Abstract
The present study evaluated the effect of chronic cold stress on intestinal epithelial cell proliferation and inflammation. Male Wistar rats were subjected to cold exposure for three weeks. At the end of the cold exposure, intestinal cell proliferation, luminal nitrite and protein levels, intestinal myeloperoxidase activity and mast cell numbers were evaluated. Severely compromised proliferation rate of the crypt-base cells was observed under chronic stress conditions. Cells isolated from stressed rats showed a decreased DNA content in villus and lower villus cell fractions and an increased DNA content in the crypt cells, as compared to controls. Chronic cold stress resulted in increased luminal nitrite, luminal protein levels, and intestinal myeloperoxidase activity. The number of mast cells was significantly elevated under chronic stress conditions. Chronic cold stress resulted in a compromised intestinal epithelial cell proliferation rate and induced inflammation in the rat small intestine, through the combined action of nitric oxide, neutrophils and mast cells.
Introduction
Stressful life events have long been implicated in the pathogenesis of various gastrointestinal diseases, such as inflammatory bowel disease and ulcerative colitis (Collins Citation2001). Recent observations suggest stress to be a potent modulator of the intestinal inflammatory response (Collins Citation2001) but there are limited experimental data to support the hypothesis that stress per se can induce inflammation. To study the possibility of disease-promoting effects of life stressors, several models of nontraumatic “physiological” stress have been developed (Söderholm and Perdue Citation2001). There are studies describing the effect of acute stressors on gastrointestinal pathologies, but consequences of long-term exposure to stressors on stress-induced inflammation have not received much attention. In the present study, long term cold stress was chosen as the model because it is a continuous stressor and has both psychological and physiological components (Kim et al. Citation1999), and it also includes acclimatization. In this model the Hypothalamus-pituitary axis is activated which is a key marker of a stress response.
Earlier, we had shown that chronic cold stress results in the generation of oxidative stress in various rat tissues (Kaushik and Kaur Citation2003). Particularly, in the small intestine, chronic stress alters the prooxidant-antioxidant balance of the free radical process—with an increase in xanthine oxidase activity and lipid peroxidation and a decrease in glutathione level, superoxide dismutase and glutathione reductase activities. Since membrane lipids and proteins are the main target of the oxygen-derived free radicals (Butterfield et al. Citation1998), we studied intestinal brush border membrane composition and enzyme activities, which have been observed to be altered in chronic cold stress-exposed rats (Kaushik et al. Citation2003). Moreover, an increase in reactive oxygen intermediates has also been increasingly implicated in the process of inflammation (Kruidenier et al. Citation2003). Hence, it was of interest to evaluate the role of stress in inducing intestinal inflammation. Due to its enormous mucosal surface, which is continuosly exposed to a myriad of antigenic, mitogenic and toxic stimuli, the small intestine is highly susceptible to such inflammatory responses. The homeostasis and integrity of the small intestinal epithelium are dependent largely on the continuous proliferation, migration, and differentiation of crypt cells (Stappenbeck et al. Citation1998). Intestinal mucosal cells have one of the highest turnover rates in the body and hence are highly sensitive to various environmental stress conditions (Hart and Kamm Citation2002). The observed changes in brush border membrane structure and function (Kaushik et al. Citation2003) can be a consequence of altered cell proliferation in the crypt.
Therefore, the present study was designed to evaluate the effect of chronic cold stress on intestinal cell proliferation and inflammation. Crypt cell proliferation was studied by [3H]-thymidine incorporation into cellular DNA. The inflammatory markers studied were luminal nitrite and protein content, tissue myeloperoxidase activity and mast cell number in the rat intestine.
Materials and methods
Materials
[3H]-Thymidine was purchased from BRIT, Bombay (India). Hexadecyltrimethylammonium bromide (HTAB) was purchased from Merck. Bovine serum albumin, o-dianisidine dihydrochloride, sulfonyl salicylic acid, sulfanilamide, naphthyl ethylenediamine dihydrochloride, diphenylamine, toluidine blue and standard DNA were purchased from Sigma Chemical Co., USA.
