Abstract
Aberrant functioning of the hypothalamic-pituitary-adrenal (HPA) axis is a hallmark of conditions such as depression, anxiety disorders, and post-traumatic stress disorder. Early-life adversity and genetic variation can interaction to disrupt HPA axis regulation, potentially contributing to certain forms of psychopathology. This study employs a rhesus macaque model to investigate how early parental neglect interacts with a single nucleotide polymorphism within the promoter region of the corticotropin-releasing hormone (CRH-248) gene, impacting the development of the HPA axis. For the initial six months of life, 307 rhesus monkey infants (n = 146 females, n = 161 males) were either reared with their mothers (MR) in conditions emulating the natural environment (control group) or raised without maternal care in groups with constant or 3-hours daily access to same-aged peers (NR). Blood samples collected on days 30, 60, 90, and 120 of life under stressful conditions were assayed for plasma cortisol and adrenocorticotropic hormone (ACTH) concentrations. Findings revealed that NR subjects exhibited a significant blunting of both ACTH and cortisol concentrations. Notably, there was a gene-by-environment interaction observed for ACTH and cortisol levels, with NR subjects with the polymorphism displaying higher ACTH concentrations and lower cortisol concentrations. To the extent that these results generalize to humans, they suggest that early parental neglect may render individuals vulnerable to HPA axis dysfunction, a susceptibility that is modulated by CRH-248 genotype—a gene-by-environment interaction that leaves a lasting developmental signature.
Introduction
Dysregulation of the hypothalamic pituitary adrenal (HPA) axis is a feature of various psychiatric conditions, including depression, anxiety, and post-traumatic stress disorder (Laryea et al., Citation2012; Morris & Rao, Citation2013; Young et al., Citation2016). Early life adversity is a known contributor to such dysregulation, with childhood neglect and abuse often evincing hormonal deviations in the stress-responsive HPA axis, including variation in concentrations in corticotropin-releasing hormone (CRH), adrenocorticotropic hormone (ACTH), and cortisol (Koss & Gunnar, Citation2018). This may be, in part, due to the loss of the critical experience-expectant input typically provided by sensitive attachment figures during early development, and facilitating stress reduction, as well as the development of self-regulation (Bick & Nelson, Citation2017; Greenough et al., Citation1987). Failure to meet experience-expectant demands leads to psychological disturbances, dysregulated HPA axis activity and increased vulnerability to psychopathology (Bloch et al., Citation2007; Bunea et al., Citation2017; Carpenter et al., Citation2004; Citation2009; Gunnar et al., Citation2009; Hengesch et al., Citation2018; Ouellet-Morin et al., Citation2011; Tyrka et al., Citation2008). For the HPA system to function appropriately, age-appropriate, experience-expectant input is critical (Greenough et al., Citation1987). Natural selection prefers mothers that respond sensitively to their infants’ needs to assure that their experience-expectant needs are met. Abuse, or impoverished environments are associated with enduring HPA axis dysfunction (Carrion et al., Citation2002; Koss et al., Citation2014), resulting in phenotypically blunted stress responses and reduced cortisol (Bernard et al., Citation2015; Bruce et al., Citation2009; Karlamangla et al., Citation2019; Kuras et al., Citation2017; McLaughlin et al., Citation2015). Conversely, secure attachment bonds enable appropriate behavioral and HPA axis response to stress.
While many experience substantial downstream effects from early neglect and abuse (Nelson et al., Citation2020), some exhibit resilience (Daskalakis et al., Citation2013; Santarelli et al., Citation2017), suggesting potential gene-by-environment interactions (Battaglia, Citation2013; Claessens et al., Citation2011); [hereafter GxE]. CRH is a central orchestrator in the neuroendocrine response to stress, acting as a key mediator in the cascade leading to ACTH and cortisol release. Given CRH’s pivotal role in initiating the HPA axis response (Erickson et al., Citation2005), CRH genes are key candidates for understanding individual variations in stress reactivity. Furthermore, CRH genes exhibit significant variation, including single nucleotide polymorphisms (SNPs), which are associated with HPA axis variation (Barr et al., Citation2009) and risk for psychopathological outcomes (Keck et al., Citation2008; Ressler et al., Citation2010; Smoller et al., Citation2003; Citation2005; Wolf et al., Citation2013). For example, SNPs affecting CRH binding capacity (CRHBP) lead to blunted cortisol responses to pain (Ersig et al., Citation2019), while SNPs in CRH receptor 1 (CRHR1) are linked to elevated cortisol responses to stress (Sheikh et al., Citation2013).
Nonhuman primates serve as valuable models for studying the long-term effects of adverse early experiences . Their closely-controlled environments, similar social needs, and genetic similarities to humans make them ideal for studying the impact of adverse early experiences such as parental neglect on HPA axis development (Sanchez, Citation2006). Conditions in which subjects are reared without their mothers but with access to same-aged peers (nursery rearing; NR) lead to increased anxiety-like behavior and aberrant HPA axis responses (Corcoran et al., Citation2012; Higley et al., Citation1992). For example, comparisons between mother-reared (MR) subjects and NR subjects show that MR subjects exhibit an HPA system that is more sensitive to stress with both a rapid increase in HPA activity and a more rapid return to baseline; whereas, subjects neglected or reared in impoverished environments are more likely to show a blunted response to stress as well as blunted diurnal rhythms (Engel & Gunnar, Citation2020; Higley et al., Citation1992; Shannon et al., Citation1998).
This study is part of a long-term investigation of developmental outcomes in rhesus monkeys. This longitudinal study is designed to characterize subjects’ temperament under normative resting conditions and following stress. Previous papers from this group show that early life temperament as well as social separation stress-induced cortisol predicts alcohol intake in adolescence and young adulthood (Fahlke et al., Citation2000; Wood et al., Citation2020), and led to the identification of a low frequency functional SNP in the rhesus monkey CRH promoter region (248 C > T). This variant in part accounts for overall HPA axis variation and results in alterations in ACTH and cortisol release (Barr et al., Citation2009). Variation in the CRH-248 genotype was associated with increased percent change in ACTH output and reduced percent change in glucocorticoid sensitivity in juvenile and adult rhesus macaques (Barr et al., Citation2008; Citation2009; Ferguson et al., Citation2012). Importantly, CRH-248 genotype effects occurred only within specific contexts or after early adverse experiences, such as early maternal abuse or parental neglect (NR; peer or surrogate peer rearing) (Sanchez, Citation2006; Sánchez et al., Citation2005). It remains unclear as to whether the HPA-axis modulating effects of CRH-248 genetic variation are detectable earlier in life, which if so, would provide important information concerning both normative and pathological development in rhesus monkey infants.
