Abstract
Successful and efficient emotion regulation (ER) is a key mechanism for mental health. However, acute stress may impact the ability to cognitively regulate negative emotions due to its immediate effects on executive functioning. Based on previous studies, we expected that the time at which ER is tested after a stressor might have a decisive influence, with impairments in ER being more pronounced immediately after stress as compared to a later post-stress phase. To investigate such a time-dependent effect of stress on ER, we investigated 50 healthy adults (26 female) who were exposed to either the Trier Social Stress Test (n = 25) or a control condition (n = 25). Afterwards subjects conducted a cognitive ER task during which they were instructed to either regulate (cognitive reappraisal) or passively view neutral and negative visual stimuli. The ER task was divided into an early (0–20 minutes) and a late post-stress phase (20–40 minutes). Salivary cortisol and α-amylase were assessed as markers of the neuroendocrine stress response. Self-reported emotional state, the mean activity of the late positive potential measured via electroencephalogram (EEG), and corrugator electromyographic activity (EMG) were used as indices of ER. While the groups did not differ in the early post-stress phase, our results suggest a stress-related impairment in ER in the late post-stress phase. This effect was evident in all ER outcome variables (subjective rating, EEG, and EMG data). These results suggest a time-specific stress effect on cognitive reappraisal, which would have implications for reappraisal as a possible stress management technique.
1. Introduction
The deliberate and effective regulation of emotions is considered a key mechanism for maintaining mental health (McRae & Gross, Citation2020). However, its potential utility as a stress management technique highly depends on the extent to which we can rely on our regulation skills when we need them most: in the face of acute stressors.
As compared to unconscious implicit regulation processes of emotional incidents, this study investigates cognitive emotion regulation (ER) as an active and deliberate regulatory mechanism that adaptively and flexibly adjusts responses to emotional stimuli and/or situations (Gross & John, Citation2003). Among several ER strategies, cognitive reappraisal is highly effective (Webb et al., Citation2012) and has been associated with long-term positive health outcomes (e.g. Aldao et al., Citation2010; Gross & John, Citation2003). Cognitive reappraisal involves the conscious reinterpretation of emotion-eliciting situations by modifying their meaning. Individuals who use reappraisal strategies in daily life are more likely to experience generally more positive emotions and less anger, show more adaptive physiological responses to threat, report greater overall well-being, and generally have fewer psychopathological symptoms (Feder et al., Citation2009; Kalisch et al., Citation2015; Troy & Mauss, Citation2011). For this reason, reappraisal training is often incorporated into cognitive behavioral therapy. Interestingly, however, the use and benefits of reappraisal seem to depend on individual and contextual factors (Ford & Troy, Citation2019) and its flexible use (Bonanno & Burton, Citation2013), so it is of great interest for both basic emotion regulation research and clinical practice to identify factors that challenge reappraisal performance, such as acute stress. Given the crucial role of cognitive reappraisal in the etiology and prevention of stress-related psychopathology, as well as its beneficial effects against stress-related dysfunction, the question arises as to whether reappraisal could potentially be a valuable tool for stress management in general. To get closer to answering this question, it seems important to investigate the effects of acute stress on ER functioning, especially cognitive reappraisal.
Acute stress is characterized by a cascade of neuroendocrine stress responses: (1) the rapid-acting sympathetic nervous system results in the release of adrenalin and noradrenalin, followed by (2) the slow-going hypothalamic-pituitary-adrenal (HPA) axis, leading to the release of glucocorticoids (mainly cortisol). While catecholamines increase immediately in the face of stress and normalize within ∼15 minutes, cortisol usually peaks 15–25 minutes after stress onset and contributes to the active restoration of homeostasis in the aftermath of a stressor via genomic effects lasting for hours or even days after stress (Hermans et al., Citation2014). On a neural level, both catecholamines and cortisol interact with cortical and subcortical structures, exerting specific and in part opposing effects. These neuroendocrine interactions orchestrate a strategic reallocation of cognitive resources in the face of stress, with the aim of promoting vigilance and salience processing to enable rapid, unpremeditated responses to a potential threat, but at the expense of higher-order prefrontal functions (see Hermans et al., Citation2014, for details). Note, however, that cognitive reappraisal is known to rely heavily on prefrontal functioning (Buhle et al., Citation2014): To reappraise an aversive situation requires the ability to think of an alternative interpretation (cognitive flexibility) and to successfully implement and defend it against competing thoughts (working memory, response inhibition). Importantly, previous studies have reported detrimental effects of acute stress on both, working memory and cognitive flexibility (Duan et al., Citation2020; Plessow et al., Citation2011; Shields et al., Citation2016). Interestingly, there is also evidence of stress-induced improvements in cognitive functioning (for results on emotion regulation, see below). To explain this, numerous possible factors are currently discussed, the experimental timing being one of them (for an overview, see Plieger & Reuter, Citation2020, and Shields, Citation2020). Hence, there are biological and theoretical considerations suggesting that cognitive reappraisal might show impairments shortly after acute stress, while it might be less affected or even improved in the longer aftermath of stress, suggesting a high-sensitive time-dependent effect of stress on cognitive ER.
In recent years, scientific interest in the effects of stress on ER has increased considerably, yielding an ambiguous patchwork of results: while some earlier studies report detrimental effects of stress on the regulation of fear (Raio et al., Citation2013) and anger (Zhan et al., Citation2017) or the ability to distract oneself from aversive pictures (i.e. distraction as another ER strategy; Kinner et al., Citation2014), other studies report no effects of an acute stress manipulation on cognitive reappraisal abilities (Sandner et al., Citation2021; Shermohammed et al., Citation2017). There are even some recent studies that provide preliminary evidence that acute stress may improve cognitive ER under certain conditions (Langer et al., Citation2020, Citation2021, Citation2022a, Citation2023). A closer look at these ambiguous results shows that the presence, intensity and direction of a stress effect on ER might critically depend on various influencing factors (see discussion), one of them certainly being the experimental timing: some studies indicate detrimental effects of stress when ER is tested immediately or shortly after the stressor (Kinner et al., Citation2014; Raio et al., Citation2013; Zhan et al., Citation2017) while others studies reported improved ER when tested in the longer aftermath of stress (Langer et al., Citation2020, Citation2021; but see also Langer et al., Citation2023). In addition, studies that administered cortisol externally instead of experimental stress induction also reported an improvement in ER abilities (Jentsch et al., Citation2019; Langer et al., Citation2022b). These latter studies emphasize the importance of cortisol in the restoration of homeostasis in the longer aftermath of a stressor and underline the decisive role of timing in the dynamic interplay of stress and emotion regulation.