Animals and experimental design
Male albino rats (Wistar strain) 2–3 months old, weighing 100–120 g at the beginning of the experiments, were used. The rats were randomized into two groups. Each group had 6 rats, and each animal was housed in an individual cage. The control group rats were kept at room temperature (∼25°C) and the cold-stressed group rats were kept at 6–8°C in a cold room continuously for 3 weeks. Rats were maintained on a 12 h light: 12 h dark cycle with lights on at 07.00 h. The rats were housed in polypropylene cages containing rice husk, and were provided water and a standard rat pellet diet (Ashirwad Industries, Ropar, India) ad libitum. The protocol was approved by the institute's “ethical committee on animal experiments”.
Tissue preparation
After the cold exposure for 3 weeks, the overnight fasted rats were killed under sodium pentobarbital anaesthesia (40 mg/kg, i.p.), always between 08.00 and 09.00 h. The abdominal cavity was opened, and starting from the ligament of Trietz, the entire small intestine was excised and luminal washings were collected by flushing 10 ml of chilled saline (0.9% (w/v) NaCl) through the intestine, for the estimation of nitrite and proteins. The small intestines were thoroughly rinsed with chilled saline, blotted dry and a proximal 1 cm section was removed and stored in 10% formalin for histological examination, and the remaining tissue was processed for myeloperoxidase activity measurement.
Measurement of intestinal cell proliferation
Intestinal cell proliferation was evaluated by examining thymidine kinase activity as follows (Sundaram et al. Citation1991). Ninety minutes before sacrifice, [3H]-thymidine (50 μCi/100 g body weight) was given intraperitoneally to three rats from each group. Epithelial cells from the intestines were isolated, following the method of Weiser (Citation1973), which involved a series of incubations and washings of intestinal loops at 37°C for specified time intervals. The sequential fractions (1–8), which define a gradient of cells from the villus tip to lower villus and crypt base areas, were isolated. The radioactivity incorporated into the cells from the different fractions was measured. Also, the DNA content of each of the fractions was measured spectrophotometrically by the method of Burton (Citation1956). The thymidine kinase specific activity was expressed as cpm/mg DNA.
Luminal nitrite and protein assay
Luminal washings were centrifuged at 900g for 15 min and the supernatant obtained was used for the estimation of total nitrite and protein contents. For the nitrite assay, the supernatant was mixed in a 96-well microtiter plate, with Griess reagent (1% sulfanilamide/0.1% naphthyl ethylenediamine dihydrochloride/25% H3PO4) to form a chromophore absorbing at 595 nm in accordance with the method of Green et al. (Citation1981). The total luminal nitrite levels were calculated from a standard curve generated with sodium nitrite. The total luminal protein content was determined by the method of Lowry et al. (Citation1951).
Myeloperoxidase (MPO) activity
Intestinal inflammation was assessed by measuring the activity of tissue MPO, an enzyme found in granulated cells and previously shown to be a reliable index of inflammation (Miller et al. Citation1993). The activity of MPO was assayed according to a previously described method with slight modifications (Krawisz et al. Citation1984). Briefly, intestinal tissue (200–400 mg) was minced in a beaker containing 5 ml of HTAB buffer (0.5% HTAB in 50 mM phosphate buffer, pH 6.0). HTAB is a detergent that releases MPO from the primary granules of the neutrophils. The preparation was homogenized and centrifuged at 20,000g for 15 min at 4°C to pellet the insoluble cellular debris. The supernatant, which contained < 5% of the total MPO activity, was discarded. The pellet was then rehomogenized in an equivalent volume of HTAB buffer. The samples were centrifuged at 20,000g for 15 min at 4°C and the supernatants saved. MPO activity was assessed by combining 100 μl of supernatant with 2.9 ml of 50 mM phosphate buffer, pH 6.0, containing 0.167 mg/ml o-dianisidine hydrochloride and 0.0005% hydrogen peroxide. The change in absorbance at 460 nm was measured spectrophotometrically. One unit of MPO activity is defined as that degrading 1 μmol of peroxide per minute at 25°C. Because MPO activity decreases despite storage at − 80°C, all samples were assayed on the day of sacrificing the rats.
Histological examination for mast cells
Full thickness segments of duodenal tissues were fixed in formalin for a minimum of 24 h, embedded in wax and transverse sections were cut and stained with toluidine blue. The sections were analyzed blindly for mast cell numbers at 1000X magnification.