While previous research highlights the importance of GxE interactions in shaping stress responses in juveniles and adults, this study aims to address critical gaps in current research by genotyping neonate and young infant rhesus monkeys for CRH-248 variation. Identifying how early life experiences, particularly early adverse experiences, interact with genetic risk to produce stress response variation during critical developmental periods will provide valuable insight into early vulnerability to HPA axis dysfunction. By examining ACTH and cortisol concentrations as key indicators of stress responsiveness, this research contributes to a nuanced understanding of how variation in the CRH-248 gene may modulate the impact of early experiences on hormonal regulation. The infants in the study were reared either with their mothers in social groups approximating the natural rhesus monkey social environments, or without mothers in peer-only groups with access to only agemates. Previous studies show that early adverse rearing experiences have strong effects on behavior, physiology, and functioning (Zhang, Citation2017). As parental neglect’s impact on the HPA axis is more pronounced under stress (Bunea et al., Citation2017), in this study we hypothesize that NR subjects with the CRH-248 C > T SNP will exhibit pathological HPA axis stress responses (blunted ACTH and cortisol) early in life, when compared to NR subjects with the CC genotype or MR subjects with either the CC genotype or the C > T SNP.
Materials and methods
Subjects
Subjects were 307 rhesus macaques (Macaca mulatta; n = 146 females, n = 161 males) born between 1991 and 2003 and housed at the Laboratory of Comparative Ethology National Institutes of Child Health and Human Development rhesus monkey colony. As part of an ongoing longitudinal study assessing the long-term effects of early rearing on socioemotional and HPA axis development, baseline and challenge samples were obtained during the neonatal and infancy period. Subjects were reared in one of two conditions. Briefly, mother-reared subjects (MR; n = 175) were raised by their biological mothers in social groups approximating the natural conditions seen in the wild, with eight-to-14 adult females with infants, and one-to-two adult males. Nursery-reared (NR) subjects (n = 132) were separated from their mothers at birth and placed in an incubator where they were hand fed for the first 14 days. From days 15 to 37, they were placed in a neonatal nursery where they lived in single cages with a terrycloth-covered, rocking surrogate. Infants fed from a bottle attached to the surrogate. At 37 days of age, subjects either remained in these surrogate conditions (SPR; n = 47), with limited access to other age-mates (2 hours/day, 5-days/week) or they were placed in a group cage with three other age-mates with which they had continuous contact (peer-reared [PR]; n = 85). PR subjects were hand-fed a 50:50 mixture of Similac™ (Ross Laboratories, Columbus, Ohio) and Primalac™ (Bio-Serv, Frenchtown, NJ) formula every 2 hours until 30 days of age when they could self-feed. Thereafter, until four months of age, 400 mL of Primalac™ was provided, supplemented with the essential amino acid docosahexaenoic acid to more closely approximate MR subjects’ milk (Champoux et al., Citation2002). Standard high-protein monkey chow was gradually introduced to the diet, and the infants were provided ad libitum access to water. MR subjects lived in indoor/outdoor runs, and the NR subjects lived in an area with large windows and were thus maintained under an ambient seasonal day/night cycle, with external lights turned on at 0700 hours. Both groups were provided Purina High Protein monkey chow (#5038) ad libitum. NR subjects were socialized each day with 3 other subjects born at approximately the time and both the NR and MR subjects were randomly assigned to social groups. All MR infants from each birth cohort were studied thus assuring a random sample of infants from high- and low-ranking mothers. All procedures for this study were reviewed and approved by the Institutional Animal Care and Use Committee of the National Institute on Alcohol Abuse and Alcoholism.
At 30, 60, 90, and 120 days of life, infants were captured, and blood samples were obtained. The cortisol and ACTH samples were taken under both resting (Day-60) and social separation stress conditions (Days-30, −90, −120) as part of the parent study’s goal of characterizing subjects’ stress responsiveness. Identical procedures were used across the decade: infants were hand captured 5 minutes after the animal handlers entered the room, and blood samples were taken from the femoral vein under restraint without anesthesia. To assure that other variables did not affect ACTH or cortisol levels, animal care and nursery staff did not enter the housing or nursery areas beginning one hour prior to initiating sampling procedures. The day-30 sample was taken following 30 minutes of handling by an experimenter assessing infant temperament and reflexes (Schneider & Suomi, Citation1992). Day-60 samples were taken immediately following capture to serve as a nonstress sample. Day-90 and −120 samples were taken following 30 minutes of separation and isolation in a sound-attenuated room. All samples were obtained between 1300 and 1400 hours.
Cortisol and ACTH assays
Blood samples were obtained using EDTA coated vacutainers, aliquoted into cryogenic tubes, quick frozen in liquid nitrogen, and stored in a −70 C° freezer until they were assayed. Assays were performed within six months following collection, using radioimmunoassays to determine concentrations of plasma ACTH and cortisol, as previously described (Barr et al., Citation2009). Assays were performed in duplicate, and the inter- and intra-assay coefficients of variation were all less than 12%.
CRH-248 genotyping
DNA was isolated from the whole blood using standard extraction methods. Genotyping procedures are described in detail elsewhere (Barr et al., Citation2009). Briefly, genotyping was performed by the 5′ exonuclease method using fluorescent, allele-specific MGB probes. Oligonucleotide primers (Forward: 5′ –GGH CTT TCA TAG TAA GAG GTC AAT ATG T-3′; Reverse: 5′- CGC CTC TTG ACG TCA A-3′) and probe sets (-248 C, 6FAM-TCA TAA GAA GCC CTT CCA TT and −248 T, VIC-GTC ATA AGA AGCTCT TCC ATT) were designed based on the rhesus macaque sequence, with an overall error rate of 0.5%. Genotype frequencies were CC (n = 293) and CT (n = 14).
Statistical analyses
Due to low sample volumes and assay error, some subjects had missing data. To maintain a sufficiently large sample size for statistical analyses, in instances where data were missing (fewer than 5%), single data points were replaced using linear interpolation. Subjects with available data from at least three out of four timepoints were identified for linear interpolation using SPSS statistics procedures. All interpolation was conducted for identified subjects within their respective rearing condition and genotype. Subjects with more than two missing measures were excluded from analyses.
The relationship between CRH-248 genotype and rearing condition on plasma ACTH and cortisol concentrations over the first four months of life was examined. A small portion of the subjects from this study were included in a previous report examining the response to stress in juvenile subjects (Barr et al., Citation2009). In this study we include over double the number of subjects and investigate an earlier developmental period. In this study, we initially examined the breakdown of the genotype frequencies per early rearing condition, with the distribution of the CT genotype by rearing group was as follows: MR: n = 8, PR: n = 4, SPR: n = 2. Preliminary analyses showed no significant differences between PR and SPR subjects’ levels of ACTH, or cortisol (p > 0.100). Thus, PR and SPR subjects were grouped together as NR subjects for all formal analyses (NR subjects with CT genotype: n = 6). Detailed distribution of the subjects per rearing group can be found in . As initial analyses showed that Day-90 and Day-120 cortisol and ACTH levels were highly correlated within each hormone (ACTH: r = .707, p < .001; cortisol: r = .682, p < .001), these time points were averaged to create a Day-90-120 Average (see Supplemental Tables 1 and 2). As is the case with many hormone studies, the data were not normally distributed; however, we note that studies indicate that analysis of variance (ANOVA) is robust to violations of normality (Blanca Mena et al., Citation2017). Formal models included two separate mixed-design, repeated measures ANOVAs, with rearing condition (MR or NR) and CRH-248 genotype as the independent variables and ACTH or cortisol concentrations at Day-30, Day-60, and Day-90-120 Average as the three repeated measures time variables. Within- and between-subject a posteriori comparisons were made to determine significant differences. All analyses were conducted in SPSS, version 29.