Against the backdrop of this empirical patchwork, this study aimed at systematically examining the factor of timing by exposing participants to either a stress or a control condition followed by an ER paradigm including two different time windows (early phase: 0–20 minutes after stressor offset; late phase: 20–40 minutes after stressor offset). With the aim to investigate whether, when and for how long reappraisal abilities are affected by acute stress, we chose these time windows to meet theoretical as well as methodological considerations, which prevented us from investigating a delayed cortisol-induced facilitation of cognitive emotion regulation processes in the aftermath of stress (see limitations in the discussion section). We included sex and the individual cortisol increase as covariates of interest in all our analyses since recent studies suggest cortisol-related sex differences in the presence and direction of a stress effect on ER (Langer et al., Citation2020, Citation2022a, Citation2023), see discussion for details. As a further essential contribution to this field, we tested the effect of stress on reappraisal at multiple psychophysiological outcome levels reflecting the three components of emotional responses (Barrett et al., Citation2007): (1) subjective emotional state ratings, (2) event-related potentials, i.e. the late positive potential (LPP), and (3) electromyogram (EMG) of the corrugator supercilii. The LPP (2) typically becomes evident at 500 ms post stimulus presentation over posterior recording sites (Hajcak et al., Citation2009). It is associated with the emotional intensity of a stimulus and was linked to facilitated attention to emotional stimuli and emotion processing (Hajcak et al., Citation2010; Schupp et al., Citation2006). The LPP served as a neurophysiological marker of emotional responding in a variety of ER studies (e.g. Foti & Hajcak, Citation2008; Hajcak et al., Citation2010; Schönfelder et al., Citation2014). The use of facial EMG (3) has proven sufficient validity evidence to measure emotional expressivity (Mauss & Robinson, Citation2009). The activity of the corrugator supercilia is believed to increase with the unpleasantness of affective stimuli (Bradley & Lang, Citation2000) and has therefore been assessed via EMG in a multitude of studies investigating emotional processing and regulation (e.g. Jackson et al., Citation2000; Pedder et al., Citation2016; Ray et al., Citation2010; Schönfelder et al., Citation2014).
Based on the theoretical assumptions and the discussed results of previous research, we hypothesized a stress-related impairment of cognitive reappraisal (as indicated by increased subjective emotional state ratings, LPP magnitudes and corrugator supercilii activity) in the stress compared to the control group. We expected this impairment to be more pronounced in the early compared to the late ER phase due to the stress-related immediate downregulation of higher-order executive functioning.
2. Materials and methods
2.1. Participants
A priori calculations of the required sample size were conducted with G*Power 3.1 (Faul et al., Citation2009), assuming a small-sized effect (d = 0.3) based on previous results (Langer et al., Citation2020, Citation2022a). To detect an interaction between group (stress vs. control) and the ER phases (early vs. late) in a repeated-measure design with a power of 1- β ≥ 0.95, an α-error probability of 0.05 and an assumed correlation of r = 0.4 for repeated measurements, 46 participants (in total) were required. Therefore, 50 medication-free volunteers (26 female) aged between 18 and 28 were recruited for study participation at the University of Mainz, Germany. For descriptive data of the sample and statistical indices of the respective group comparisons see . Subjects were randomly assigned to either a stress (SG, n = 25) or a control group (CG, n = 25). All participants were nonsmokers. All female volunteers were not pregnant, were not using hormonal oral contraceptives, and were tested during the luteal phase of their menstrual cycle (defined as the 15th to 21st day of the menstrual cycle as assessed by self-report). Exclusion criteria included: any acute or chronic physical disease (e.g. cardiovascular disease, diabetes, endocrine disease, epilepsy), previous experience with the stress protocol or the ER paradigm, and illegal drug consumption. These exclusion criteria were verified by telephone and/or face-to-face structured interviews which were carried out by trained psychologists. Subjects were instructed to refrain from eating and drinking anything but water one hour prior to the experiment and to abstain from alcohol and exercise on the day of assessment, which took place between 14:00 and 18:00. All participants gave written informed consent before study participation. The study was carried out in accordance with The Code of Ethics of the World Medical Association (Declaration of Helsinki) and was approved by the Ethics Committee of the University of Mainz (approval number 2016-JGU-psychEK-008), Institute of Psychology. All participants received course credit or 40€for their participation.
Table 1. Descriptive data and statistical indices for sample characteristics as well as stress induction measures and CERT difference scores.
2.2. Experimental design and procedure
Upon arrival at the laboratory, subjects rested for about 60 minutes while EEG and EMG preparation took place. Participants were then given detailed instructions for the experimental procedure and the ER strategy. Participants were instructed to reappraise the content of the image with the aim to decrease their upcoming emotional reaction to it while staying in the displayed scene (by e.g. thinking of a positive ending of the given situation) instead of distracting themselves (by thinking of e.g. the next trip to the supermarket). To practice the reappraisal strategy, eight example pictures were presented, and the participants’ responses were reviewed by the experimenters until they felt confident that the instructions were properly understood, and the participants applied the strategy correctly. Thereafter, either the stress or the control condition was carried out, see Section 2.3. Saliva sampling took place prior to the stress task or placebo protocol (T0, baseline), at the end of the stress protocol prior to the ER paradigm (T1, +20 minutes), between the early and late phases of the ER paradigm (T2, +40 minutes), after completion of the experiment (T3, +60 minutes), and after the complete testing (T4, +90 minutes). In addition, negative affect was measured before (T0, baseline) and after the stress induction (T1, +20 minutes) as well as after the ER paradigm (T2, +60 minutes). After completion of the ER paradigm, the participants completed a short questionnaire measuring the individual’s effort regarding the ER task. The study procedure is displayed in .
2.3. Stress protocol (stress vs. placebo) and measures of reactivity
For stress induction, we used the Trier Social Stress Test (TSST), a widely used and valid procedure to induce stress in the laboratory. The TSST was carried out according to Kirschbaum et al. (Citation1993). Participants were instructed to prepare a 5-minute free speech during which they presented themselves as the perfect candidate for a vacant position (‘dream job’) to a committee composed of one male and one female evaluator dressed in white lab coats. The committee was asked to maintain a neutral face during the whole experiment. Additionally, the speech was recorded by a video camera placed in front of the participant. Preparation time was 10 minutes (for which the committee left the room). After the speech, a mental arithmetic task (counting backwards in steps of 17 from 2043) was performed for 5 minutes. In case of an error, participants had to start again from 2043. For the control condition, we followed the procedure proposed by Het et al. (Citation2009), where the participant is accompanied into an empty room with the instruction to talk for 5 minutes about a favorite topic with a preparation time of 10 min. After preparation, the experimenter entered the room and asked the participant to stand up and take a position somewhere in the room. Subsequently, the experimenter left the room and the participant talked aloud about his/her chosen topic. After 5 minutes, the participant was told adding the number 15 starting at 0 for 5 minutes. The placebo condition took place in the same room as the TSST, but there was no camera, microphone, or committee present. At the end of the study, each participant received a full debriefing with respect to the study aims.
We collected five saliva samples to ensure frequent monitoring of the endocrine stress response (cortisol and α-amylase). All saliva samples were stored at −20 °C and assayed by Dresden LabService GmbH (Dresden, Germany) using chemiluminescence immunoassay kits. In addition, subjective stress responses to the TSST were assessed by subjective ratings of negative affect at three time points using the PANAS (Watson et al., Citation1988). The PANAS consists of 20 adjectives reflecting positive or negative emotional states. The participants’ ratings on a five-point Likert scale can be summed up to a total score of positive and negative affect with higher scores reflecting more intense emotional states.
