Abstract
There is now compelling evidence, coming both from animal and human studies that an early exposure to undernutrition is frequently associated with low birth weight and programs HPA axis alterations throughout the lifespan. Although animal models have reported conflicting findings arising from differences in experimental paradigms and species, they have clearly demonstrated that such programming not only affects the brain but also the pituitary corticotrophs and the adrenal cortex. In fetuses, maternal undernutrition reduces HPA axis function and implicates a reduction of placental 11β-HSD2 activity and a greater transplacental transfer of glucocorticoids (GRs). In young adults, usually only fine HPA axis alterations were observed, whereas in older ones, maternal undernutrition was frequently associated with chronic hyperactivity of this neuroendocrine axis. In humans, evidence of HPA axis dysregulation in people who were small at birth has recently emerged. Thus, we suggest that such alterations in adults may be implicated in the aetiology of several disorders related to the metabolic syndrome as well as to immune or inflammatory diseases. To reverse such programming, recent experimental reports have shown that postnatal environmental interventions, dietary modifications and the use of agents modulating the epigenomic state could partly restore physiological functions and thus open new therapeutic strategies.
Introduction
Undernutrition is one of the most prevalent conditions affecting humans in both developed and developing countries in the world. According to the Food and Agriculture Organisation (FAO), there are some 800 million people in the world who do not have enough to eat to supply their basic energy needs. Hunger and undernutrition prevent the normal growth and development of children. Recent surveys report that approximately 40% of the world's children below 5 years of age are significantly underweight, primarily because of inadequate nutrition (FAO/WHO Citation2001). In humans, moderate to severe periods of undernutrition prior to the first 2 years of life are known to be associated with behavioral deficits and delays in cognitive development and poor school performance. These deficits can persist despite nutritional rehabilitation for as long as 20 years after the original episode (Galler et al. Citation1997). It has been proposed that the human fetus and infant can respond to unbalanced nutrition or other adverse influences by changing their developmental and growth pattern (Gluckman and Hanson Citation2004a). This developmental plasticity may, however, play a role in the generation of human diseases (Gluckman and Hanson Citation2004b). This phenomenon, termed “programming”, has led to the theory of “fetal origins of adult disease” in humans (Barker Citation1998). Namely, alterations in fetal nutrition and endocrine status may result in developmental adaptations that permanently change morphology, physiology and metabolism of the offspring, thereby predisposing individuals to metabolic, endocrine, and cardiovascular diseases in adult life. Although the physiological mechanisms involved in such fetal programming remain largely unknown, the development of several animal models has allowed study of these alterations. In particular, animal models mimicking an overexposure of the fetus to GRs, such as maternal stress or dexamethasone injection during gestation strongly suggest that elevated maternal GRs could play a key role in fetal programming (Levitt et al. Citation1996; Langley-Evans Citation1997; O'Regan Citation2001; Edwards et al. Citation2001; Edwards and McMillen Citation2001; Bertram and Hanson Citation2002; Maccari et al. Citation2003; Lesage et al. Citation2004; Seckl and Meaney Citation2004; Woods and Weeks Citation2005). Numerous studies have clearly established that several different perinatal manipulations, including both prenatal (maternal stress, exposure to synthetic GR or alcohol, immune challenge, low-protein diet, maternal undernutrition) and postnatal manipulations (neonatal handling, modified maternal behavior, infection, exposure to synthetic GR, maternal undernutrition), can program hypothalamic–pituitary–adrenal (HPA) axis function in adult primates, guinea pigs, sheep and rodents (Weinstock et al. Citation1992; Clarke et al. Citation1994; Reul et al. Citation1994, Citation2004; Henry et al. Citation1994; Ogilvie and Rivier Citation1997; Clark Citation1998; Koehl et al. Citation1999; Vallee et al. Citation1999; Lesage et al. Citation2001; Chisari et al. Citation2001; Lingas and Matthews Citation2001; Liu et al. Citation2001; Ordyan et al. Citation2001; O'Regan et al. Citation2001; Phillips Citation2001; Jezova et al. Citation2002, Citation2002a, Matthews Citation2002; Leonhardt et al. Citation2002; Sebaai et al. Citation2002; Bloomfield et al. Citation2003; Zhang et al. Citation2005; Kapoor and Matthews Citation2005). However, the phenotype of HPA function following early manipulation depends on several parameters such as the animal species, the timing and intensity of the stress, the nature of the stressor and the gender of the fetus or neonate. For example, using prenatal maternal stress as a model it has been shown, in rats, that both basal ACTH and pituitary–adrenocortical responses to stress are increased in female offspring whereas basal HPA activity remains unaffected and responses to stress are reduced in male offspring (McCormick et al. Citation1995). The wide spectrum of experimental paradigms has give rise to highly variable results that are difficult to interpret. Therefore, this review will be solely dedicated to the effects of perinatal maternal undernutrition on the offspring HPA axis. Since few studies dealing with human populations have been reported, we will focus largely on results of animal models of protein-calorie undernutrition to provide detailed information on the effects of early undernutrition on the HPA axis during development and throughout life. We will conclude with a discussion of the significance of these findings and recommendations for future research.