Table 1. Sample characteristics.
Heritability
The colony from which subjects derive is outbred, with an average identity-by-descent of 1.86%. This is approximately equivalent to third cousins, who share 0.78% of their DNA (Newman et al., Citation2005; Robin et al., Citation1997). To verify that the effects reported here are related to CRH-248 variation and not to general heritability of HPA axis response, the heritability (h2) of all dependent variables was calculated using Sequential Oligogenic Linkage Analyses Routines (SOLAR version 2.0.7 (Almasy & Blangero, Citation1998)), a software that uses variance components analysis to partition total phenotypic variance of a trait into genetic and environmental components. Results showed that h2 values for all dependent variables were non-significant (ACTH values ranged from 0.00-0.04 and cortisol values ranged from 0.00-0.29).
Results
ACTH concentrations
Results indicated a significant main effect of rearing on ACTH concentrations (F(1,301) = 3.90, p = .049), with NR subjects exhibiting lower ACTH concentrations (M = 242.81 ± 14.26 pmol/mL), when compared to MR subjects (M = 262.07 ± 12.35 pmol/mL). There was a significant main effect of CRH-248 genotype on ACTH concentrations (F(1,301) = 14.58, p < .001), with subjects with the CC genotype exhibiting lower ACTH concentrations (M = 207.42 ± 4.05 pmol/mL), when compared to subjects with the CT genotype (M = 279.46 ± 18.43 pmol/mL). There was also a significant main effect of time on ACTH concentrations (F(1,301) = 27.27, p < .001), with Day-60 ACTH concentrations significantly lower than Day-30 and Day-90-120 and Day-30 significantly lower than Day-90-120 (Day-30: M: 258.94 ± 15.22 pmol/mL, Day-60: 116.90 ± 5.23 pmol/mL, Day-90-120: 354.48 ± 17.26 pmol/mL).
There was also a statistically significant rearing-by-genotype interaction (F(1,301) = 8.69, p = .003), with NR CC subjects exhibiting lower concentrations of ACTH (M = 16.99 ± 6.13 pmol/mL), when compared to MR CC subjects (M = 253.85 ± 5.28 pmol/mL; p < .001) and to NR CT subjects (M = 288.64 ± 27.86 pmol/mL; p < .001) and to MR CT subjects (M = 270.28 ± 24.13 pmol/mL; p < .001). MR subjects’ ACTH concentrations were undifferentiated by genotype (p = .51) and there was a not a significant difference in ACTH concentrations between MR CC subjects and NR CT subjects (p = .22) or between MR CT and NR CT subjects (p = .62). See and Supplemental Figure 1.
Figure 1. There were significant rearing and CRH-248 genotype effects on ACTH concentrations, with NR subjects exhibiting lower ACTH concentrations when compared to MR subjects (p <.049), and CC subjects exhibiting lower ACTH concentrations, when compared to CT subjects (p <.001). There was a rearing-by-CRH-248-genotype interaction, with NR CC subjects exhibiting lower ACTH concentrations, when compared to MR CC subjects (p =.003) and to NR CT subjects (p <.001) and to MR CT subjects (p <.001). MR subject’s ACTH concentrations were undifferentiated by genotype (p =.051) and there was not a significant difference in ACTH concentrations for MR CC and NR CT subjects (p =.22) or between MR CT and NR CT subjects (p =.62).
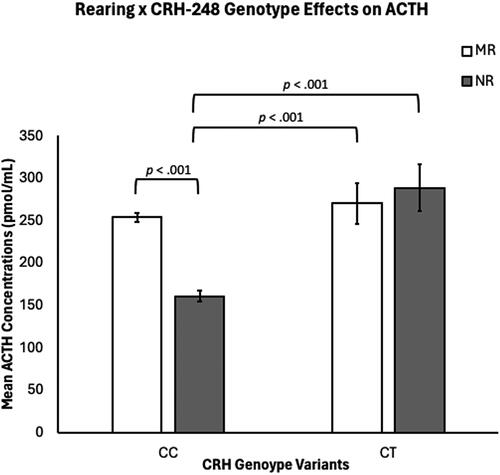
Cortisol concentrations
Results indicated a statistically significant main effect of rearing on cortisol concentrations (F(1,286) = 17.13, p < .001), with NR subjects exhibiting lower cortisol concentrations (M = 36.54 ± 1.663 pmol/mL), when compared to MR subjects (M = 45.65 ± 1.44 pmol/mL). There was not a main effect for CRH-248 genotype on cortisol concentrations (F(1,301) = 0.11, p > .05). There was not a significant main effect of time on cortisol concentrations (p > .05).
There was a significant rearing-by-genotype interaction (F(1,286) = 5.14, p = .024), with NR CC subjects exhibiting significantly lower concentrations of cortisol (M = 39.39 ± 0.73 pmol/mL), when compared to MR CC subjects (M = 43.52 ± 0.64 pmol/mL; p < 0.001). NR CT subjects exhibited significantly lower cortisol concentrations (M = 33.68 ± 3.25 pmol/mL), when compared to MR CT subjects (M = 47.78 ± 2.81 pmol/mL; p = .001) and MR CC subjects (p = .003). NR CC subjects also exhibited significantly lower cortisol concentrations than MR CT subjects (p = .01). NR CT subjects also exhibited trending lower cortisol concentrations, when compared to NR CC subjects (p = .08). MR subjects’ cortisol concentrations were undifferentiated by genotype (p = .16). See and Supplemental Figure 2.
Figure 2. There were significant rearing effects on cortisol concentrations (p <.001), with NR subjects exhibiting lower cortisol concentrations, when compared to MR subjects. There was not a main effect for CRH-248 genotype on cortisol concentrations (p >.05). There was a rearing-by-CRH-248-genotype interaction (p =.024), with NR CC subjects exhibiting lower cortisol concentrations, when compared to MR CC subjects (p <.001). NR CT subjects exhibited lower cortisol concentrations, when compared to MR CT subjects (p =.001) and MR CC subjects (p = .003). NR CT subjects also exhibited lower cortisol concentrations than MR CT subjects (p = .01) and their cortisol concentrations trended lower, when compared to NR CC subjects (p = .08). MR subjects’ cortisol concentrations were undifferentiated by genotype (p >.05).