2.4. Emotion regulation paradigm
We employed a modified version of the Cognitive Emotion Regulation Task (CERT), which has been previously described and applied in several studies (Kanske et al., Citation2011; Sandner et al., Citation2021; Schönfelder et al., Citation2014) and has demonstrated sufficient validity evidence to be applied when investigating cognitive ER in an experimental setting. In the present study, negative and neutral pictures were used. The stimuli were selected from the International Affective Picture System (Lang et al., Citation2008) and the Emotional Picture Set (Wessa et al., Citation2010). All images depicted human figures or at least parts of the human body, as it has been shown that images with social content may be differentially processed on a neural (Tso et al., Citation2018) and psychophysiological level (Ferri et al., Citation2012). Normative ratings of neutral stimuli and negative stimuli differed significantly in valence (neutral: Mv= 4.98, SDv= 0.20; negative: Mv= 2.09, SDv= 0.42; t(158) = 55.02, p < 0.001) and arousal (neutral: Ma= 2.92, SDa= 0.28; negative: Ma= 6.59, SDa= 0.50; t(158) = 57.46, p < 0.001). In the present study, two within-subject task conditions were presented. In the ‘view’ condition, participants were instructed to draw their attention to the presented stimulus and to process the stimulus as naturally as possible without altering their emotional reaction. In the ‘reappraisal’ condition, participants were instructed to reinterpret the situation depicted in the picture (situation-focused reappraisal) in such a way as to reduce the negative emotion evoked by the stimulus, e.g. by imagining a rather positive outcome of the situation. The condition*task combinations were balanced with respect to affective norm values provided by the authors of the picture sets (Lang et al., 2008; Wessa et al., Citation2010). To investigate the temporal dynamics of the stress response, stimuli were presented in a randomized block design with two blocks, an early post-stress phase and a late post-stress phase, each lasting for 20 minutes. Each block consisted of 20 view-neutral trials, 20 view-negative, 20 reappraisal-negative, (and 20 reappraisal-neutral trials that were not included in this paper’s analyses). The picture-instruction combinations were presented in a pseudo-randomized order, i.e. neither the condition nor the task was repeated more than three times in a row. No picture was used twice.
Each trial of the ER task started with a fixation cross (500 ms) followed by a single-word instruction (“View” or “Reappraise”) (2000 ms), displayed as white text on a black background. Subsequently, the stimulus was presented (5000 ms), followed by 4000 ms rating phase using the Self-Assessment-Manikin Scale for valence (SAM; Bradley & Lang, Citation1994) ranging from 1 (negative valence) to 9 (positive valence) to assess the individual emotional state. Finally, a variable inter-trial interval of 3500–5000 ms was presented. The trial structure of the ER task is shown in . The complete ER paradigm lasted about 40 minutes, with a break of approximately 2 minutes between the early and late post-stress phase.
2.5. Neurophysiological data acquisition and analysis (EEG, EMG)
EEG was recorded using Ag/AgCl-electrodes from 32 scalp positions according to the international 10–20 system. Online EEG signals were referenced to the right mastoid. Four additional electrodes were placed around the orbital regions of the face to monitor vertical and horizontal eye movements (EOG). Electrode impedances were kept <10 kΩ before starting the experimental paradigm. EEG and EOG data were registered with a sampling rate of 1 kHz and 16-bit A/D conversion using BrainAMP amplifiers (Brain Products, Inc., Munich, Germany). Brain Vision Analyzer II software (Brain Products GmbH, Munich, Germany) was used for offline analysis. EEG data were down-sampled to 250 Hz, re-referenced to the averaged mastoid, and filtered with a 0.1 to 40 Hz (24 dB/oct) bandpass filter (Picton et al., Citation2000). An independent component analysis algorithm was applied to correct for stereotypic artifacts (eye-blinks) using the internal algorithm provided by the Brain Vision Analyzer II software. Event-related potential (ERP) epochs were extracted from −500 to 5000 ms relative to stimulus presentation and segments were baseline corrected to 250 ms pre-stimulus onset. Artifacts were rejected semi-automatically using the following criteria: peak-to-peak differences > 300 µV, voltage steps of 50 µV between sampling points and a maximum difference of less than .50 µV within 100 ms intervals. Additional artifacts were identified and rejected based on visual inspection. Stimulus-locked ERPs were constructed using EEG signals of a parietal cluster (P1-, Pz- and P2-electrodes) by averaging trials separately for each condition and participant. For visualization of the ERPs in , the Pz-electrode was used. The LPP comprised the time-range of 500 to 5000 ms.
To measure EMG activity related to negative valence of the stimuli, two 4 mm Ag/AgCl electrodes were placed over the corrugator supercilii, see for a schematic depiction of EMG electrode placement (Fridlund & Cacioppo, Citation1986). EMG was amplified using a BrainAmp ExG amplifier (Brain Products GmbH, Munich, Germany) and registered with a sampling frequency of 1 kHz. Raw EMG signals were filtered using a 30 Hz low cutoff, a 500 Hz high cutoff and a 50 Hz notch filter. EMG signals were full wave rectified and smoothed with a moving average over 125 ms. EMG scores were calculated as the change in activity relative to the baseline period of 1000 ms prior to stimulus onset (Blumenthal et al., Citation2005). The time window was defined by averaging the segment of 0 ms to 5000 ms continuous data spanning the entire 5 seconds of stimulus presentation.
Figure 2. Schematic depiction of the electrode placement for EMG recording over the corrugator supercilii: one electrode is placed on an imagery vertical line that traverses the inner commissure of the eye fissure; the second electrode is placed 1 cm lateral and slightly superior to the first one (see Fridlund and Cacioppo, Citation1986).
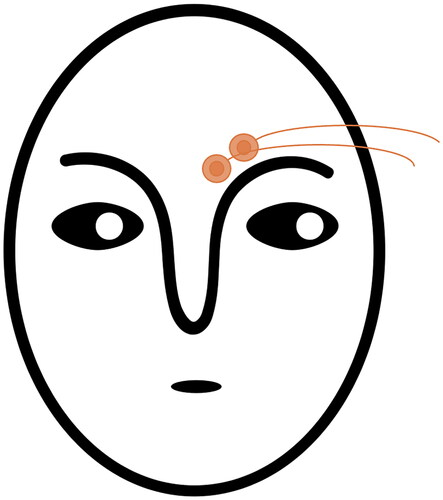
2.6. Statistical analyses
Statistical analyses were carried out using SPSS 23 (SPSS Inc., Chicago, IL, USA). For all analyses of variance (ANOVAs) described below, statistical effects were evaluated using the Greenhouse–Geisser correction where appropriate. To minimize type I error, Bonferroni Holm correction was applied for post-hoc analyses (Holm’s α).
To test for effective experimental manipulation, we first analyzed group differences in acute stress reactivity to the TSST: (a) Regarding changes in negative affect, we analyzed PANAS data using a 3 × 2 mixed ANOVA with ‘time’ (3 levels, within-subject factor) and ‘group’ (2 levels, between-subject factor). (b) Cortisol and (c) α-amylase data were logarithmised to base 10 to reduce typical data skewness. Changes in salivary concentrations were compared using 5 × 2 mixed ANOVAs with ‘time’ (5 levels) as within-subject factor and ‘group’ (2 levels) as between-subject factor. As some studies have reported sex differences in acute stress reactivity (Kudielka & Kirschbaum, Citation2005), we included ‘sex’ as a covariate in all ANOVAs. Second, to check for successful manipulation of the CERT irrespective of stress, we conducted a 2 × 3 rmANOVA with ‘phase’ (2 levels: early and late) and ‘task’ (3 levels: view-neutral, view-negative, reappraisal-negative) as within-subjects factors.