Undernutrition, growth and brain development
Maternal undernutrition during gestation reduces placental and fetal growth in both animals and humans (Barker and Clark Citation1997; Bell and Ehrhardt Citation2002). Several recent lines of evidence suggest that fetal growth is most vulnerable to maternal dietary deficiencies of nutrients (e.g. protein and micronutrients) during the peri-implantation period and during rapid placental development (Wu et al. Citation2004). However, undernutrition in fetuses may not only result from low maternal intake of dietary nutrients, but could also be due to placental insufficiency, both producing reduced transfer of nutrients to the fetus (Bell and Ehrhardt Citation2002). In addition, one cannot dismiss the consequences of early or closely-spaced pregnancies in fetal undernutrition (King Citation2003). Intrauterine growth retardation (IUGR), which may result from fetal undernutrition, represents a specific adaptation to the conditions of fetal life. This immediate adaptive response is not only the consequence of the effects of undernutrition in utero, but is also a mechanism by which the fetus uses an early environmental cue to “predict its future” and adopts a developmental pathway that might best suit its expected adult environment (Gluckman et al. Citation2005). However, when these in utero physiological homeostatic mechanisms to match this predicted postnatal environment are mismatched with actual environment conditions in later life, the organism is left with a postnatal physiology that is inappropriate, putting it at increased risk to later develop diseases (Barker and Clark Citation1997; Barker Citation1998; Hoet and Hanson Citation1999; Edwards et al. Citation2001a; Holemans et al. Citation2003; Szitanyi et al. Citation2003; Gluckman et al. Citation2005). Predictive responses can be also induced during the early postnatal period as previously recognized in the case of human infant thermal environment which leads to determination of active sweat glands (Kawahata and Sakamoto Citation1951).
The availability of nutrients plays a critical role in the maturation of the brain and in the development of optimal cognitive capacity (Galler et al. Citation1997). For example, as diet-derived amino acids are essential for the synthesis of structural proteins, enzymes, neuropeptides, and neurotransmitters (Rassin Citation1987), protein-restriction during the perinatal period will affect numerous factors implicated in anatomy, chemistry, neurophysiology or behavior. However, to evaluate precisely the consequences of malnutrition on the developing brain, four important factors must be considered: the type, the severity, the timing and the duration of the nutritional insult. Additionally, the roles of environmental and genetic factors are also crucial.
We will restrict this discussion largely to protein-calorie undernutrition, which is the most common condition found in human populations (Galler and Ross Citation1993). The severity and the timing of undernutrition constitute particularly important factors that determine the long-term consequences of food restriction (FR) on brain functions (see discussion of vulnerable period below). Finally, concerning duration, this factor also has impact on long-term consequences since undernutrition could be episodic or present over extended periods during the life. We will focus here on consequences of perinatal undernutrition exposure.
