). Predominately glucocorticoid agonist actions of RU-486 in young specific-pathogen-free Zucker rats. The American Journal of Physiology, 271(3 Pt 2), R710–7. https://doi.org/10.1152/ajpregu.1996.271.3.R710
- Heilman, F. R., & Kendall, E. C. (1956). The influence of the hormones of the adrenal cortex, compounds A, B, and E, on the deposition of fat in the mouse. Proceedings of the Staff Meetings. Mayo Clinic, 31(16), 454–459.
- Henley, D., Lightman, S., & Carrell, R. (2016). Cortisol and CBG - Getting cortisol to the right place at the right time. Pharmacology & Therapeutics, 166, 128–135. https://doi.org/10.1016/j.pharmthera.2016.06.020
- Herman, J. P., Figueiredo, H., Mueller, N. K., Ulrich-Lai, Y., Ostrander, M. M., Choi, D. C., & Cullinan, W. E. (2003). Central mechanisms of stress integration: Hierarchical circuitry controlling hypothalamo-pituitary-adrenocortical responsiveness. Frontiers in Neuroendocrinology, 24(3), 151–180. https://doi.org/10.1016/j.yfrne.2003.07.001
- Jiang, Z., Chen, C., Weiss, G. L., Fu, X., Stelly, C. E., Sweeten, B. L. W., Tirrell, P. S., Pursell, I., Stevens, C. R., Fisher, M. O., Begley, J. C., Harrison, L. M., & Tasker, J. G. (2022). Stress-induced glucocorticoid desensitizes adrenoreceptors to gate the neuroendocrine response to somatic stress in male mice. Cell Reports, 41(3), 111509. https://doi.org/10.1016/j.celrep.2022.111509
- Kachroo, P., Stewart, I. D., Kelly, R. S., Stav, M., Mendez, K., Dahlin, A., Soeteman, D. I., Chu, S. H., Huang, M., Cote, M., Knihtilä, H. M., Lee-Sarwar, K., McGeachie, M., Wang, A., Wu, A. C., Virkud, Y., Zhang, P., Wareham, N. J., Karlson, E. W., … Lasky-Su, J. A. (2022). Metabolomic profiling reveals extensive adrenal suppression due to inhaled corticosteroid therapy in asthma. Nature Medicine, 28(4), 814–822. https://doi.org/10.1038/s41591-022-01714-5
- Karssen, A. M., Meijer, O. C., van der Sandt, I. C., Lucassen, P. J., de Lange, E. C., de Boer, A. G., & De Kloet, E. R. (2001). Multidrug resistance P-glycoprotein hampers the access of cortisol but not of corticosterone to mouse and human brain. Endocrinology, 142(6), 2686–2694. https://doi.org/10.1210/endo.142.6.8213
- Kim, J. S., & Iremonger, K. J. (2019). Temporally tuned corticosteroid feedback regulation of the stress axis. Trends in Endocrinology and Metabolism 30(11), 783–792. https://doi.org/10.1016/j.tem.2019.07.005
- Kroon, J., Schilperoort, M., In Het Panhuis, W., van den Berg, R., van Doeselaar, L., Verzijl, C. R. C., van Trigt, N., Mol, I. M., Sips, H. H. C. M., van den Heuvel, J. K., Koorneef, L. L., van der Sluis, R. J., Fenzl, A., Kiefer, F. W., Vettorazzi, S., Tuckermann, J. P., Biermasz, N. R., Meijer, O. C., Rensen, P. C. N., & Kooijman, S. (2021). A physiological glucocorticoid rhythm is an important regulator of brown adipose tissue function. Molecular Metabolism, 47, 101179. https://doi.org/10.1016/j.molmet.2021.101179
- Lai, M., Horsburgh, K., Bae, S.-E., Carter, R. N., Stenvers, D. J., Fowler, J. H., Yau, J. L., Gomez-Sanchez, C. E., Holmes, M. C., Kenyon, C. J., Seckl, J. R., & Macleod, M. R. (2007). Forebrain mineralocorticoid receptor overexpression enhances memory, reduces anxiety and attenuates neuronal loss in cerebral ischaemia. The European Journal of Neuroscience, 25(6), 1832–1842. https://doi.org/10.1111/j.1460-9568.2007.05427.x
- Laugesen, K., Jørgensen, J. O. L., Petersen, I., & Sørensen, H. T. (2019). Fifteen-year nationwide trends in systemic glucocorticoid drug use in Denmark. European Journal of Endocrinology, 181(3), 267–273. https://doi.org/10.1530/EJE-19-0305
- Leeuwen, J. M. C., Vink, M., Fernández, G., Hermans, E. J., Joëls, M., Kahn, R. S., & Vinkers, C. H. (2018). At-risk individuals display altered brain activity following stress. Neuropsychopharmacology: official Publication of the American College of Neuropsychopharmacology, 43(9), 1954–1960. https://doi.org/10.1038/s41386-018-0026-8
- Lightman, S. L., & Conway-Campbell, B. L. (2010). The crucial role of pulsatile activity of the HPA axis for continuous dynamic equilibration. Nature Reviews. Neuroscience, 11(10), 710–718. https://doi.org/10.1038/nrn2914
- Limumpornpetch, P., Morgan, A. W., Tiganescu, A., Baxter, P. D., Nyawira Nyaga, V., Pujades-Rodriguez, M., & Stewart, P. M. (2022). The effect of endogenous cushing syndrome on all-cause and cause-specific mortality. The Journal of Clinical Endocrinology and Metabolism, 107(8), 2377–2388. https://doi.org/10.1210/clinem/dgac265
- Mckenzie, R., Reynolds, J. C., OFallon, A., Dale, J., Deloria, M., Blackwalder, W., & Straus, S. E. (2000). Decreased bone mineral density during low dose glucocorticoid administration in a randomized, placebo controlled trial. Journal of Rheumatology, 27(9), 2222–2226.