To test our hypothesis of time-dependent group differences in ER, we calculated difference scores with higher scores indicating higher regulation success for (1) subjective valence ratings (reappraise-negative minus view-negative), (2) EEG data (view-negative minus reappraisal-negative), and (3) EMG data (view-negative minus reappraise-negative). For each difference score, we conducted a 2 × 2 mixed rmANOVA with ‘phase’ (2 levels: early and late) as a within-subject factor and ‘group’ (2 levels) as between-subject factor. (Note that groups did not differ in emotional reactivity as assessed by view-neutral minus view-negative in both experimental phases and in all outcome measures, see Supplement 3 for details.) As some studies reported stress effects on ER depending on sex and cortisol reactivity (Kinner et al., Citation2014; Langer et al., Citation2020, Citation2021, Citation2023), we included ‘sex’ and the individual ‘cortisol increase’ (individual maximum after stress minus baseline T0) as a covariate in all ANOVAs.
3. Results
3.1. Manipulation check
3.1.1. TSST-manipulation check: successful stress induction
To verify successful stress induction by the TSST, we analyzed group differences in temporal fluctuations of (a) salivary cortisol concentrations, (b) α-amylase concentrations, and (c) subjective ratings of negative affect. See for means and standard deviations for each group. (For within-group analyses of the stress parameters, see Supplement 1.)
The 5x2 mixed ANOVA on cortisol data revealed a significant interaction effect ‘time*group’ after controlling for sex, F(4, 176) = 11.34, p < 0.001, η2p = 0.21 (Greenhouse-Geisser corrected, ε = 0.57). Post-hoc t-tests revealed significant group differences at all time points after stress (all p < 0.001), see and .
The 5x2 mixed ANOVA on α-amylase data revealed a significant main effect of ‘time’ after controlling for sex, F(4, 176) = 5.22, p = 0.002, η2p = 0.12 (Greenhouse-Geisser corrected, ε = 0.77). Post-hoc t-tests revealed a significant difference in amylase concentrations between T1 and the other time points, irrespective of group (all p < 0.001), see . No significant interaction effect with group was found.
The 3x2 mixed ANOVA on the PANAS negative mean scores resulted in a significant main effect for ‘time’, F(2, 94) = 4.63, p = 0.019, η2p = 0.09, as well as a significant ‘time*group’ interaction effect after controlling for sex, F(2, 94) = 6.72, p = 0.004, η2p = 0.13 (Greenhouse-Geisser corrected, ε = .79). Post-hoc t-tests revealed a significant group difference at T2, indicating more negative mood ratings in the SG after the TSST, see and .
Figure 3. Manipulation Check: Acute stress reactivity assessed with (a) saliva cortisol concentrations, (b) saliva α-amylase concentrations, and (c) subjective PANAS-ratings of negative affect. PANAS: Positive and Negative Affect Scale (Watson et al., Citation1988); S1-S6: saliva concentrations (untransformed). Error bars represent SEM; *α < 0.05.
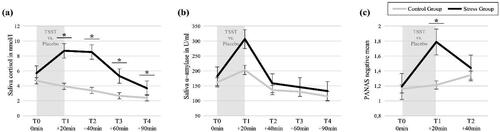
3.1.2. CERT-manipulation check: effective emotion induction and regulation
The 2 × 3 rmANOVA testing for effective emotion induction and regulation in the CERT irrespective of stress yielded a significant main effect of ‘task’, F(2, 49) = 120.30, p < 0.001, η2p = 0.71, indicating (A) successful emotion induction (alias emotional reactivity), i.e. more aversive evaluation of negative compared to neutral pictures and (B) effective downregulation via reappraisal, i.e. more aversive evaluation of negative pictures in view compared to reappraisal trials (see Supplementary Table 3 for means, standard deviations, and statistical indices of post-hoc t-tests). No significant main effect of ‘phase’ or ‘task*phase’ interaction was found. Please find a graphic depiction of these results as well as a manipulation check for the psychophysiological outcome measures in Supplement 2.
3.2. Time-dependent stress effects on emotion regulation
To investigate stress effects on ER, we checked for phase-specific group differences in (1) subjective valence ratings, (2) ERP data, and (3) EMG data, controlling for sex and cortisol increase. (1) For the subjective CERT ratings, the 2 × 2 mixed rmANOVA resulted in a significant ‘phase*group’ interaction effect, F(1, 46) = 5.61, p = 0.022, η2p = 0.11, after controlling for sex and cortisol increase. Post-hoc t-tests indicated a group difference only in the late phase (SG: M(SD) = 1.68 (1.33); CG: M(SD) = 2.47 (1.42); t(48) = −2.02, p = 0.049, Cohen’s d = 0.55), indicating higher reappraisal success in the CG compared to the SG in the late phase, see . (2) We excluded one participant for the EEG analysis (ncontrol = 1) due to excessive data artifacts. The 2 × 2 mixed rmANOVA on the parietal LPP-activity difference score resulted in a significant ‘phase*group’ interaction effect, F(1, 45) = 9.14, p = 0.004, η2p = 0.17, after controlling for sex and cortisol increase. Post hoc t-tests indicated a group difference in the late phase only (SG: M(SD) = 0.56 (3.23); CG: M(SD) = 2.59 (2.86); t(47) = −2.23, p = 0.026, Cohen’s d = 0.31), again indicating higher reappraisal success in the CG compared to the SG in the late phase, see and . (3) Four participants had to be excluded from EMG data analysis (ncontrol = 2; nstress = 2) due to excessive data artifacts or scores >3SD above mean (outlier). The 2 × 2 mixed rmANOVA on the LPP-activity difference score resulted in a significant ‘phase*group’ interaction effect, F(1, 42) = 4.48, p = 0.040, η2p = 0.10, after controlling for sex and cortisol increase. Post hoc t-tests again indicated a significant group difference in the late phase only (SG: M(SD) = −0.17 (3.21); CG: M(SD) = 3.19 (4.65); t(44) = 2.85, p = 0.007, Cohen’s d = 0.78), indicating significantly higher reappraisal success in the CG compared to the SG again only in the late phase, see and . Note that indicates that the interaction effects may result from both, a decrease in reappraisal success in the SG as well as an increase in the CG. See Supplementary Figure 2 for a graphic depiction of the group-specific CERT mean scores on all outcome levels.
Figure 4. Time-dependent stress effects on emotion regulation outcome measures (difference scores): (1) Valence Rating (Reappraisal minus View), (2) EEG activity (View minus Reappraisal), and (3) EMG activity (View minus Reappraisal). Error bars represent SEM; *α < 0.05.
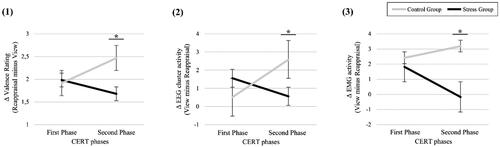
Figure 5. (A) EEG activity to the CERT: ERPs (Pz-electrode) for the early and the late post-stress phase. Note that in the late phase, LPP activity differs between view- and reappraise-trials for the control group only, while no such regulation effect was found for the stress group. (B) EMG activity to the CERT: corrugator activity for the early and late post-stress phase. Note, that again in the late phase, corrugator activity differs between view- and reappraise-trials for the control group only, while no such regulation effect was found for the stress group. Note also, that groups did not differ in emotional reactivity (see Supplement). CERT: Cognitive Emotion Regulation Task; EEG: electroencephalogram; EMG: electromyogram; ERP: event-related potential; LPP: late positive potential.