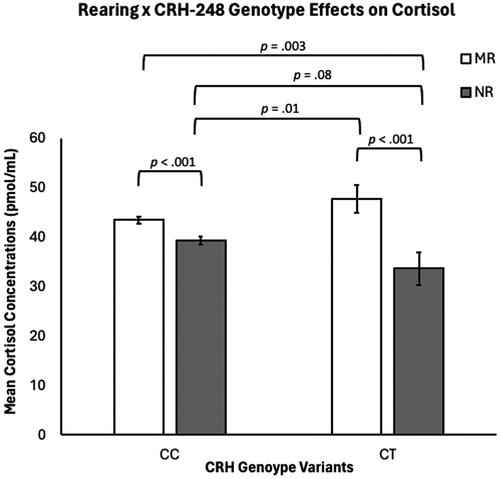
Discussion
For cortisol, we found robust support for our hypotheses. Subjects reared without parents (i.e., NR subjects) exhibited attenuated levels of plasma cortisol, on average. Moreover, these early rearing effects on cortisol were modulated by CRH-248 genotype, demonstrating a compelling GxE interaction. These findings largely align with existing evidence showcasing that adverse rearing experiences in rhesus macaques result in atypical cortisol profiles (Fahlke et al., Citation2000; Higley et al., Citation1992; Shannon et al., Citation1998). Further corroborating our results are other studies showing that unfavorable rearing conditions result in blunted cortisol (Barr et al., Citation2009; Barrett et al., Citation2009; Fahlke et al., Citation2000; Sánchez et al., Citation2005). Comparable patterns emerge from human studies involving Romanian orphans, which share resemblances with our NR cohort (Gunnar et al., Citation2001). The blunted mean cortisol levels observed in NR infants echo findings from another laboratory, where NR infant rhesus macaques exhibited lower than average cortisol in comparison to their MR counterparts, indicating that parental care is crucial in the development of normative cortisol response (Capitanio et al., Citation2005; Kinnally & Capitanio, Citation2015). Studies show that a shallow diurnal cortisol slope following adoption is a hallmark of institutionalized children and predict future behavioral problems (Koss et al., Citation2014). It is of note that other studies show that the effects of early rearing experiences on the cortisol response can extend across multiple generations (Kinnally & Capitanio, Citation2015; Sproul Bassett et al., Citation2020). These collective findings elucidate the influence of adverse rearing conditions on basal and stress-induced cortisol levels, but our findings emphasize the need to consider genotype when assessing the impact of early rearing on the cortisol response.
For cortisol and ACTH concentrations, MR subjects were not differentiated by genotype, a finding that parallels those seen in other studies where mother-rearing has a protective effect from the deleterious effects of relatively rare, non-ancestral genotypic variants (Bennett et al., Citation2002). A main effect for ACTH concentrations revealed that NR infants showed blunted plasma ACTH, compared to MR infants. This mean rearing difference was largely explained by the lower ACTH levels in the NR infants with the CC genotype. NR infants with the CC genotype showed substantial blunting of their ACTH response, consistent with our hypothesis. Inconsistent with our hypothesis, however, infants with the CT genotype did not differ in mean ACTH values when compared to either MR genotype group, and NR subjects with the CT genotype exhibited double the ACTH levels compared to the NR CC subjects. There appears to be an HPA axis response fractionization as the NR CT genotypic effects on cortisol were blunted, whereas the NR CT genotype effects on ACTH were elevated. While our prior study of juveniles did not look at resting or absolute values for ACTH (Barr et al., Citation2009), the stress-induced percent change in ACTH was higher in the NR CT subjects when compared to the NR CC homozygotes. Thus, our ACTH findings are unexpected and represent an area for subsequent study.
It should be noted that it is unlikely that the differences between the MR and NR subjects are a simple result of habituation to human handling. Regardless of early rearing experiences, social separation is a potent stressor resulting in significant distress and HPA activation (Capitanio et al., Citation2005; Higley et al., Citation1992). Due to its stress-inducing effects, social separation is one of the most commonly used methods of inducing stress, including in humans (Ainsworth, Citation1979; Bowlby, Citation1969). NR monkeys form strong bonds with a specific “best buddies” and respond to stress with distress vocalizations and other stress related behaviors (Higley et al., Citation1992). While in the nursery, the NR subjects are removed from the nursery cage each day for weighing until they are 30 days of age. After Day-30, they are no longer handled by humans, except for the monthly blood draws described in the manuscript, and both groups receive identical treatment.
Our results show that the effects of CRH-248 genotypic variation on mean ACTH and cortisol levels is modulated by early rearing experiences (GxE interaction) and suggest that it emerges earlier in life than previously studied, manifesting differences as early as the neonatal period. Independent of genotype, the NR subjects showed blunted cortisol, which is also consistent with previous research in nonhuman primates showing that MR subjects exhibit a more responsive HPA axis than NR subjects (Capitanio et al., Citation2005). This finding parallels the now-classic rodent studies showing that handled rats (a parallel to normative rodent maternal stimulation) show a more responsive system (Levine, Citation1962). It also parallels findings that children reared in impoverished institutional settings show a blunted cortisol response (Engel & Gunnar, 2020). Taken together, these findings suggest that early sensitive parental responsiveness sculpts the HPA axis, often leading to a more sensitive response to stress.
To the extent that blunted cortisol represents a dysregulated, less responsive system, our findings also suggest that, at least for the cortisol response, rearing with a mother serves a protective effect, allowing a more responsive stress response, as is evident when the cortisol levels of MR CT genotype subjects are compared to the NR CT subjects. This parallels studies comparing the effects of rearing on other genotypes (e.g., 5-HTTLPR) (Barr et al., Citation2004; Bennett et al., Citation2002), where MR infants remain undifferentiated by genotype. As emphasized in the introduction, evolution has selected for primary attachment figures to provide an infant’s experience-expectant brain particular input. Mothers and other sensitive caregivers play a vital role in developing a stress response and are central to the development of self-regulation and stress-coping abilities (Bick & Nelson, Citation2017; Greenough et al., Citation1987). Our findings indicate that genetic variation should also be considered when assessing rearing experience effects on the HPA axis response. To the extent that our results generalize to humans, they imply that infants reared without such nurturing inputs are susceptible to developing aberrant HPA axis regulation. Alternatively, our findings suggest that maternal absence (i.e., nursery rearing), may function as a traumatic or adverse life experience that contributes to pathological HPA axis functioning, as observed in studies investigating the effect of childhood trauma on the stress response (Lee et al., Citation2005; Schäfer et al., Citation2010).
For both cortisol and ACTH, MR infants were undifferentiated by genotype, signifying the ameliorative role of maternal presence in dampening the CRH-248 genotype’s effect on the HPA axis response. In the absence of mothers (NR), infants’ genotype exerted a strong effect on the HPA axis response. NR infants with the CC genotype displayed significantly lower mean levels of ACTH than the NR infants with the CT genotype, as well as CC and MR CT infants. For cortisol, NR infants with the CT genotype exhibited lower mean cortisol than MR CT and MR CC subjects and the cortisol concentrations of NR CT subjects trended lower, compared to the NR CC subjects. NR infants with the CC genotype demonstrated markedly lower mean cortisol concentrations than MR infants, regardless of their genotype. These findings accentuate the interaction between early maternal presence/absence and the CRH-248 genotype in shaping HPA axis activity. This study investigated the impact of the CRH-248 genotype on HPA axis functioning, echoing studies showing the effects of early rearing condition and serotonin transporter genotype on CNS serotonin functioning (Bennett et al., Citation2002). While this study offers valuable insights into the association between CRH-248 and early rearing condition on HPA axis development, it should be considered a pilot study, as the CT genotype is relatively uncommon. Caution should be exercised when extrapolating these results and replication should be a priority, particularly when considering the relative rarity of the minor allele (approximately 5%). The interpretations of these findings are also limited by our inability to assess sex differences due to low power.