Statistical analysis
All values were computed as means ± S.D. The data on thymidine kinase activity and DNA content in various cell fractions across the crypt villus axis were analysed by using a 2-way parametric analysis of variance and Kruskal-Wallis non-parametric analysis, respectively, followed by post-hoc tests. Student's t-test was employed for statistical comparisons between the control and cold-stressed groups for other parameters. Significance was considered at the level of P < 0.05.
Results
The mean food intake of each rat was 15 ± 2 g/day in the control group and 20 ± 2 g/day in the cold-stressed group (P < 0.05). No significant difference in the body weight of control and stressed rats was observed. At the time of sacrifice, the body weights of the control and stressed rats were 159 ± 10 and 149 ± 8 g, respectively.
Intestinal cell proliferation
depicts the specific activity of thymidine kinase in isolated crypt-villus cells from control and cold-stressed rat intestines. Since thymidine kinase is a marker of crypt cells, it assesses the effectiveness of our fractionation procedure. As evident from , thymidine kinase was minimum in fraction 1 of isolated cells and progressively increased to fraction 8 in both groups of rats. There was a five fold increase in the enzyme activity in fraction 8 over fraction 1, in both the control and cold stressed groups, suggesting that similar cell populations were present with a gradient of activity from the villus to the crypt cells in the two groups. However, this enzyme activity showed a significant decrease across the crypt-villus axis in rats exposed to chronic cold stress, indicating a depressed proliferative potential of the crypt-base cells, in this condition.
Figure 1 Effect of chronic cold stress on thymidine kinase activity in isolated crypt-villus cells from rat intestine. Fractions 1–3, 4–6, and 7–8 represent the villus tip, mid villus and crypt base cells, respectively. Values are mean ± S.D. *P < 0.05, **P < 0.01 as compared to control. F = 253, P < 0.001 ▪ Control; ▪ Cold-stressed.
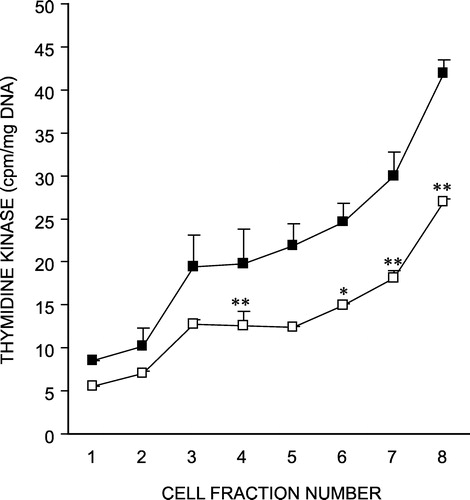
DNA content of the isolated cell fractions was also measured (). Cells isolated from rats exposed to cold stress showed a decreased DNA content in the villus and lower villus cell fractions as compared to the controls. However, the crypt cells from the cold stress group revealed an increased DNA content compared to those from control rats. As DNA content depicts the cell number, the observations suggest a reduced number of mature villus cells and an increased number of proliferating crypt cells in the cold-stressed rats.
Luminal nitrite and protein
Chronic cold stress resulted in a significant increase in total luminal nitrite (P < 0.001) and protein (P < 0.01) () contents, which are established markers of inflammation (Miller et al. Citation1993).
Myeloperoxidase activity
Use of MPO to quantitate intestinal inflammation has been shown to correlate well with the appearance of neutrophils in the intestinal mucosa (Krawisz et al. Citation1984). compares MPO activity in control and cold-stressed rats. Our data show that MPO activity was significantly increased (P < 0.01) after chronic cold stress, indicating that there is substantial neutrophil influx into the mucosa, under this condition.
Mast cells
Studies on intestinal mucosal ultrastructure have revealed mucosal mast cell activation in various intestinal pathologies (Yu and Perdue Citation2001). Hence, we studied the number of mast cells in the intestinal mucosa of control and stressed rats. As shown in , the average number of mast cells stained per section was significantly greater in stressed rat small intestine than in controls. is a representative photomicrograph of mast cells in duodenal sections from control and cold-stressed rats.