Notion of vulnerable period
Any early insult is thought to have its most severe impact during the period of rapid brain “growth” that differs greatly among species. These species differences must be considered when comparing the consequences of malnutrition between studies. As shown in (A), the major brain growth period in humans extends from the second trimester of gestation through the first 18–24 months of postnatal life. In rats, it begins prenatally and peaks during the first 3 weeks after birth. In contrast, the guinea pig gives birth to mature young, as extensive neurological and neuroendocrine development occurs during fetal life (Matthews Citation1998). During these periods, changes induced by undernutrition are permanent and could perturb numerous brain developmental events such as neurogenesis, gliogenesis, cell migration and differentiation. All these alterations have been particularly well documented in the hippocampus (for review see Galler et al. Citation1997). However, since specific brain regions in every species have their own developmental “timetables”, undernutrition could also differently affect developmental events in the various parts of the brain before, and may be after, this period of rapid brain growth.
Figure 1 (A) Comparison of the periods of brain growth and development (indicated by the velocity curves) in human and rat. The major developmental cellular processes are also shown. Note the shift of events to the early postnatal period in rats. This schema was adapted from Galler et al. (Citation1997). Below (B) is indicated the FR50 experimental model. In FR50 rats, daily maternal food intake is reduced by 50% from the last week of gestation until the end of lactation.
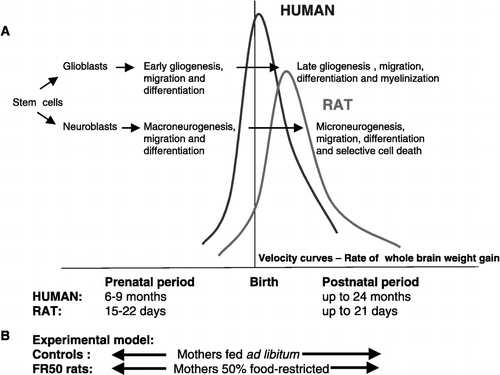
Our research program has dealt with examining the effects of a severe perinatal maternal FR in rats. A 50% food-restricted diet is provided to the pregnant female, starting at day 14 of gestation (E14 for fetuses) until the end of lactation (PND21 for offspring), whereas control pregnant females are provided an ad libitum diet (). This procedure imposes undernutrition during the perinatal period on the assumption that this timeframe corresponds to the period of maximal vulnerability of brain development in this species. This approach, useful in ascertaining how malnutrition affects brain anatomy, chemistry and physiology in the animal, has, however, the disadvantage of malnourishing the mothers, which could in consequence alter their maternal behavior during lactation.
Since cortisol and its related HPA regulatory pathways could be characterized as resource allocators rather than simply stress mediators, the quality of early nutritional environment could be associated with adaptive adjustments in the HPA axis during the entire lifespan of an individual, e.g. from fetal to adult stages. Accordingly, a literature over several decades has documented the effects of early environment on subsequent HPA functions (Levine Citation1957). Interestingly, GRs are suspected to be, at least in part, responsible for the metabolic syndrome (Seckl Citation1997; Björntorp Citation1995) which is correlated with low birth weight (Gluckman et al. Citation2005). In the sections below, we will develop evidence about how early malnutrition alters the structure and function of the HPA axis from an early developmental stage (in the fetus) until adulthood.
Maternal undernutrition and the HPA axis in the perinatal period
Glucocorticoids, fetal development and the ontogeny of the HPA axis
During the second half of pregnancy, the fetal HPA axis is essential for the development, maturation and homeostasis of the fetus, and also to prepare for the survival of the neonate (for review see Freemark Citation1999). For example, in the late gestational period, the fetal adrenal gland plays a pivotal role. A surge of fetal GR production near term (Brooks et al. Citation1996) increases the expression of surfactant proteins B and C in the fetal lung, reduces interalveolar wall thickness and increases potential airspace volume and lung compliance (Hooper and Young Citation1998), which prepare the fetus for independent respiration after birth. GRs enhance the conversion of thyroxine (T4) to triiodothyronine (T3) and increase the activity of adrenomedullary phenylethanolamine N-methyltransferase, which catalyzes the methylation of norepinephrine to epinephrine (Brooks et al. Citation1996; Jaffe et al. Citation1998). Striking increases in release of these hormones at birth activate lipolysis in brown and white adipose tissue, providing heat and energy. In the fetal liver, GRs increase the storage of glycogen, which provides an important source of glucose immediately after delivery. GRs induce expression of the gluconeogenic enzyme glucose-6-phosphatase (Fowden et al. Citation1995), which maintains normoglycemia during the postnatal period of intermittent food intake. GRs also accelerate the differentiation of fetal gastric epithelial cells and regulate the processing of lipoproteins in the fetal jejunum (Loirdighi et al. Citation1997), facilitating the shift to a high fat, milk-based oral diet. Finally, it was reported that GRs increase the expression of growth hormone (GH) as well as prolactin (PRL) receptors in fetal sheep liver (Li et al. Citation1996; Phillips et al. Citation1999). These findings indicate that GRs are implicated in the control of growth as well as in hepatic carbohydrate and lipid metabolism in early life.