Future research should strive to disentangle the distinction between the benefits conferred by maternal presence and the effects of adverse early caregiving experiences. For example, one interesting future direction could adopt methods used by Coplan and colleagues, who reared infants with their mothers in variable foraging demand rearing conditions where mothers’ capabilities to care for their infant was unpredictable because food availability varied from week to week (Coplan et al., Citation2011; Citation2014). Future G x E studies could use such paradigms to differentiate the effect of maternal presence and the effects of a maternal stressor, or comparisons could be made using infants that are abused by their mothers.
Conclusion
We observed a significant interaction of CRH-248 genotype and early rearing conditions, exerting an effect on plasma concentrations of ACTH and cortisol. The overall sample size is large for a primate study. Nevertheless, the CT genotype was relatively uncommon. Consequently, our findings should be considered pilot in nature, prompting a call for in-depth exploration of longitudinal CRH-248 variant outcomes in relation to specific HPA axis stress-linked behaviors and anxiety disorders.
Author contributions
E.K.W., S.A.A., P.H.O., and J.D.H. conceptualized the study and wrote the original manuscript. E.K.W. and S.A.A. analyzed and interpreted data. E.K.W. and N.G. prepared the figures. P.H.O., E.H., A.N.S., E.S., M.J., Z.B., and N.G. assisted with review and editing of the manuscript. M.L.S. and S.G.L. collected the data and M.L.S. managed the data files. C.S.B. performed the genotyping, with the assistance of S.G.L. J.D.H., S.J.S., and C.S.B. oversaw the rearing of the subjects and the data collection and oversaw the project. Authors Wood and Aston contributed equally to this work.
Supplemental Material
Download Zip (367.9 KB)Acknowledgements
The authors wish to thank Jayde Shackett and the students who assisted in data collection and care of the nursery-reared subjects. We also express our appreciation to the animal care staff who cared for the subjects.
Disclosure statement
No potential conflict of interest was reported by the author(s).
Additional information
Funding
Notes on contributors
Elizabeth K. Wood
Elizabeth K. Wood, PhD, is a developmental psychobiologist in the Psychiatry Department at Oregon Health & Science University. Her research focuses on the biological and environmental contributors to mental health and well-being, particularly through identifying the prenatal and emerging origins of mental health concerns.
S. Andrew Aston
S. Andrew Aston is a PhD candidate in Cellular and Molecular Medicine at Johns Hopkins University School of Medicine studying behavioral neuroscience and, specifically, the effects of maternal diet on dopamine release and reward processing in offspring.
Patrick H. O’Connell
Patrick H. O’Connell, MD is a psychiatry resident at Emory University with interests in psychosomatic medicine and neuromodulation.
Elia Hafen
Elia Hafen is an undergraduate student studying Psychology at Brigham Young University. Her research interests include early environmental contributions to development.
Andrea N. Skowbo
Andrea N. Skowbo holds an MS in Psychology from Brigham Young University. Her research interests include evaluating the role of genes and early life experience on long-term developmental outcomes, including risk for alcohol abuse.
Melanie L. Schwandt
Melanie L. Schwandt, PhD, is a Staff Scientist in the Office of the Clinical Director, National Institute on Alcohol Abuse and Alcoholism. Her research interests include the effects of early adverse experiences and stress on risk for, and resilience to, alcohol-related behavioral and neurobiological phenotypes in humans.
Stephen G. Lindell
Stephen G. Lindell, MS, is a biologist with the National Institute on Alcohol Abuse and Alcoholism. His work includes identifying genetic variants predicting stress resilience and alcohol consumption in rhesus macaques.
Ellie Smith
Ellie Smith, BS, holds a degree in Psychology from Brigham Young University. Her research focuses on the relationship between mother-infant attachment and the development of psychopathology.
Miranda Johnson
Miranda Johnson holds a BS in Neuroscience and Psychology from Brigham Young University. Her research interests focus on examining and identifying the biopsychosocial factors across the lifespan that contribute to the risk and development of mental illness.
Zachary Baron
Zachary Baron holds an MS in Neuroscience from Brigham Young University. Zachary is interested in behavioral genetics.
Natalia Gabrielle
Natalia Gabrielle, BS Psychology, is a behavioral and neurodevelopmental researcher at Oregon Health & Science University’s Oregon National Primate Research Center. Their research currently focuses on behavioral, cognitive, and neurodevelopment of infant rhesus macaques with or without prenatal alcohol exposure.
Christina S. Barr
Christina S. Barr, PhD, studies functional genetic variants affecting reward sensitivity and stress reactivity in nonhuman primates, examining how stress impacts epigenetic regulation and gene expression in the brain, particularly in the context of gene-environment interactions.
Stephen J. Suomi
Stephen J. Suomi, PhD, is a psychobiologist investigating how genetic and environmental factors influence behavioral and physiological outcomes. His research focuses on the role of early social experiences and maternal care in shaping emotional and social development and the impact of stress and change on development.
David Goldman
David Goldman, MD, Clinical Director and Chief of the Lab of Neurogenetics at National Institute on Alcohol Abuse and Alcoholism, National Institutes of Health, is a geneticist identifying functional loci that predispose to psychiatric disorders and secondary pathologies.
J. Dee Higley
J. Dee Higley, PhD, is a professor emeritus of Psychology and Neuroscience at Brigham Young University. His research targets on serotonin-mediated differences in temperament and risk for developmental psychopathology, focusing on gene by environment interactions.
References
- Ainsworth, M. S. (1979). Infant–mother attachment. American Psychologist, 34(10), 932–937. https://doi.org/10.1037/0003-066X.34.10.932
- Almasy, L., & Blangero, J. (1998). Multipoint quantitative-trait linkage analysis in general pedigrees. American Journal of Human Genetics, 62(5), 1198–1211. https://doi.org/10.1086/301844
- Barr, C. S., Dvoskin, R. L., Gupte, M., Sommer, W., Sun, H., Schwandt, M. L., Lindell, S. G., Kasckow, J. W., Suomi, S. J., Goldman, D., Higley, J. D., & Heilig, M. (2009). Functional CRH variation increases stress-induced alcohol consumption in primates. Proceedings of the National Academy of Sciences of the United States of America, 106(34), 14593–14598. https://doi.org/10.1073/pnas.0902863106
- Barr, C. S., Dvoskin, R. L., Yuan, Q., Lipsky, R. H., Gupte, M., Hu, X., Zhou, Z., Schwandt, M. L., Lindell, S. G., McKee, M., Becker, M. L., Kling, M. A., Gold, P. W., Higley, D., Heilig, M., Suomi, S. J., & Goldman, D. (2008). CRH haplotype as a factor influencing cerebrospinal fluid levels of corticotropin-releasing hormone, hypothalamic-pituitary-adrenal axis activity, temperament, and alcohol consumption in rhesus macaques. Archives of General Psychiatry, 65(8), 934–944. https://doi.org/10.1001/archpsyc.65.8.934
- Barr, C. S., Newman, T. K., Lindell, S., Shannon, C., Champoux, M., Lesch, K. P., Suomi, S. J., Goldman, D., & Higley, J. D. (2004). Interaction between serotonin transporter gene variation and rearing condition in alcohol preference and consumption in female primates. Archives of General Psychiatry, 61(11), 1146–1152. https://doi.org/10.1001/archpsyc.61.11.1146
- Barrett, C. E., Noble, P., Hanson, E., Pine, D. S., Winslow, J. T., & Nelson, E. E. (2009). Early adverse rearing experiences alter sleep-wake patterns and plasma cortisol levels in juvenile rhesus monkeys. Psychoneuroendocrinology, 34(7), 1029–1040. https://doi.org/10.1016/j.psyneuen.2009.02.002
- Battaglia, M. (2013). Gene-environment interaction in panic disorder and posttraumatic stress disorder. Canadian Journal of Psychiatry. Revue Canadienne de Psychiatrie, 58(2), 69–75. https://doi.org/10.1177/070674371305800202
- Bennett, A. J., Lesch, K. P., Heils, A., Long, J. C., Lorenz, J. G., Shoaf, S. E., Champoux, M., Suomi, S. J., Linnoila, M. V., & Higley, J. D. (2002). Early experience and serotonin transporter gene variation interact to influence primate CNS function. Molecular Psychiatry, 7(1), 118–122. https://doi.org/10.1038/sj.mp.4000949
- Bernard, K., Zwerling, J., & Dozier, M. (2015). Effects of early adversity on young children’s diurnal cortisol rhythms and externalizing behavior. Developmental Psychobiology, 57(8), 935–947. https://doi.org/10.1002/dev.21324
- Bick, J., & Nelson, C. A. (2017). Early experience and brain development. Wiley Interdisciplinary Reviews. Cognitive Science, 8(1–2), 1–2.