Discussion
All intestinal epithelial cells are derived from a small population of cells situated in the crypt base. Cells proliferate in the crypts, differentiate as they migrate toward the villi and reach the villus tips where they are shed into the intestinal lumen. Thus, changes in villus morphology and function can be a consequence of altered cell production in the crypts, which is a highly sensitive process susceptible to various environmental stress conditions (Stappenbeck et al. Citation1998). There is increasing recognition of the role of stress in influencing the symptoms and course of chronic intestinal diseases (Hart and Kamm Citation2002), but relatively little knowledge regarding stress as the primary cause of intestinal inflammation, so we sought to study the effect of chronic cold stress on intestinal crypt cell proliferation and inflammation.
The results demonstrated reduced thymidine kinase activity in all of the isolated cell fractions across the crypt-villus axis in cold stressed rats as compared to the controls, suggesting reduced cellular proliferation. This was corroborated by decreased DNA content in the villus tip and lower villus cells in stressed rats, implying reduced cell number. But a significantly increased DNA content was observed in fraction 8 (crypt cells) from rats exposed to chronic cold stress, despite reduced thymidine kinase activity. A previous report showed decreased epithelial cell proliferation in the small intestine after prolonged exposure of rats to cold-restraint stress (Greant et al. Citation1988). Recently, in a thermal injury stress model, Varedi et al. (Citation2001) have also observed a cell position dependent decrease in proliferation across the crypt-villus axis in rats. The increased amount of DNA in crypt cells may be due to a slow rate of migration of crypt cells along the crypt-villus axis, resulting in accumulation of cells in the crypt. Thus, rats exposed to cold stress for 3 weeks evidently exhibited reduced crypt cell proliferation and probably a slower rate of migration of crypt cells towards the villus.
Further, to investigate the effect of chronic cold stress on induction of intestinal inflammation, nitrite and protein contents were measured in the luminal washings. Luminal contents of nitrite and protein were markedly elevated after exposing the rats to cold stress. Content of nitrite, a stable end product of nitric oxide formation, was determined to quantify nitric oxide production because a very short half-life of nitric oxide precludes its direct measurement, especially in animal studies (Ignarro et al. Citation1993). Earlier, Roediger et al. (Citation1986) have observed increased luminal nitrite levels that accompanied chronic intestinal inflammation. Later, Miller et al. (Citation1993) by using three different experimental models of both acute as well as chronic gut insults confirmed that nitric oxide release is a common response to inflammatory conditions. Further, increased luminal nitrite levels have been reported in inflammatory bowel disease (IBD), which were attributed to inducible nitric oxide synthase (iNOS) activity (Alican and Kubes Citation1996). Elevated nitric oxide levels accompanied by iNOS activation have also been found in a rhesus monkey colitis model (Ribbons et al. Citation1995). Further, elevated NOS activity in the colonic mucosa of ulcerative colitis patients was observed (Boughton-Smith et al. Citation1993). The cellular source of luminal nitrite, under physiological conditions, most likely is the endothelium as the constitutive Ca2+-dependent form of NOS is the only form expressed under basal conditions (Salter et al. Citation1991). However, macrophages, neutrophils, mast cells and granulocytes may be activated by stress and may contribute to nitric oxide release (Morris and Billiar Citation1994). Hence, it is clear that luminal nitrite levels can be used as an index of intestinal injury but the role of nitric oxide produced under these conditions remains contradictory. At physiological concentrations, nitric oxide protects the gastrointestinal mucosa from injury by acting as an antioxidant, by inhibiting leukocyte adherence and by maintaining mucosal blood flow (Alican and Kubes Citation1996). During inflammation, iNOS is induced in the activated cells including intestinal epithelial cells. Unlike the isoform of NOS found in endothelial cells, iNOS is not regulated by intracellular calcium ion concentration and is, therefore, capable of generating high local intracellular and extracellular concentrations of nitric oxide (Miller et al. Citation1995). Abundant nitric oxide and oxygen derived radicals react rapidly to form peroxynitrite (ONOO− ), an extremely toxic and reactive species (Pryor and Squadrito Citation1995, Beckman and Koppenol Citation1996), which can result in tissue injury in the inflamed intestinal mucosa. In a recent study, peroxynitrite has been shown to inhibit epidermal growth factor receptor signaling, resulting in reduced cell proliferation in Caco-2 cells (Aliye et al. Citation2003). In the present study, the same mechanism may partly contribute to the observed decrease in cell proliferation in addition to the reduced thymidine kinase activity in stressed rats.