- Meijer, O. C. (2006). Understanding stress through the genome. Stress, 9(2), 61–67. https://doi.org/10.1080/10253890600799669
- Meijer, O. C., Van Oosten, R. V., & De Kloet, E. R. (1997). Elevated basal trough levels of corticosterone suppress hippocampal 5-hydroxytryptamine(1A) receptor expression in adrenally intact rats: implication for the pathogenesis of depression. Neuroscience, 80(2), 419–426. https://doi.org/10.1016/s0306-4522(97)00008-0
- Monczor, F., Chatzopoulou, A., Zappia, C. D., Houtman, R., Meijer, O. C., & Fitzsimons, C. P. (2019). A model of glucocorticoid receptor interaction with coregulators predicts transcriptional regulation of target genes. Frontiers in Pharmacology, 10, 214. https://doi.org/10.3389/fphar.2019.00214
- Nader, N., Chrousos, G. P., & Kino, T. (2010). Interactions of the circadian CLOCK system and the HPA axis. Trends in Endocrinology and Metabolism, 21(5), 277–286. https://doi.org/10.1016/j.tem.2009.12.011
- Nixon, M., Mackenzie, S. D., Taylor, A. I., Homer, N. Z. M., Livingstone, D. E., Mouras, R., Morgan, R. A., Mole, D. J., Stimson, R. H., Reynolds, R. M., Elfick, A. P. D., Andrew, R., & Walker, B. R. (2016). ABCC1 confers tissue-specific sensitivity to cortisol versus corticosterone: A rationale for safer glucocorticoid replacement therapy. Science Translational Medicine, 8(352), 352ra109. https://doi.org/10.1126/scitranslmed.aaf9074
- Pace, T. W. W., & Heim, C. M. (2011). A short review on the psychoneuroimmunology of posttraumatic stress disorder: From risk factors to medical comorbidities. Brain, Behavior, and Immunity, 25(1), 6–13. https://doi.org/10.1016/j.bbi.2010.10.003
- Pearce, D., & Yamamoto, K. R. (1993). Mineralocorticoid and glucocorticoid receptor activities distinguished by nonreceptor factors at a composite response element. Science, 259(5098), 1161–1165. https://doi.org/10.1126/science.8382376
- Polman, J. A. E., De Kloet, E. R., & Datson, N. A. (2013). Two populations of glucocorticoid receptor-binding sites in the male rat hippocampal genome. Endocrinology, 154(5), 1832–1844. https://doi.org/10.1210/en.2012-2187
- Ratka, A., Sutanto, W., Bloemers, M., & De Kloet, E. R. (1989). On the role of brain mineralocorticoid (type I) and glucocorticoid (type II) receptors in neuroendocrine regulation. Neuroendocrinology, 50(2), 117–123. https://doi.org/10.1159/000125210
- Reddy, T. E., Gertz, J., Crawford, G. E., Garabedian, M. J., & Myers, R. M. (2012). The hypersensitive glucocorticoid response specifically regulates period 1 and expression of circadian genes. Molecular and Cellular Biology, 32(18), 3756–3767. https://doi.org/10.1128/MCB.00062-12
- Reddy, T. E., Pauli, F., Sprouse, R. O., Neff, N. F., Newberry, K. M., Garabedian, M. J., & Myers, R. M. (2009). Genomic determination of the glucocorticoid response reveals unexpected mechanisms of gene regulation. Genome Research, 19(12), 2163–2171. https://doi.org/10.1101/gr.097022.109
- Reul, J. M., van den Bosch, F. R., & De Kloet, E. R. (1987). Relative occupation of type-I and type-II corticosteroid receptors in rat brain following stress and dexamethasone treatment: functional implications. The Journal of Endocrinology, 115(3), 459–467. https://doi.org/10.1677/joe.0.1150459
- Rosmond, R., Dallman, M. F., & Björntorp, P. (1998). Stress-related cortisol secretion in men: relationships with abdominal obesity and endocrine, metabolic and hemodynamic abnormalities. Journal of Clinical Endocrinology & Metabolism, 83(6), 1853–1859. https://doi.org/10.1210/jc.83.6.1853
- Sarabdjitsingh, R. A., Isenia, S., Polman, A., Mijalkovic, J., Lachize, S., Datson, N., de Kloet, E. R., & Meijer, O. C. (2010). Disrupted corticosterone pulsatile patterns attenuate responsiveness to glucocorticoid signaling in rat brain. Endocrinology, 151(3), 1177–1186. https://doi.org/10.1210/en.2009-1119