- Blanca Mena, M. J., Alarcón, R., Arnau, J., Bono, R., Bendayan, R. (2017). Non-normal data: Is ANOVA still a valid option? Psicothema, 29(4), 552–557.
- Bloch, M., Peleg, I., Koren, D., Aner, H., & Klein, E. (2007). Long-term effects of early parental loss due to divorce on the HPA axis. Hormones and Behavior, 51(4), 516–523. https://doi.org/10.1016/j.yhbeh.2007.01.009
- Bowlby, J. (1969). Attachment and loss. Random House.
- Bruce, J., Fisher, P. A., Pears, K. C., & Levine, S. (2009). Morning cortisol levels in preschool-aged foster children: Differential effects of maltreatment type. Developmental Psychobiology, 51(1), 14–23. https://doi.org/10.1002/dev.20333
- Bunea, I. M., Szentágotai-Tătar, A., & Miu, A. C. (2017). Early-life adversity and cortisol response to social stress: a meta-analysis. Translational Psychiatry, 7(12), 1274. https://doi.org/10.1038/s41398-017-0032-3
- Capitanio, J. P., Mendoza, S. P., Mason, W. A., & Maninger, N. (2005). Rearing environment and hypothalamic-pituitary-adrenal regulation in young rhesus monkeys (Macaca mulatta). Developmental Psychobiology, 46(4), 318–330. https://doi.org/10.1002/dev.20067
- Carpenter, L. L., Tyrka, A. R., McDougle, C. J., Malison, R. T., Owens, M. J., Nemeroff, C. B., & Price, L. H. (2004). Cerebrospinal fluid corticotropin-releasing factor and perceived early-life stress in depressed patients and healthy control subjects. Neuropsychopharmacology: official Publication of the American College of Neuropsychopharmacology, 29(4), 777–784. https://doi.org/10.1038/sj.npp.1300375
- Carpenter, L. L., Tyrka, A. R., Ross, N. S., Khoury, L., Anderson, G. M., & Price, L. H. (2009). Effect of childhood emotional abuse and age on cortisol responsivity in adulthood. Biological Psychiatry, 66(1), 69–75. https://doi.org/10.1016/j.biopsych.2009.02.030
- Carrion, V. G., Weems, C. F., Ray, R. D., Glaser, B., Hessl, D., & Reiss, A. L. (2002). Diurnal salivary cortisol in pediatric posttraumatic stress disorder. Biological Psychiatry, 51(7), 575–582. https://doi.org/10.1016/s0006-3223(01)01310-5
- Champoux, M., Hibbeln, J. R., Shannon, C., Majchrzak, S., Suomi, S. J., Salem, N., & Higley, J. D. (2002). Fatty acid formula supplementation and neuromotor development in rhesus monkey neonates. Pediatric Research, 51(3), 273–281. https://doi.org/10.1203/00006450-200203000-00003
- Claessens, S. E. F., Daskalakis, N. P., van der Veen, R., Oitzl, M. S., de Kloet, E. R., & Champagne, D. L. (2011). Development of individual differences in stress responsiveness: an overview of factors mediating the outcome of early life experiences. Psychopharmacology, 214(1), 141–154. https://doi.org/10.1007/s00213-010-2118-y
- Coplan, J. D., Abdallah, C. G., Kaufman, J., Gelernter, J., Smith, E. L. P., Perera, T. D., Dwork, A. J., Kaffman, A., Gorman, J. M., Rosenblum, L. A., Owens, M. J., & Nemeroff, C. B. (2011). Early-life stress, corticotropin-releasing factor, and serotonin transporter gene: a pilot study. Psychoneuroendocrinology, 36(2), 289–293. https://doi.org/10.1016/j.psyneuen.2010.07.011
- Coplan, J. D., Fathy, H. M., Jackowski, A. P., Tang, C. Y., Perera, T. D., Mathew, S. J., Martinez, J., Abdallah, C. G., Dwork, A. J., Pantol, G., Carpenter, D., Gorman, J. M., Nemeroff, C. B., Owens, M. J., Kaffman, A., & Kaufman, J. (2014). Early life stress and macaque amygdala hypertrophy: preliminary evidence for a role for the serotonin transporter gene. Frontiers in Behavioral Neuroscience, 8, 342. https://doi.org/10.3389/fnbeh.2014.00342
- Corcoran, C. A., Pierre, P. J., Haddad, T., Bice, C., Suomi, S. J., Grant, K. A., Friedman, D. P., & Bennett, A. J. (2012). Long-term effects of differential early rearing in rhesus macaques: Behavioral reactivity in adulthood. Developmental Psychobiology, 54(5), 546–555. https://doi.org/10.1002/dev.20613
- Daskalakis, N. P., Bagot, R. C., Parker, K. J., Vinkers, C. H., & de Kloet, E. R. (2013). The three-hit concept of vulnerability and resilience: toward understanding adaptation to early-life adversity outcome. Psychoneuroendocrinology, 38(9), 1858–1873. https://doi.org/10.1016/j.psyneuen.2013.06.008
- Engel, M. L., & Gunnar, M. R. (2020). The development of stress reactivity and regulation during human development. International Review of Neurobiology, 150, 41–76. https://doi.org/10.1016/bs.irn.2019.11.003
- Erickson, K., Gabry, K. E., Lindell, S., Champoux, M., Schulkin, J., Gold, P., Suomi, S. J., & Higley, J. D. (2005). Social withdrawal behaviors in nonhuman primates and changes in neuroendocrine and monoamine concentrations during a separation paradigm. Developmental Psychobiology, 46(4), 331–339. https://doi.org/10.1002/dev.20061
- Ersig, A. L., Schutte, D. L., Standley, J., Leslie, E. J., Zimmerman, B., Hanrahan, K., Murray, J. C., & McCarthy, A. M. (2019). Genetic variants and the cortisol response in children: An exploratory study. Biological Research for Nursing, 21(2), 157–165. https://doi.org/10.1177/1099800419826315
- Fahlke, C., Lorenz, J. G., Long, J., Champoux, M., Suomi, S. J., & Higley, J. D. (2000). Rearing experiences and stress-induced plasma cortisol as early risk factors for excessive alcohol consumption in nonhuman primates. Alcoholism, Clinical and Experimental Research, 24(5), 644–650. https://doi.org/10.1111/j.1530-0277.2000.tb02035.x
- Ferguson, B., Hunter, J. E., Luty, J., Street, S. L., Woodall, A., & Grant, K. A. (2012). Genetic load is associated with hypothalamic-pituitary-adrenal axis dysregulation in macaques. Genes, Brain, and Behavior, 11(8), 949–957. https://doi.org/10.1111/j.1601-183X.2012.00856.x