The increased luminal protein content observed in stressed rats might be due to enhanced cell shedding from the villus into the lumen, which is evident from the reduced cell number depicted by reduced DNA content under these conditions. Previously, gut lavage fluid proteins have been shown to be a marker of active IBD (O'Mahony et al. Citation1991).
Further, we determined another important marker of inflammation, MPO activity. MPO is an enzyme found mainly in neutrophils and in much smaller amounts in monocytes and macrophages (Bos et al. Citation1978, Matheson et al. Citation1981). Extravasated neutrophils were found to be a major cause of tissue injury associated with IBD (Morson Citation1980), and activity of MPO from inflamed tissue was observed to be directly proportional to the number of neutrophils seen (Krawisz et al. Citation1984). We found a significantly increased activity of MPO in intestinal mucosa from stressed rats as compared to controls, signifying an increased neutrophil infiltration and hence, inflammation in intestinal tissue from stressed rats. Stimulated neutrophils are known to produce superoxide dismutase anions, other reactive oxygen products and granules containing lysosomal enzymes, which can result in cell injury (Krawisz et al. Citation1984). Also, neutrophils are an important source of soluble mediators of inflammation like leukotriene B4 and 5-hydroxyeicosatetraenoic acid (Sharon and Stenson Citation1984). Our results are consistent with recent observations of Söderholm et al. (Citation2002) who showed infiltration of neutrophils with increased MPO activity in ileum and colon of rats subjected to chronic psychological stress.
Our results on mast cell number in the intestinal mucosa revealed a significant increase after stress exposure. Activation of mucosal nerves by stress releases corticotropin releasing hormone and acetylcholine, which activate mast cells (Castagliuolo et al. Citation1996). It has been established that mast cell activity is associated with ion and macromolecular epithelial transport changes in human and rat intestine (Berin et al. Citation1998, Crowe and Perduem Citation1992). Also, studies from various groups have suggested the importance of mast cells in stress-related changes in intestinal barrier function. Santos et al. (Citation1998) have found that acute cold stress caused luminal release of mast cell mediators, tryptase and histamine. In another recent study, chronic water avoidance stress has been shown to stimulate secretion and permeability in rat jejunal epithelium, which were mast cell dependent because mast cell deficient rats did not show any such changes (Santos et al. Citation2000). Using the same model of stress, infiltration of mononuclear cells, neutrophils and mast cells was observed in the ileum and colon of rats (Söderholm et al. Citation2002). In addition, an increased number of mast cells has been reported in the small intestine of patients with irritable bowel syndrome (Weston et al. Citation1993), the clinical evolution of this disease being strongly associated with life stress. Hence, stress can activate mast cells, which can increase gut permeability, as a result of which luminal antigens may gain access to the epithelium causing inflammation.
In conclusion, the present study suggests that chronic cold stress results in altered cell proliferation in the small intestinal mucosa of rats and supports the hypothesis that chronic stress can be an initiating factor in inducing inflammation in the small intestine, a process which might involve nitric oxide, neutrophils and mast cells.
Acknowledgements
This study was supported by the grant of junior research fellowship awarded by Indian Council of Medical Research, New Delhi to Ms Susmita Kaushik. The authors are thankful to Dr Kim Vaiphei, Department of Histopathology, PGIMER, Chandigarh, for helping in histological examination.
Notes
† Present address: Department of Anatomy and Structural Biology, Marion Bessin Liver Research Center, Albert Einstein College of Medicine, Bronx, NY 10461, USA.