In the rat, fetal HPA axis development is taking place during the second half of gestation (review in Grino et al. Citation1995). Although the adrenal glands of embryonic day 13 (E13) fetuses can synthesize and secrete corticosterone, their regulation by the adrenocorticotropic hormone (ACTH) appears only at E16–E17. Then, plasma corticosterone levels rise progressively, reaching a peak at E19. Anterior pituitary proopiomelanocortin (POMC) expression, first detected at E15, rises between E17 and E21, and parallels pituitary ACTH content (Grino et al. Citation1989). During late gestation, the secretion of ACTH by corticotroph cells is already regulated by hypothalamic corticotrophin-releasing hormone (CRH) and arginine vasopressin (AVP). CRH mRNA is first detected at E17 in the hypothalamic paraventricular nucleus (PVN), then levels of CRH mRNA increase until E19 and decrease sharply until E21 (Grino et al. Citation1989). Before E18, as neurosecretory axons are not connected with the primary portal capillary plexus (Ugrumov et al. Citation1985), it is believed that CRH may enter the hypophysial portal circulation after intercellular diffusion in hypothalamic tissue. AVP mRNA, first detected in the PVN at E18, increases from E18 to E21 (Grino et al. Citation1995). Finally, concerning the negative feedback control exerted by corticosterone, it was reported that pharmacological fetal adrenalectomy, obtained by treating the mother with metyrapone, does not change CRH mRNA levels in the PVN of E17–E18 fetuses, suggesting that GR regulation of CRH-synthesizing cells is not mature before E18 (Baram and Schultz Citation1992). Thereafter, the efficiency of this negative feedback has been demonstrated; it acts via intracellular mineralocorticoid (MR) and GR receptors activation (Diaz et al. Citation1998). GR mRNA is highly expressed throughout the brain from mid-gestation, which coincides with the activation of the fetal HPA axis, whereas MR gene expression is only observed during the last 3 days of gestation and is particularly prominent in the hippocampus and in the lateral septum (Diaz et al. Citation1998).
The fetal glucocorticoid programming hypothesis
Programming reflects the ability of factors, acting during a defined developmental stage, to exert organizational effects that persist throughout life. Steroids are ideally suited to exert these actions. This is clearly illustrated by the well-described action of androgens to program androgen-metabolizing enzyme expression in the liver, in the development of sexually dimorphic structures in the brain, and in sexual behavior in many vertebrate species (reviewed in Resko and Roselli Citation1997). Numerous observations suggest that GRs might be implicated in the genesis of adult cardiovascular and metabolic diseases due to restricted fetal growth (Seckl Citation1997). Indeed, fetal cortisol levels are increased in some human fetuses showing IUGR (Goland et al. Citation1993) and excess of GRs has been strongly correlated with delays in fetal growth in both animals and humans (Reinish et al. Citation1978; Seckl Citation1997). Moreover, several studies have shown that exposing fetuses to high levels of GRs reduced birth weight and caused permanent hypertension and hyperglycaemia in the adult rat (Benediktsson et al. Citation1993; Lindsay et al. Citation1996a; Nyirenda et al. Citation1998). Thus, alterations of the fetal HPA axis may represent a primary means by which fetal growth restriction could program adult chronic diseases.