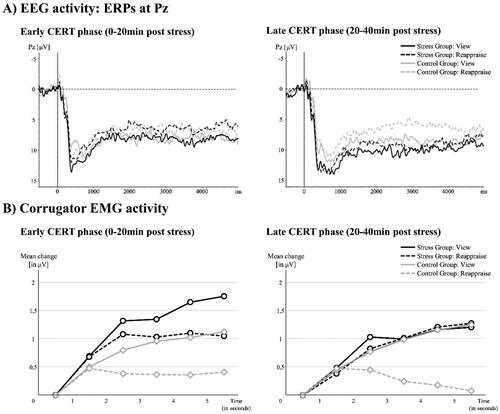
4. Discussion
In light of the ongoing debate about the potential of cognitive reappraisal as a stress management technique, the present study was specifically concerned with investigating whether acute stress could have a negative influence on the reappraisal ability. Importantly, our results provide evidence for a potentially detrimental influence of stress on an individual’s ability to regulate negative emotions through reappraisal. Across all psychophysiological outcome variables examined, we observed reduced reappraisal performance in the stress group relative to the control group in the late experimental phase. This finding may be interpreted as stress-related impairments in cognitive reappraisal, which would be consistent with findings on the neuroendocrine dynamics in the face of a stressor: glucocorticoids and catecholamines have previously been shown to affect the function of the prefrontal cortex (PFC; Arnsten, Citation2009; Hermans et al., Citation2014), parts of which play a key role in successful cognitive reappraisal (Buhle et al., Citation2014). Furthermore, previous research has shown that acute stress may impair PFC-related executive functions such as working memory, cognitive flexibility and cognitive inhibition, i.e. the ability to inhibit thoughts or internal distractors (see Shields et al., Citation2016, for a meta-analysis; see Plieger et al., Citation2020, for a review), which are thought to be crucial for successful cognitive reappraisal (Compas, Citation2006; Hofmann et al., Citation2012; Ochsner et al., Citation2012). Consistent with this, some studies have reported impairments in cognitive ER after acute stress (Kinner et al., Citation2014; Raio et al., Citation2013; Zhan et al., Citation2017). However, these detrimental effects of stress on ER so far seemed to occur in a rather early time window after stress induction, while other studies reported improvements in ER in the longer aftermath of stress induction (Langer et al., Citation2020, Citation2022a, Citation2023) or cortisol administration (Jentsch et al., Citation2019; Langer et al., Citation2022b).
Contrary to these results and to our own hypothesis, we observed impairments in reappraisal only in the late phase whereas in the early phase, groups did not differ. Current microbiological and neuroscientific theories suggest that a stress-related reduction in PFC activity and thus a potential impairment of ER capacity relates to the influence of the immediate early neuroendocrine processes in the face of stress (see Hermans et al., Citation2014, for an overview). A timing aspect of the present study is particularly important here: although our early phase started immediately after the stressor offset, it did not start until 20 minutes after the start of the stressor due to the procedure and timing of the TSST. Assuming that this early stress response starts with the onset of a stressor, this could imply that the concentration of catecholamines were already decreasing during our early ER phase. Our early ER phase could therefore have been too late to catch upon the early detrimental prefrontal processes after stress (see limitations). Consistent with this, it is striking that those studies reporting an impairment of ER after stress (Kinner et al., Citation2014; Raio et al., Citation2013; Zhan et al., Citation2017) all used a significantly shorter stress induction paradigm (i.e. the Cold Pressor Task which lasts about 3 minutes only) and were thus able to examine ER abilities significantly earlier after stress onset, i.e. at a time window when catecholaminergic activity presumably peaks.
However, if the stress processes with the greatest ER impairment potential may have occurred too early for our early ER phase, how can the group differences in the late ER phase be explained, which started about 40 minutes after stress onset? A possible explanation relates to our specific study design, which differs significantly from previous studies in one aspect: with the aim of examining the temporal dynamics in more detail, the participants in our study went through not one but two extensive phases of the CERT task, separated by only a short break of two minutes, which meant a total of 40 minutes of constant regulation effort. Given that participants in the SG had previously undergone a cognitively and psychosocially demanding stress induction procedure, impairments in reappraisal in this group might be primarily associated with greater mental fatigue, particularly in the second half of the task. Indeed, mental fatigue has previously been shown to negatively affect ER capacities (Grillon et al., Citation2015). In addition, a possible increase in mental fatigue, particularly in the stress group, could have affected memory and training processes (Shields, Citation2020). In this respect, a closer look at shows that the interaction effects were not only driven by an impairment in reappraisal in the SG, but also by an improvement in reappraisal in the CG in the later phase. Such an improvement could be explained by reappraisal learning and training effects from the early to the late phase of the experiment in the CG, whereas in the SG stress-related mental fatigue may have disrupted such training processes. For example, Doerr and colleagues observed that momentary stress interacts with fatigue in that both enhance each other mutually (Doerr et al., Citation2015). Furthermore, stress itself is known to directly interfere with and affect learning and memory processes (Shields et al., Citation2016; Wolf et al., Citation2016), and thus may have additionally interfered with any improvement in the use of cognitive reappraisal in the SG. The decisive influence of mental fatigue should be considered both in the design of future studies in this field and in the conception of tailor-made stress management trainings (see below).
Integrating our findings into the larger picture of stress management, the following considerations can be made. Ursu and Măirean (Citation2022) recently reported a positive association between reappraisal and resilience during the COVID-19 pandemic. The authors emphasized the potential utility of targeting cognitive ER strategies in the development and implementation of stress management interventions. However, it appears that not every ER strategy is equally effective in every situation, but its use and benefits rather depends on individual and contextual factors (Ford & Troy, Citation2019). For example, a recent study from our lab suggested that the effectiveness of reappraisal inventiveness depends on the familiarity of the emotional situation (Zeier et al., Citation2023). In this line, our results suggest that reappraisal may well be an effective strategy for dealing with aversive emotions even during or shortly after confrontation with an acute stressor. However, acute stress seems to have a negative impact on the continuous and prolonged use of this cognitively demanding ER strategy in the aftermath of a stressor, as it may be subject to fatigue effects. Distraction may be an effective alternative strategy for long-term ER application, as it is thought to require less cognitive effort than reappraisal and may therefore be less prone to fatigue (Shafir et al., Citation2016; Sheppes & Levin, Citation2013). However, a systematic investigation of the time-dependent effects of distraction is still lacking. Future stress management programs should therefore consider implementing and training a highly flexible application of different ER strategies, depending on timing as well as various other factors yet to be determined (e.g. type of stressor, situational familiarity, etc).