References
- Alican I, Kubes P. A critical role for nitric oxide in intestinal barrier function and dysfunction. Am J Physiol 1996; 270: G225–G237
- Aliye UC, Kooy NW, Conklin JL, Bishop WP. Peroxynitrite inhibits epidermal growth factor receptor signaling in Caco-2 cells. Dig Dis Sci 2003; 48: 2353–2359
- Beckman JS, Koppenol WH. Nitric oxide, superoxide, and peroxynitrite: The good, the bad, and ugly. Am J Physiol 1996; 271: C1424–C1437
- Berin MC, Kiliaan AJ, Yang P-C, Groot JA, Kitamura Y, Perdue MH. The influence of mast cells on pathways of transepithelial antigen transport in the rat intestine. J Immunol 1998; 161: 2561–2566
- Bos A, Wever R, Roos D. Characterization and quantification of the peroxidase in human monocytes. Biochim Biophys Acta 1978; 525: 37–44
- Boughton-Smith NK, Evans SM, Hawkey CJ, Cole AT, Balsitis M, Whittle BJ, Moncada S. Nitric oxide synthase activity in ulcerative colitis and Crohn's disease. Lancet 1993; 342: 338–340
- Burton KA. Study of the conditions and mechanism of the diphenylamine reaction for the colorimetric estimation of deoxyribonucleic acid. Biochem J 1956; 62: 315–323
- Butterfield DA, Koppal T, Howard B, Subramaniam R, Hall N, Hensley K, Yatin S, Allen K, Aksenov M, Aksenova M, Carney J. Structural and functional changes in proteins induced by free radical-mediated oxidative stress and protective action of the antioxidants N-tert-butyl-alpha-phenylnitrone and vitamin E. Ann NY Acad Sci 1998; 20: 448–462
- Castagliuolo I, Lamont JT, Qiu B, Fleming SM, Bhaskar KR, Nikulasson ST, Kornetsky C, Pothoulakis C. Acute stress causes mucin release from rat colon: Role of corticotropin-releasing factor and mast cells. Am J Physiol 1996; 271: G884–G892
- Collins SM. Stress and the Gastrointestinal Tract IV. Modulation of intestinal inflammation by stress: Basic mechanisms and clinical relevance. Am J Physiol 2001; 280: G315–G318
- Crowe SE, Perduem MH. Gastrointestinal food hypersensitivity: Basic mechanisms of pathophysiology. Gastroenterology 1992; 103: 1075–1095
- Greant P, Delvaux G, Willems G. Influence of stress on epithelial cell proliferation in the gut mucosa of rats. Digestion 1988; 40: 212–218
- Green LC, Tannebaum SR, Goldman P. Nitrate synthesis in the germfree and conventional rat. Science 1981; 212: 56–58
- Hart A, Kamm MA. Mechanisms of initiation and perpetuation of gut inflammation by stress. Aliment Pharmacol Ther 2002; 16: 2017–2028
- Ignarro LJ, Fukuto JM, Griscavage JM, Rogers NE, Byrns RE. Oxidation of nitric oxide in aqueous solution to nitrite but not nitrate: Comparison with enzymatically formed nitric oxide from l-arginine. Proc Natl Acad Sci USA 1993; 90: 8103–8107
- Kaushik S, Kaur J. Chronic cold exposure affects the antioxidant defense system in various rat tissues. Clin Chim Acta 2003; 333: 69–77
- Kaushik S, Kaler P, Kaur J. Chronic cold stress-induced alterations in brush border membrane composition and enzyme activities in rat intestine. Indian J Biochem Biophys 2003; 40: 180–185
- Kim CK, Giberson PK, Yu W, Zoeller RT, Weinberg J. Effects of prenatal ethanol exposure on hypothalamic-pituitary-adrenal responses to chronic cold stress in rats. Alcohol Clin Exp Res 1999; 23: 301–310
- Krawisz JE, Sharon P, Stenson WF. Quantitative assay for acute intestinal inflammation based on myeloperoxidase activity. Assessment of inflammation in rat and hamster models. Gastroenterology 1984; 87: 1344–1350
- Kruidenier L, Kuiper I, Van Duijn W, Mieremet-Ooms MA, van Hogezand RA, Lamers CB, Verspaget HW. Intestinal oxidative damage in inflammatory bowel disease: Semi-quantification, localization, and association with mucosal antioxidants. J Pathol 2003; 201: 17–27
- Lowry OH, Rosenbergh NJ, Farr AL, Randall RJ. Protein measurement with the Folin phenol reagent. J Biol Chem 1951; 193: 265–275