- Greenough, W. T., Black, J. E., & Wallace, C. S. (1987). Experience and brain development. Child Development, 58(3), 539–559. https://doi.org/10.2307/1130197
- Gunnar, M. R., Frenn, K., Wewerka, S. S., & Van Ryzin, M. J. (2009). Moderate versus severe early life stress: associations with stress reactivity and regulation in 10-12-year-old children. Psychoneuroendocrinology, 34(1), 62–75. https://doi.org/10.1016/j.psyneuen.2008.08.013
- Gunnar, M. R., Morison, S. J., Chisholm, K., & Schuder, M. (2001). Salivary cortisol levels in children adopted from Romanian orphanages. Development and Psychopathology, 13(3), 611–628. https://doi.org/10.1017/s095457940100311x
- Hengesch, X., Elwenspoek, M. M. C., Schaan, V. K., Larra, M. F., Finke, J. B., Zhang, X., Bachmann, P., Turner, J. D., Vögele, C., Muller, C. P., & Schächinger, H. (2018). Blunted endocrine response to a combined physical-cognitive stressor in adults with early life adversity. Child Abuse & Neglect, 85, 137–144. https://doi.org/10.1016/j.chiabu.2018.04.002
- Higley, J. D., Hopkins, W. D., Thompson, W. W., Byrne, E. A., Hirsch, R. M., & Suomi, S. J. (1992). Peers as primary attachment sources in yearling rhesus monkeys (Macaca mulatta). Developmental Psychology, 28(6), 1163–1171. https://doi.org/10.1037/0012-1649.28.6.1163
- Higley, J. D., Suomi, S. J., & Linnoila, M. (1992). A longitudinal assessment of CSF monoamine metabolite and plasma cortisol concentrations in young rhesus monkeys. Biological Psychiatry, 32(2), 127–145. https://doi.org/10.1016/0006-3223(92)90016-s
- Karlamangla, A. S., Merkin, S. S., Almeida, D. M., Friedman, E. M., Mogle, J. A., & Seeman, T. E. (2019). Early-life adversity and dysregulation of adult diurnal cortisol rhythm. The Journals of Gerontology. Series B, Psychological Sciences and Social Sciences, 74(1), 160–169. https://doi.org/10.1093/geronb/gby097
- Keck, M. E., Kern, N., Erhardt, A., Unschuld, P. G., Ising, M., Salyakina, D., Müller, M. B., Knorr, C. C., Lieb, R., Hohoff, C., Krakowitzky, P., Maier, W., Bandelow, B., Fritze, J., Deckert, J., Holsboer, F., Müller-Myhsok, B., & Binder, E. B. (2008). Combined effects of exonic polymorphisms in CRHR1 and AVPR1B genes in a case/control study for panic disorder. American Journal of Medical Genetics. Part B, Neuropsychiatric Genetics: The Official Publication of the International Society of Psychiatric Genetics, 147B(7), 1196–1204. https://doi.org/10.1002/ajmg.b.30750
- Kinnally, E. L., & Capitanio, J. P. (2015). Paternal early experiences influence infant development through non-social mechanisms in Rhesus Macaques. Frontiers in Zoology, 12 Suppl 1(Suppl 1), S14. https://doi.org/10.1186/1742-9994-12-S1-S14
- Koss, K. J., & Gunnar, M. R. (2018). Annual Research Review: Early adversity, the hypothalamic-pituitary-adrenocortical axis, and child psychopathology. Journal of Child Psychology and Psychiatry, and Allied Disciplines, 59(4), 327–346. https://doi.org/10.1111/jcpp.12784
- Koss, K. J., Hostinar, C. E., Donzella, B., & Gunnar, M. R. (2014). Social deprivation and the HPA axis in early development. Psychoneuroendocrinology, 50, 1–13. https://doi.org/10.1016/j.psyneuen.2014.07.028
- Kuras, Y. I., Assaf, N., Thoma, M. V., Gianferante, D., Hanlin, L., Chen, X., Fiksdal, A., & Rohleder, N. (2017). Blunted diurnal cortisol activity in healthy adults with childhood adversity. Frontiers in Human Neuroscience, 11, 574. https://doi.org/10.3389/fnhum.2017.00574
- Laryea, G., Arnett, M. G., & Muglia, L. J. (2012). Behavioral studies and genetic alterations in Corticotropin-Releasing Hormone (CRH) neurocircuitry: Insights into human psychiatric disorders. Behavioral Sciences (Basel, Switzerland), 2(2), 135–171. https://doi.org/10.3390/bs2020135
- Lee, R., Geracioti, T. D., Kasckow, J. W., & Coccaro, E. F. (2005). Childhood trauma and personality disorder: positive correlation with adult CSF corticotropin-releasing factor concentrations. The American Journal of Psychiatry, 162(5), 995–997. https://doi.org/10.1176/appi.ajp.162.5.995
- Levine, S. (1962). Plasma-free corticosteroid response to electric shock in rats stimulated in infancy. Science (New York, NY), 135(3506), 795–796. https://doi.org/10.1126/science.135.3506.795-a
- McLaughlin, K. A., Sheridan, M. A., Tibu, F., Fox, N. A., Zeanah, C. H., & Nelson, C. A. (2015). Causal effects of the early caregiving environment on development of stress response systems in children. Proceedings of the National Academy of Sciences of the United States of America, 112(18), 5637–5642. https://doi.org/10.1073/pnas.1423363112
- Morris, M. C., & Rao, U. (2013). Psychobiology of PTSD in the acute aftermath of trauma: Integrating research on coping, HPA function and sympathetic nervous system activity. Asian Journal of Psychiatry, 6(1), 3–21. https://doi.org/10.1016/j.ajp.2012.07.012
- Nelson, C. A., Scott, R. D., Bhutta, Z. A., Harris, N. B., Danese, A., & Samara, M. (2020). Adversity in childhood is linked to mental and physical health throughout life. BMJ (Clinical Research ed.), 371, m3048. https://doi.org/10.1136/bmj.m3048
- Newman, T. K., Syagailo, Y. V., Barr, C. S., Wendland, J. R., Champoux, M., Graessle, M., Suomi, S. J., Higley, J. D., & Lesch, K.-P. (2005). Monoamine oxidase A gene promoter variation and rearing experience influences aggressive behavior in rhesus monkeys. Biological Psychiatry, 57(2), 167–172. https://doi.org/10.1016/j.biopsych.2004.10.012