The results of the present study should be considered within the scope of some limitations that relate in particular to the rather small sample size and our study design. In addition to the limited generalizability of our results, the sample size did not allow for a sub-analysis of sex-specific effect, although recent evidence suggests that these may play a decisive role in the stress-ER-interaction: improvements in ER after stress were predominantly found in male participants, where regulation success was associated (Langer et al., Citation2020, Citation2022a, Citation2023) or even fully mediated (Langer et al., Citation2023) by the amount of stress-related cortisol increase. In women, however, higher stress-related sympathetic activation was associated with emotion regulation impairments (Langer et al., Citation2023). We tried to control for this effect by including sex and the individual cortisol increase as covariates in our analyses. However, there is some evidence that stress may affect ER differently in women and men, which has so far been attributed to differences in endocrine concentrations but may also be due to differences in the extent of stress-induced fatigue. This needs to be systematically investigated in follow-up studies before it should be considered in the design of stress management training programs. Further, and also in relation to the constraints of the relatively small within-group sample sizes, we were not able to reliably estimate predictors of individual differences in the dependent variable (here: emotion regulation success), as, for example, stress hormone responses or changes in stress-induced affect (see also Brysbaert (Citation2019) for the discussion of sample sizes for specific statistical analyses).
Another limitation refers to the time windows we chose for performing the ER task. As pointed out earlies, we chose these time windows according to the main study goal, i.e. the detection of possible ER impairments due to acute stress induction, but also in line with some methodological considerations: although the early window was immediately after stressor onset, it was possibly too late to detect catecholamine-driven impairing ER effects (see above), while the late window was certainly too early to investigate cortisol-driven facilitation of prefrontal function including ER. However, EEG-related constraints forced us to limit the length of the ER task windows to minimize the risk of artifacts (e.g. movement, increasing electrode resistances) and resulting low data quality. These caveats should be considered when conducting future studies with different time windows and longer break between task phases, which could reduce the risk of fatigue effects. Further, we did not discriminate between different types of negative emotions, such as fear, anger, or sadness. Due to time constraints, we were not able to include enough stimuli per category to analyze them separately, particularly with respect to event-related potentials. Stress may alter the regulation of some emotions while leaving others rather unaffected. Future studies could take this aspect into account, as this might also be crucial for both training programs as well as clinical interventions.
In summary, we found that stress significantly affects subjective and psychophysiological measures of ER, with stress exposure hampering cognitive reappraisal predominantly during the late phase. Together with recent findings from other groups, the present study suggests that stress-related effects on cognitive ER crucially depend on the timing of applying ER strategies in relation to stress exposure.
Supplemental Material
Download MS Word (553.3 KB)Acknowledgements
We thank Anna Bergmann, Jana Rensland, Johanna Malich and Mike Kirschke for their assistance in data acquisition, subject recruitment and for providing their excellent acting abilities to the TSST. We gratefully acknowledge the subjects who participated in the study.
Disclosure statement
No potential conflict of interest was reported by the author(s).
Additional information
Funding
Notes on contributors
Michèle Wessa
Michèle Wessa, PhD, is a Full Professor in the Department of Clinical Psychology and Neuropsychology at the Johannes Gutenberg-University Mainz with a cross-appointment in the Leibniz-Institute of Resilience Research, Mainz, Germany. Her research program focuses on the neuropsychological mechanisms underlying the etiology of affective disorders, resilience, and mental health in competitive sports.
Magdalena Sandner
Magdalena Sandner, PhD, received her Bachelor’s, MSc, and PhD in Psychology (focus Emotion Regulation and Stress) from the Johannes Gutenberg University (JGU) Mainz, Germany. She is licensed cognitive-behavioral therapist and is currently lecturer in Clinical Psychology and Psychotherapy at JGU, Mainz.
Jérôme Rimpel
Jérôme Rimpel, PhD, received his Bachelor’s in Psychology from the Saarland University, his MSc in Psychology from the University of Bremen and his PhD in Clinical Psychology and Neuropsychology (focus Emotion Regulation and Stress) from the Johannes Gutenberg University Mainz. He is working freelance in the field of clinical psychology and legal psychology.
Sandra Schönfelder
Sandra Schönfelder, MSc, received her Diploma in Psychology from the University Dresden, Germany. She then worked with Michèle Wessa at the Central Institute of Mental Health (Mannheim), Johannes Gutenberg University Mainz and Leibniz Institute of Resilience Research (Mainz) as research associate. She now holds a permanent position in the editorial office of Beltz Verlag.
References
- Aldao, A., Nolen-Hoeksema, S., & Schweizer, S. (2010). Emotion-regulation strategies across psychopathology: A meta-analytic review. Clinical Psychology Review, 30(2), 1–11. https://doi.org/10.1016/j.cpr.2009.11.004
- Arnsten, A. F. T. (2009). Stress signalling pathways that impair prefrontal cortex structure and function. Nature Reviews. Neuroscience, 10(6), 410–422. https://doi.org/10.1038/nrn2648
- Barrett, L. F., Mesquita, B., Ochsner, K. N., & Gross, J. J. (2007). The Experience of Emotion. Annual Review of Psychology, 58(1), 373–403. https://doi.org/10.1146/annurev.psych.58.110405.085709
- Blumenthal, T. D., Cuthbert, B. N., Filion, D. L., Hackley, S., Lipp, O. V., & Van Boxtel, A. (2005). Committee report: Guidelines for human startle eyeblink electromyographic studies. Psychophysiology, 42(1), 1–15. https://doi.org/10.1111/j.1469-8986.2005.00271.x
- Bonanno, G. A., & Burton, C. L. (2013). Regulatory Flexibility: An Individual Differences Perspective on Coping and Emotion Regulation. Perspectives on Psychological Science: a Journal of the Association for Psychological Science, 8(6), 591–612. https://doi.org/10.1177/1745691613504116
- Bradley, M. M., & Lang, P. J. (1994). Measuring emotion: The self-assessment manikin and the semantic differential. Journal of Behavior Therapy and Experimental Psychiatry, 25(1), 49–59. https://doi.org/10.1016/0005-7916(94)90063-9
- Bradley, M. M., & Lang, P. J. (2000). Measuring emotion: Behavior, feeling, and physiology. In R. D. Lane, & L. Nadel (Eds.), Cognitive neuroscience of emotion (pp. 242–276). Oxford University Press.