In both animals and humans, the development of the HPA axis in fetuses is protected from the influence of maternal GRs by placental 11β-hydroxysteroid dehydrogenase type 2 (11β-HSD2), which catalyzes the rapid metabolism of corticosterone and cortisol to inert 11-keto products, namely 11-dehydrocorticosterone and cortisone (Murphy et al. Citation1974; Seckl Citation1993). This protective placental enzymatic barrier ensures that most maternal cortisol is inactivated upon crossing to the fetus, in such a way that it is considered that about 75% of the active cortisol in the human fetal circulation at term is derived from the fetal adrenals (Beitins et al. Citation1973). Furthermore, it has been demonstrated in rats that a deficiency of placental 11β-HSD2, which increases fetal GRs exposure, retards growth and programs responses leading to diseases in later life (Seckl Citation1997). Reduced placental 11β-HSD2 expression and activity has also been reported in some babies with low birth weight (Stewart et al. Citation1995). In rats, treatment of pregnant mothers with carbenoxolone, an 11β-HSD2 inhibitor, causes IUGR and produces permanent hypertension and insulin resistance in the offspring (Lindsay et al. Citation1996a,Citationb).
In conclusion, regarding the GR load on the fetus, both maternal GR levels and placental 11β-HSD2 activity represent critical factors for fetal HPA axis development and modulate its future capacity to respond to stimuli.
Effects of maternal undernutrition on the fetal HPA axis
As previously mentioned, protein-calorie malnutrition, which is the most common condition found in human populations (Galler and Ross Citation1993), dramatically disturbs the energy balance of the organism. One of the crucial endogenous responses to preserve this balance is the activation of the HPA axis. Indeed, it is well established that food availability influences both the rythmicity and the activity of this axis. Starvation and FR stimulate HPA axis activity in both humans (Fichter and Pirke Citation1986; Gold et al. Citation1986) and rats (Stewart et al. Citation1988; Belda et al. Citation2005). However, the mechanisms by which FR activates the HPA axis remain not fully understood. The mechanisms could necessitate an increase in ACTH secretion (Jacobson et al. Citation1997) as well as an enhanced adrenal sensitivity to ACTH (Dallman et al. Citation1978; Han et al. Citation1998). Thus, in association with malnutrition, putative increased GR exposure in FR fetuses could disturb the development of their HPA axis.
The effects of maternal undernutrition on fetal HPA axis development have been especially well-studied in sheep. In this species, it is well established that the normal timing of parturition (around day 147 of gestation) is dependent on a prepartum activation of the fetal pituitary–adrenal axis. In a series of studies, Hawkins et al. (Citation2000a,Citationb, Citation2001) have shown that a 15% reduction in maternal food intake, during the first 70 days of gestation, reduces HPA function in fetuses at 126–130 days of gestation. In these animals, although the fetal ACTH response to CRF and AVP was unaffected, cortisol responses were reduced significantly. Finally, at the molecular level, it was demonstrated that both CRF and preproenkephalin mRNA expression in the PVN, as well as anterior pituitary GR mRNA expression were reduced in fetuses from nutrient restricted ewes. Some other studies, also performed in sheep, have shown that a 50% maternal nutrient restriction performed during the period of rapid placental growth (i.e. 28–77 days of gestation) decreased placental 11β-HSD2 expression and activity in mid-gestation, but increased placental weight in newborn offspring without affecting their body weight (Whorwood et al. Citation2001). In their adrenals, GR and type 1 angiotensin receptor (AT1) mRNA expression was increased whereas 11β-HSD2 mRNA was reduced (Whorwood et al. Citation2001). It was suggested that the early alterations of the HPA axis could play a role in mediating the elevation of arterial blood pressure observed in lambs exposed to undernutrition.