- Matheson NR, Wong PS, Travis J. Isolation and properties of human neutrophils Myeloperoxidase. Biochemistry 1981; 20: 325–330
- Miller MJ, Zhang XJ, Sadowska-Krowicka H, Chotinaruemol S, McIntyre JA, Clark DA, Bustamante SA. Nitric oxide release in response to gut injury. Scand J Gastroenterol 1993; 28: 149–154
- Miller MJ, Thompson JH, Zhang XJ, Sadowska-Krowicka H, Kakkis JL, Munshi UK, Sandoval M, Rossi JL, Eloby-Childress S, Beckman JS, et al. Role of inducible nitric oxide synthase expression and peroxynitrite formation in guinea pig ileitis. Gastroenterology 1995; 109: 1475–1483
- Morris SM, Jr, Billiar TR. New insights into the regulation of inducible nitric oxide synthesis. Am J Physiol 1994; 266: E829–E839
- Morson BC. Pathology of ulcerative colitis. Inflammatory bowel disease, JP Kirsner, RG Shorter. Lea & Febiger. 1980; 281–291
- O'Mahony S, Choudari CP, Barton JR, Walker S, Ferguson A. Gut lavage fluid proteins as markers of activity of inflammatory bowel disease. Scand J Gastroenterol 1991; 26: 940–944
- Pryor WA, Squadrito GL. The chemistry of peroxynitrite and peroxynitrous acid: Products from the reaction of nitric oxide with superoxide. Am J Physiol 1995; 268: 699–721
- Ribbons KA, Zhang XJ, Thompson JH, Greenberg SS, Moore WM, Kornmeier CM, Currie MG, Lerche N, Blanchard J, Clark DA, et al. Potential role of nitric oxide in a chronic model of colitis in rhesus macaques. Gastroenterology 1995; 108: 705–711
- Roediger WEW, Lawson MJ, Nance SH, Radcliffe BC. Detectable colonic nitrite levels in inflammatory bowel disease-mucosal or bacterial malfunction. Digestion 1986; 35: 199–204
- Salter M, Knowles RG, Moncada S. Widespread tissue distribution, species distribution and changes in activity of Ca2+-dependent and Ca2+-independent nitric oxide synthases. FEBS 1991; 291: 145–149
- Santos J, Benjamin M, Yang PC, Prior T, Perdue MH. Chronic stress impairs rat growth and jejunal epithelial barrier function: Role of mast cells. Am J Physiol 2000; 278: G847–G854
- Santos J, Saperas E, Nogueiras C, Mourelle M, Antolin M, Cadahia A, Malagelada JR. Release of mast cell mediators into the jejunum by cold pain stress in humans. Gastroenterology 1998; 114: 640–648
- Sharon P, Stenson WF. Enhanced synthesis of leukotriene by colonic mucosa in inflammatory bowel disease. Gastroenterology 1984; 86: 453–460
- Söderholm JD, Perdue MH. Stress and the gastrointestinal tract II. Stress and intestinal barrier function. Am J Physiol 2001; 280: G7–13
- Söderholm JD, Yang PC, Ceponis P, Vohra A, Riddell R, Sherman PM, Perdue MH. Chronic stress induces mast cell-dependent bacterial adherence and initiates mucosal inflammation in rat intestine. Gastroenterology 2002; 123: 1099–1108
- Stappenbeck TS, Wong MH, Saam JR, Mysorekar IU, Gordon JI. Notes from some crypt watchers: Regulation of renewal in the mouse intestinal epithelium. Curr Opin Cell Biol 1998; 10: 702–709
- Sundaram U, Knickelbein RG, Dobbins JW. pH regulation in ileum: Na+ -H+ and Cl-HCO− 3 exchange in isolated crypt and villus cells. Am J Physiol 1991; 260: G440–G449
- Varedi M, Chinery R, Greeley GH, Jr, Herndon DN, Englander EW. Thermal injury effects on intestinal crypt cell proliferation and death are cell position dependent. Am J Physiol 2001; 280: G157–G163
- Weiser M. Intestinal epithelial cell surface membrane glycoprotein synthesis I. An indicator of cellular differentiation. J Biol Chem 1973; 248: 2536–2541
- Weston AP, Biddle WL, Bathia PS, BjrMiner P. Terminal ileal mucosal mast cells in irritable bowel syndrome. Dig Dis Sci 1993; 38: 1590–1595
- Yu LC, Perdue MH. Role of mast cells in intestinal mucosal function: Studies in models of hypersensitivity and stress. Immunol Rev 2001; 179: 61–73