- Brysbaert, M. (2019). How many participants do we have to include in properly powered experiments? a tutorial of power analysis with reference tables. Journal of Cognition, 2(1), 16. https://doi.org/10.5334/joc.72
- Buhle, J. T., Silvers, J. A., Wager, T. D., Lopez, R., Onyemekwu, C., Kober, H., Weber, J., & Ochsner, K. N. (2014). Cognitive reappraisal of emotion: A meta-analysis of human neuroimaging studies. Cerebral Cortex (New York, N.Y.: 1991), 24(11), 2981–2990. https://doi.org/10.1093/cercor/bht154
- Compas, B. E. (2006). Psychobiological Processes of stress and coping: implications for resilience in children and adolescents–comments on the papers of Romeo & McEwen and Fisher et al. Annals of the New York Academy of Sciences, 1094(1), 226–234. https://doi.org/10.1196/annals.1376.024
- Doerr, J. M., Ditzen, B., Strahler, J., Linnemann, A., Ziemek, J., Skoluda, N., Hoppmann, C. A., & Nater, U. M. (2015). Reciprocal relationship between acute stress and acute fatigue in everyday life in a sample of university students. Biological Psychology, 110, 42–49. https://doi.org/10.1016/j.biopsycho.2015.06.009
- Duan, H., Wang, X., Hu, W., & Kounios, J. (2020). Effects of acute stress on divergent and convergent problem-solving. Thinking & Reasoning, 26(1), 68–86. https://doi.org/10.1080/13546783.2019.1572539
- Faul, F., Erdfelder, E., Buchner, A., & Lang, A.-G. (2009). Statistical power analyses using G*Power 3.1: Tests for correlation and regression analyses. Behavior Research Methods, 41(4), 1149–1160. https://doi.org/10.3758/BRM.41.4.1149
- Feder, A., Nestler, E. J., & Charney, D. S. (2009). Psychobiology and molecular genetics of resilience. Nature Reviews. Neuroscience, 10(6), 446–457. https://doi.org/10.1038/nrn2649
- Ferri, J., Weinberg, A., & Hajcak, G. (2012). I see people: The presence of human faces impacts the processing of complex emotional stimuli. Social Neuroscience, 7(4), 436–443. https://doi.org/10.1080/17470919.2012.680492
- Ford, B. Q., & Troy, A. S. (2019). Reappraisal reconsidered: A closer look at the costs of an acclaimed emotion-regulation strategy. Current Directions in Psychological Science, 28(2), 195–203. https://doi.org/10.1177/0963721419827526
- Foti, D., & Hajcak, G. (2008). Deconstructing Reappraisal: Descriptions preceding arousing pictures modulate the subsequent neural response. Journal of Cognitive Neuroscience, 20(6), 977–988. https://doi.org/10.1162/jocn.2008.20066
- Fridlund, A. J., & Cacioppo, J. T. (1986). Guidelines for human electromyographic research. Psychophysiology, 23(5), 567–589. https://doi.org/10.1111/j.1469-8986.1986.tb00676.x
- Grillon, C., Quispe-Escudero, D., Mathur, A., & Ernst, M. (2015). Mental fatigue impairs emotion regulation. Emotion (Washington, D.C.), 15(3), 383–389. https://doi.org/10.1037/emo0000058
- Gross, J. J., & John, O. P. (2003). Individual differences in two emotion regulation processes: Implications for affect, relationships, and well-being. Journal of Personality and Social Psychology, 85(2), 348–362. https://doi.org/10.1037/0022-3514.85.2.348
- Hajcak, G., Dunning, J. P., & Foti, D. (2009). Motivated and controlled attention to emotion: Time-course of the late positive potential. Clinical Neurophysiology: official Journal of the International Federation of Clinical Neurophysiology, 120(3), 505–510. https://doi.org/10.1016/j.clinph.2008.11.028
- Hajcak, G., MacNamara, A., & Olvet, D. M. (2010). Event-related potentials, emotion, and emotion regulation: An integrative review. Developmental Neuropsychology, 35(2), 129–155. https://doi.org/10.1080/87565640903526504
- Hermans, E. J., Henckens, M. J. A. G., Joëls, M., & Fernández, G. (2014). Dynamic adaptation of large-scale brain networks in response to acute stressors. Trends in Neurosciences, 37(6), 304–314. https://doi.org/10.1016/j.tins.2014.03.006
- Het, S., Rohleder, N., Schoofs, D., Kirschbaum, C., & Wolf, O. T. (2009). Neuroendocrine and psychometric evaluation of a placebo version of the ‘Trier Social Stress Test. Psychoneuroendocrinology, 34(7), 1075–1086. https://doi.org/10.1016/j.psyneuen.2009.02.008
- Hofmann, W., Schmeichel, B. J., & Baddeley, A. D. (2012). Executive functions and self-regulation. Trends in Cognitive Sciences, 16(3), 174–180. https://doi.org/10.1016/j.tics.2012.01.006
- Jackson, D. C., Malmstadt, J. R., Larson, C. L., & Davidson, R. J. (2000). Suppression and enhancement of emotional responses to unpleasant pictures. Psychophysiology, 37(4), 515–522. https://doi.org/10.1111/1469-8986.3740515
- Jentsch, V. L., Merz, C. J., & Wolf, O. T. (2019). Restoring emotional stability: Cortisol effects on the neural network of cognitive emotion regulation. Behavioural Brain Research, 374, 111880. https://doi.org/10.1016/j.bbr.2019.03.049
- Kalisch, R., Müller, M. B., & Tüscher, O. (2015). Advancing empirical resilience research. The Behavioral and Brain Sciences, 38, e128. https://doi.org/10.1017/S0140525X15000023
- Kanske, P., Heissler, J., Schönfelder, S., Bongers, A., & Wessa, M. (2011). How to Regulate Emotion? Neural Networks for Reappraisal and Distraction. Cerebral Cortex (New York, N.Y.: 1991), 21(6), 1379–1388. https://doi.org/10.1093/cercor/bhq216
- Kinner, V. L., Het, S., & Wolf, O. T. (2014). Emotion regulation: Exploring the impact of stress and sex. Frontiers in Behavioral Neuroscience, 8, 397. https://doi.org/10.3389/fnbeh.2014.00397
- Kirschbaum, C., Pirke, K.-M., & Hellhammer, D. H. (1993). The ‘Trier Social Stress Test’ – a tool for investigating psychobiological stress responses in a laboratory setting. Neuropsychobiology, 28(1-2), 76–81. https://doi.org/10.1159/000119004
- Kudielka, B. M., & Kirschbaum, C. (2005). Sex differences in HPA axis responses to stress: A review. Biological Psychology, 69(1), 113–132. https://doi.org/10.1016/j.biopsycho.2004.11.009
- Lang, P. J., Bradley, M. M., & Cuthbert, B. N. (2008). International Affective Picture System (IAPS): Instruction manual and affective ratings. Technical Report A-8. The Center for Research in Psychophysiology, University of Florida.
- Langer, K., Hagedorn, B., Stock, L.-M., Otto, T., Wolf, O. T., & Jentsch, V. L. (2020). Acute stress improves the effectivity of cognitive emotion regulation in men. Scientific Reports, 10(1), 11571. https://doi.org/10.1038/s41598-020-68137-5
- Langer, K., Jentsch, V. L., & Wolf, O. T. (2022a). Acute stress influences strategy preference when dealing with high intensity emotions in men. Biological Psychology, 169, 108264. https://doi.org/10.1016/j.biopsycho.2022.108264
- Langer, K., Jentsch, V. L., & Wolf, O. T. (2022b). Cortisol promotes the cognitive regulation of high intensive emotions independent of timing. The European Journal of Neuroscience, 55(9-10), 2684–2698. https://doi.org/10.1111/ejn.15182
- Langer, K., Jentsch, V. L., & Wolf, O. T. (2023). Rapid effects of acute stress on cognitive emotion regulation. Psychoneuroendocrinology, 151, 106054. https://doi.org/10.1016/j.psyneuen.2023.106054
- Langer, K., Wolf, O. T., & Jentsch, V. L. (2021). Delayed effects of acute stress on cognitive emotion regulation. Psychoneuroendocrinology, 125, 105101. https://doi.org/10.1016/j.psyneuen.2020.105101
- Mauss, I. B., & Robinson, M. D. (2009). Measures of emotion: A review. Cognition & Emotion, 23(2), 209–237. https://doi.org/10.1080/02699930802204677
- McRae, K., & Gross, J. J. (2020). Emotion regulation. Emotion (Washington, D.C.), 20(1), 1–9. https://doi.org/10.1037/emo0000703
- Ochsner, K. N., Silvers, J. A., & Buhle, J. T. (2012). Functional imaging studies of emotion regulation: A synthetic review and evolving model of the cognitive control of emotion: Functional imaging studies of emotion regulation. Annals of the New York Academy of Sciences, 1251(1), E1–E24. https://doi.org/10.1111/j.1749-6632.2012.06751.x
- Pedder, D. J., Terrett, G., Bailey, P. E., Henry, J. D., Ruffman, T., & Rendell, P. G. (2016). Reduced facial reactivity as a contributor to preserved emotion regulation in older adults. Psychology and Aging, 31(1), 114–125. https://doi.org/10.1037/a0039985
- Picton, T. W., Bentin, S., Berg, P., Donchin, E., Hillyard, S. A., Johnson, R., Miller, G. A., Ritter, W., Ruchkin, D. S., Rugg, M. D., & Taylor, M. J. (2000). Guidelines for using human event-related potentials to study cognition: Recording standards and publication criteria. Psychophysiology, 37(2), 127–152. https://doi.org/10.1111/1469-8986.3720127
- Plieger T, Reuter M. (2008). Stress & executive functioning: A review considering moderating factors. Neurobiology of Learning and Memory, 173, 107254. https://doi.org/10.1016/j.nlm.2020.107254.