- Ouellet-Morin, I., Odgers, C. L., Danese, A., Bowes, L., Shakoor, S., Papadopoulos, A. S., Caspi, A., Moffitt, T. E., & Arseneault, L. (2011). Blunted cortisol responses to stress signal social and behavioral problems among maltreated/bullied 12-year-old children. Biological Psychiatry, 70(11), 1016–1023. https://doi.org/10.1016/j.biopsych.2011.06.017
- Ressler, K. J., Bradley, B., Mercer, K. B., Deveau, T. C., Smith, A. K., Gillespie, C. F., Nemeroff, C. B., Cubells, J. F., & Binder, E. B. (2010). Polymorphisms in CRHR1 and the serotonin transporter loci: gene x gene x environment interactions on depressive symptoms. American Journal of Medical Genetics. Part B, Neuropsychiatric Genetics: The Official Publication of the International Society of Psychiatric Genetics, 153B(3), 812–824. https://doi.org/10.1002/ajmg.b.31052
- Robin, R. W., Chester, B., Rasmussen, J. K., Jaranson, J. M., & Goldman, D. (1997). Prevalence and characteristics of trauma and posttraumatic stress disorder in a southwestern American Indian community. The American Journal of Psychiatry, 154(11), 1582–1588. https://doi.org/10.1176/ajp.154.11.1582
- Sanchez, M. M. (2006). The impact of early adverse care on HPA axis development: nonhuman primate models. Hormones and Behavior, 50(4), 623–631. https://doi.org/10.1016/j.yhbeh.2006.06.012
- Sánchez, M. M., Noble, P. M., Lyon, C. K., Plotsky, P. M., Davis, M., Nemeroff, C. B., & Winslow, J. T. (2005). Alterations in diurnal cortisol rhythm and acoustic startle response in nonhuman primates with adverse rearing. Biological Psychiatry, 57(4), 373–381. https://doi.org/10.1016/j.biopsych.2004.11.032
- Santarelli, S., Zimmermann, C., Kalideris, G., Lesuis, S. L., Arloth, J., Uribe, A., Dournes, C., Balsevich, G., Hartmann, J., Masana, M., Binder, E. B., Spengler, D., & Schmidt, M. V. (2017). An adverse early life environment can enhance stress resilience in adulthood. Psychoneuroendocrinology, 78, 213–221. https://doi.org/10.1016/j.psyneuen.2017.01.021
- Schäfer, I., Teske, L., Schulze-Thüsing, J., Homann, K., Reimer, J., Haasen, C., Hissbach, J., & Wiedemann, K. (2010). Impact of childhood trauma on hypothalamus-pituitary-adrenal axis activity in alcohol-dependent patients. European Addiction Research, 16(2), 108–114. https://doi.org/10.1159/000294362
- Schneider, M. L., & Suomi, S. J. (1992). Neurobehavioral assessment in rhesus monkey neonates (Macaca mulatta): Developmental changes, behavioral stability, and early experience. Infant Behavior and Development, 15(2), 155–177. https://doi.org/10.1016/0163-6383(92)80021-L
- Shannon, C., Champoux, M., & Suomi, S. J. (1998). Rearing condition and plasma cortisol in rhesus monkey infants. American Journal of Primatology, 46(4), 311–321. https://doi.org/10.1002/(SICI)1098-2345(1998)46:4<311::AID-AJP3>3.0.CO;2-L
- Sheikh, H. I., Kryski, K. R., Smith, H. J., Hayden, E. P., & Singh, S. M. (2013). Corticotropin-releasing hormone system polymorphisms are associated with children’s cortisol reactivity. Neuroscience, 229, 1–11. https://doi.org/10.1016/j.neuroscience.2012.10.056
- Smoller, J. W., Rosenbaum, J. F., Biederman, J., Kennedy, J., Dai, D., Racette, S. R., Laird, N. M., Kagan, J., Snidman, N., Hirshfeld-Becker, D., Tsuang, M. T., Sklar, P. B., & Slaugenhaupt, S. A. (2003). Association of a genetic marker at the corticotropin-releasing hormone locus with behavioral inhibition. Biological Psychiatry, 54(12), 1376–1381. https://doi.org/10.1016/s0006-3223(03)00598-5
- Smoller, J. W., Yamaki, L. H., Fagerness, J. A., Biederman, J., Racette, S., Laird, N. M., Kagan, J., Snidman, N., Faraone, S. V., Hirshfeld-Becker, D., Tsuang, M. T., Slaugenhaupt, S. A., Rosenbaum, J. F., & Sklar, P. B. (2005). The corticotropin-releasing hormone gene and behavioral inhibition in children at risk for panic disorder. Biological Psychiatry, 57(12), 1485–1492. https://doi.org/10.1016/j.biopsych.2005.02.018
- Sproul Bassett, A. M., Wood, E. K., Lindell, S. G., Schwandt, M. L., Barr, C. S., Suomi, S. J., & Higley, J. D. (2020). Intergenerational effects of mother’s early rearing experience on offspring treatment and socioemotional development. Developmental Psychobiology, 62(7), 920–931. https://doi.org/10.1002/dev.21959
- Tyrka, A. R., Wier, L., Price, L. H., Ross, N., Anderson, G. M., Wilkinson, C. W., & Carpenter, L. L. (2008). Childhood parental loss and adult hypothalamic-pituitary-adrenal function. Biological Psychiatry, 63(12), 1147–1154. https://doi.org/10.1016/j.biopsych.2008.01.011
- Wolf, E. J., Mitchell, K. S., Logue, M. W., Baldwin, C. T., Reardon, A. F., Humphries, D. E., & Miller, M. W. (2013). Corticotropin releasing hormone receptor 2 (CRHR-2) gene is associated with decreased risk and severity of posttraumatic stress disorder in women. Depression and Anxiety, 30(12), 1161–1169. https://doi.org/10.1002/da.22176
- Wood, E. K., Champoux, M., Lindell, S. G., Barr, C. S., Suomi, S. J., & Higley, J. D. (2020). Neonatal temperament and neuromotor differences are predictive of adolescent alcohol intake in rhesus monkeys (Macaca mulatta). American Journal of Primatology, 82(11), e23043. https://doi.org/10.1002/ajp.23043
- Young, J. J., Silber, T., Bruno, D., Galatzer-Levy, I. R., Pomara, N., & Marmar, C. R. (2016). Is there progress? An overview of selecting biomarker candidates for major depressive disorder. Frontiers in Psychiatry, 7, 72. https://doi.org/10.3389/fpsyt.2016.00072
- Zhang, B. (2017). Consequences of early adverse rearing experience (EARE) on development: insights from non-human primate studies. Zoological Research, 38(1), 7–35. https://doi.org/10.13918/j.issn.2095-8137.2017.002