In others studies, McMillen and Edwards (reviewed in McMillen et al. Citation2004) have investigated the effects of maternal periconceptional undernutrition associated or not with the maintenance of undernutrition for the remainder of pregnancy. They showed that maternal periconceptional undernutrition (30% food-restriction, from 60 days prior to mating until 7 days after mating) results in stimulation of the fetal pituitary–adrenal axis in late gestation, and that this effect is not reversed by providing a maintenance control diet from the second week of gestation (Edwards et al. Citation2002a). They also demonstrated that both the number and sex of fetuses have an impact on the timing of the prepartum activation of the HPA axis (Edwards and McMillen Citation2002b). This early activation involves both an increase in ACTH secretion and an overexpression of steroidogenic acute regulatory proteins in the adrenal gland of sheep fetuses (Edwards et al. Citation2002b). Finally, the acceleration of fetal HPA axis maturation induced by maternal periconceptual undernutrition results in preterm labor of 50% of pregnant sheep (Kumarasamy et al. Citation2005). Phillips et al. (Citation1996) have investigated the impact of a reduction of placental substrate delivery to sheep fetuses during the entire gestational period. They showed that the plasma cortisol level was increased in placentally restricted fetuses only after day 130 of gestation. Moreover, although anterior pituitary POMC mRNA expression level was reduced at term in these animals, the plasma ACTH level was not affected whereas the adrenal mass was increased. The authors concluded that the trigger for this developmental change may be mediated through a resetting of the fetal HPA axis.
In rats, to our knowledge only one study, performed in our laboratory, has investigated the effects of maternal FR on the HPA axis in fetuses (Lesage et al. Citation2001). In this study, pregnant mothers were subjected to a 50% food deprivation (FR50) during the last week of gestation (from E14 to E21). We showed that such food reduction in mothers increased both the plasma ACTH and corticosterone levels from E19 to E21. At term (E21), their adrenal weight was increased and their plasma corticosteroid-binding globulin (CBG) level was diminished. CBG is a plasma corticosterone carrier, which is usually reported to limit the amount of plasma free corticosterone, hence controlling the plasma level of the biologically active form of this hormone. The mechanism by which maternal FR reduces plasma CBG level at term remains unknown but our results suggest that FR50 during late gestation stimulates maternal HPA axis activity and increases the level of the free fraction of corticosterone in the maternal compartment, and thus may facilitate its transplacental passage. At term, we also reported that maternal FR50 decreased 11β-HSD2 gene expression in the placenta, suggesting that placental 11β-HSD2 activity could also be reduced and may thus participate in fetal overexposure to maternal GRs (Lesage et al. Citation2001). Such an effect was reported in rats in response to maternal dietary protein restriction (Langley-Evans et al. Citation1996). In humans, a low placental 11β-HSD2 activity has been observed in some babies with low birth weights (Stewart et al. Citation1995). Accordingly, the body weight of FR50 fetuses was reduced at term demonstrating that maternal FR50 is indeed a good model of IUGR in the rat. In addition, the HPA axis in FR50 fetuses also showed numerous alterations such as adrenal atrophy, reduced GR and MR gene expression in the hippocampus, reduced CRH mRNA levels in the hypothalamic PVN and drastic reduction of plasma ACTH level. However, although maternal FR50 induces both adrenal atrophy and HPA axis hypoactivity, the plasma corticosterone levels were increased in the FR50 rats at birth. This observation is in agreement with previous results which suggest an increased transfer of GRs to the fetus exposed to maternal FR. Low activity of the HPA axis in newborns exposed to maternal FR50 was also suggested by the lack of increasing circulating levels of corticosterone 2 h after birth, which is an adaptation to birth. In this study, we also evaluated the effects of hypersecretion of maternal corticosterone, induced by the FR, on the disturbances on both fetal growth and HPA axis activity. Using both adrenalectomy and corticosterone supply to maintain normal basal plasma levels of corticosterone in mothers, we showed that this procedure abolished all the alterations of the HPA axis in fetuses exposed to maternal FR without affecting placental 11β-HSD2 gene expression or body weight at term. These data showed that the hypoactivity of the fetal HPA axis is related to the negative feedback control exerted by high circulating levels of corticosterone arising from the maternal compartment. In contrast, the reduced placental 11β-HSD2 mRNA level and body weight at term imply direct effects of maternal FR or indirect actions via disturbances in maternal and/or placental metabolism. For example, we also showed that maternal FR50 reduced at term the placental transfer of glucose to the fetus (Lesage et al. Citation2002b). Our findings on the mechanisms and alterations induced by maternal FR on the HPA axis of fetuses are summarized in and .