- Plessow, F., Fischer, R., Kirschbaum, C., & Goschke, T. (2011). Inflexibly Focused under Stress: Acute Psychosocial Stress Increases Shielding of Action Goals at the Expense of Reduced Cognitive Flexibility with Increasing Time Lag to the Stressor. Journal of Cognitive Neuroscience, 23(11), 3218–3227. https://doi.org/10.1162/jocn_a_00024
- Plieger, T., & Reuter, M. (2020). Stress & executive functioning: A review considering moderating factors. Neurobiology of Learning and Memory, 173, 107254. https://doi.org/10.1016/j.nlm.2020.107254
- Raio, C. M., Orederu, T. A., Palazzolo, L., Shurick, A. A., & Phelps, E. A. (2013). Cognitive emotion regulation fails the stress test. Proceedings of the National Academy of Sciences of the United States of America, 110(37), 15139–15144. https://doi.org/10.1073/pnas.1305706110
- Ray, R. D., McRae, K., Ochsner, K. N., & Gross, J. J. (2010). Cognitive reappraisal of negative affect: Converging evidence from EMG and self-report. Emotion (Washington, D.C.), 10(4), 587–592. https://doi.org/10.1037/a0019015
- Sandner, M., Zeier, P., Lois, G., & Wessa, M. (2021). Cognitive emotion regulation withstands the stress test: An fMRI study on the effect of acute stress on distraction and reappraisal. Neuropsychologia, 157, 107876. https://doi.org/10.1016/j.neuropsychologia.2021.107876
- Schönfelder, S., Kanske, P., Heissler, J., & Wessa, M. (2014). Time course of emotion-related responding during distraction and reappraisal. Social Cognitive and Affective Neuroscience, 9(9), 1310–1319. https://doi.org/10.1093/scan/nst116
- Schupp, H. T., Flaisch, T., Stockburger, J., & Junghöfer, M. (2006). Emotion and attention: Event-related brain potential studies. In Progress in Brain Research (Vol. 156, pp. 31–51). Elsevier. https://doi.org/10.1016/S0079-6123(06)56002-9
- Shafir, R., Thiruchselvam, R., Suri, G., Gross, J. J., & Sheppes, G. (2016). Neural processing of emotional-intensity predicts emotion regulation choice. Social Cognitive and Affective Neuroscience, 11(12), 1863–1871. https://doi.org/10.1093/scan/nsw114
- Sheppes, G., & Levin, Z. (2013). Emotion regulation choice: Selecting between cognitive regulation strategies to control emotion. Frontiers in Human Neuroscience, 7, 179. https://doi.org/10.3389/fnhum.2013.00179
- Shermohammed, M., Mehta, P. H., Zhang, J., Brandes, C. M., Chang, L. J., & Somerville, L. H. (2017). Does psychosocial stress impact cognitive reappraisal? behavioral and neural evidence. Journal of Cognitive Neuroscience, 29(11), 1803–1816. https://doi.org/10.1162/jocn_a_01157
- Shields, G. S. (2020). Stress and cognition: A user’s guide to designing and interpreting studies. Psychoneuroendocrinology, 112, 104475. https://doi.org/10.1016/j.psyneuen.2019.104475
- Shields, G. S., Sazma, M. A., & Yonelinas, A. P. (2016). The effects of acute stress on core executive functions: A meta-analysis and comparison with cortisol. Neuroscience and Biobehavioral Reviews, 68, 651–668. https://doi.org/10.1016/j.neubiorev.2016.06.038
- Troy, A. S., & Mauss, I. B. (2011). Resilience in the face of stress: Emotion regulation as a protective factor. In S. M. Southwick, B. T. Litz, D. Charney, & M. J. Friedman (Eds.), Resilience and mental health: Challenges across the lifespan. Cambridge University Press.
- Tso, I. F., Rutherford, S., Fang, Y., Angstadt, M., & Taylor, S. F. (2018). The “social brain” is highly sensitive to the mere presence of social information: An automated meta-analysis and an independent study. PloS One, 13(5), e0196503. https://doi.org/10.1371/journal.pone.0196503
- Ursu, A., & Măirean, C. (2022). Cognitive emotion regulation strategies as mediators between resilience and stress during COVID-19 pandemic. International Journal of Environmental Research and Public Health, 19(19), 12631. https://doi.org/10.3390/ijerph191912631
- Watson, D., Clark, L. A., & Tellegen, A. (1988). Development and validation of brief measures of positive and negative affect: The PANAS scales. Journal of Personality and Social Psychology, 54(6), 1063–1070. https://doi.org/10.1037/0022-3514.54.6.1063
- Webb, T. L., Miles, E., & Sheeran, P. (2012). Dealing with feeling: A meta-analysis of the effectiveness of strategies derived from the process model of emotion regulation. Psychological Bulletin, 138(4), 775–808. https://doi.org/10.1037/a0027600
- Wessa, M., Kanske, P., Neumeister, P., Bode, K., Heissler, J., & Schönfelder, S. (2010). EmoPics: Subjektive und psychophysiologische Evaluation neuen Bildmaterials für die klinisch-bio-psychologische Forschung. Zeitschrift Für Klinische Psychologie Und Psychotherapie, 39(1), 77.
- Wolf, O. T., Atsak, P., De Quervain, D. J., Roozendaal, B., & Wingenfeld, K. (2016). Stress and memory: A selective review on recent developments in the understanding of stress hormone effects on memory and their clinical relevance. Journal of Neuroendocrinology, 28(8). https://doi.org/10.1111/jne.12353
- Zeier, P., Sandner, M., & Wessa, M. (2023). Regulating emotions with experience – the effectiveness of reappraisal inventiveness depends on situational familiarity. Anxiety, Stress, & Coping, 37, 77–85. https://doi.org/10.1080/10615806.2023.2200999
- Zhan, J., Wu, X., Fan, J., Guo, J., Zhou, J., Ren, J., Liu, C., & Luo, J. (2017). Regulating Anger under Stress via cognitive reappraisal and sadness. Frontiers in Psychology, 8, 1372. https://doi.org/10.3389/fpsyg.2017.01372