- Schilperoort, M., Bravenboer, N., Lim, J., Mletzko, K., Busse, B., van Ruijven, L., Kroon, J., Rensen, P. C. N., Kooijman, S., & Winter, E. M. (2020). Circadian disruption by shifting the light-dark cycle negatively affects bone health in mice. FASEB Journal: Official Publication of the Federation of American Societies for Experimental Biology, 34(1), 1052–1064. https://doi.org/10.1096/fj.201901929R
- Schilperoort, M., Kroon, J., Kooijman, S., Smit, A. E., Gentenaar, M., Mletzko, K., Schmidt, F. N., van Ruijven, L., Busse, B., Pereira, A. M., Appelman-Dijkstra, N. M., Bravenboer, N., Rensen, P. C. N., Meijer, O. C., & Winter, E. M. (2021). Loss of glucocorticoid rhythm induces an osteoporotic phenotype in female mice. Aging Cell, 20(10), e13474. https://doi.org/10.1111/acel.13474
- Schinkel, A. H., Smit, J. J., van Tellingen, O., Beijnen, J. H., Wagenaar, E., van Deemter, L., Mol, C. A., van der Valk, M. A., Robanus-Maandag, E. C., & Te Riele, H. P. (1994). Disruption of the mouse mdr1a P-glycoprotein gene leads to a deficiency in the blood-brain barrier and to increased sensitivity to drugs. Cell, 77(4), 491–502. https://doi.org/10.1016/0092-8674(94)90212-7
- So, A. Y.-L., Bernal, T. U., Pillsbury, M. L., Yamamoto, K. R., & Feldman, B. J. (2009). Glucocorticoid regulation of the circadian clock modulates glucose homeostasis. Proceedings of the National Academy of Sciences of the United States of America, 106(41), 17582–17587. https://doi.org/10.1073/pnas.0909733106
- Tholen, S., Patel, R., Agas, A., Kovary, K. M., Rabiee, A., Nicholls, H. T., Bielczyk-Maczyńska, E. Yang, W., Kraemer, F. B., & Teruel, M. N. (2022). Flattening of circadian glucocorticoid oscillations drives acute hyperinsulinemia and adipocyte hypertrophy. Cell Reports, 13(39), 111018. https://doi.org/10.1016/j.celrep.2022.111018
- Upton, T. J., Zavala, E., Methlie, P., Kämpe, O., Tsagarakis, S., Øksnes, M., Bensing, S., Vassiliadi, D. A., Grytaas, M. A., Botusan, I. R., Ueland, G., Berinder, K., Simunkova, K., Balomenaki, M., Margaritopoulos, D., Henne, N., Crossley, R., Russell, G., Husebye, E. S., & Lightman, S. L. (2023). High-resolution daily profiles of tissue adrenal steroids by portable automated collection. Science Translational Medicine, 15(701), eadg8464. https://doi.org/10.1126/scitranslmed.adg8464
- van Haarst, A. D., Oitzl, M. S., & De Kloet, E. R. (1997). Facilitation of feedback inhibition through blockade of glucocorticoid receptors in the hippocampus. Neurochemical Research, 22(11), 1323–1328. https://doi.org/10.1023/a:1022010904600
- Van Staa, T. P., Leufkens, H. G. M., Abenhaim, L., Zhang, B., & Cooper, C. (2005). Use of oral corticosteroids and risk of fractures. Journal of Bone and Mineral Research, 20(8), 1486–1493. https://doi.org/10.1359/jbmr.2005.20.8.1486
- Viho, E. M., Kroon, J., Feelders, R. A., Houtman, R., van den Dungen, E., Pereira Arias, A. M., Hunt, H., Hofland, L. J., & Meijer, O. C. (2022). Peripheral Glucocorticoid receptor antagonism by relacorilant with modest HPA axis disinhibition. Journal of Endocrinology, 256(2), 222063. https://doi.org/10.1530/JOE-22-0263
- Walker, J. J., Spiga, F., Gupta, R., Zhao, Z., Lightman, S. L., & Terry, J. R. (2015). Rapid intra-adrenal feedback regulation of glucocorticoid synthesis. Journal of the Royal Society, Interface, 12(102), 20140875. https://doi.org/10.1098/rsif.2014.0875
- Windle, R. J., Wood, S. A., Kershaw, Y. M., Lightman, S. L., Ingram, C. D., & Harbuz, M. S. (2001). Increased corticosterone pulse frequency during adjuvant-induced arthritis and its relationship to alterations in stress responsiveness. Journal of Neuroendocrinology, 13(10), 905–911. https://doi.org/10.1046/j.1365-2826.2001.00715.x
- Wyrwoll, C. S., Holmes, M. C., & Seckl, J. R. (2011). 11β-hydroxysteroid dehydrogenases and the brain: from zero to hero, a decade of progress. Frontiers in Neuroendocrinology, 32(3), 265–286. https://doi.org/10.1016/j.yfrne.2010.12.001