Figure 2 Effects of 50% food restriction during the last week of gestation on maternal and fetal HPA axis relationship in late pregnancy. Food-restriction of pregnant rats stimulates their HPA axis activity. Both plasma ACTH and corticosterone levels are increased and maternal adrenal glands are hypertrophied at the end of gestation. Maternal undernutrition also reduces both plasma CBG levels and placental 11β-HSD2 gene expression at term. As a result, rat fetuses are overexposed to high levels of free fraction of corticosterone arriving from the maternal compartment, thus potentiating the glucocorticoid negative feedback on the fetal brain and disturbing the development of their HPA axis. At the end of gestation (E21), fetuses show numerous alterations such as a drastic adrenal atrophy, reduced plasma ACTH and corticosterone levels and diminished gene expression affecting hippocampal MR and GR, hypothalamic CRH and anterior pituitary POMC. Hipp, hippocampus; PVN, hypothalamic paraventricular nucleus; Ap, anterior pituitary; Ac, adrenal cortex; MR, mineralocorticoid receptor; GR, glucocorticoid receptor; CRH, corticotrophin-releasing hormone; POMC, proopiomelanocortin; ACTH, adrenocorticotropic hormone; CBG, corticosteroid-binding globulin; 11β-HSD2, 11β-hydroxysteroid dehydrogenase type 2. (+) = positive/excitatory effect; ( − ) = negative/inhibitory effect. Based on data reported in Lesage et al. (Citation2001).
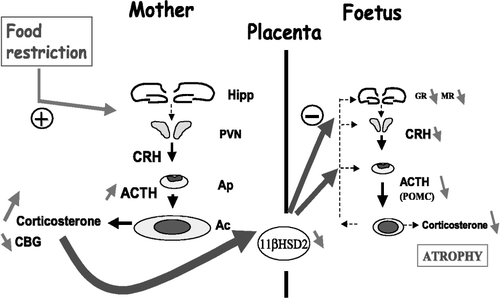
Table I. Effects of perinatal maternal undernutrition (FR50) on growth and HPA axis parameters in male rat offspring under basal conditions across the lifespan.
Effects of perinatal maternal undernutrition on the HPA axis at weaning
Since it has been previously shown that prenatal maternal stress or dexamethasone injection during gestation has long-term consequences on the HPA axis activity in the offspring (for review see Andrews and Matthews Citation2004), we evaluated the effects of maternal FR50 in animals at weaning (post-natal day 21: PND21). Rats were exposed to maternal FR50 from E14 to PND21, the period corresponding to the increase in brain weight in this species. In FR50 offspring at weaning, the body weight as well as the weight of the adrenals, liver and thymus were reduced (Léonhardt et al. Citation2002). Under resting conditions, although FR50 offspring displayed normal total plasma corticosterone levels, their plasma ACTH and CBG levels were diminished, and the latter contributed to an increase in the free corticosterone concentrations (). In the hippocampus, these animals showed increased MR and GR gene expression. Finally, after an ether inhalation stress, the increase in plasma ACTH was lower than in controls, and the increase in plasma corticosterone returned to values lower than basal level 90 min after this stressful procedure. Chisari et al. (Citation2001) have also demonstrated in weaned rats (21-days-old) that a severe maternal undernutrition during the entire gestation period until the end of lactation dramatically reduces the body weight gain of offspring, and diminishes the ACTH secretory response to an insulin injection. However, both basal plasma ACTH and corticosterone levels were unaffected. Altogether, these findings suggest that perinatal maternal FR reduces the HPA axis activity in offspring at weaning. Moreover, in our model, as FR50 rats have increased circulating free corticosterone concentrations and probably more hippocampal MR receptors, they showed a reinforced and prolonged rapid negative feedback of free corticosterone on HPA axis activity in response to stress.
Plasma ACTH and corticosterone levels are low during the first 2 weeks of postnatal life and increase thereafter to reach adult-like values around PND21 (Grino et al. Citation1995). In weaned FR50 rats, the plasma ACTH level at rest was reduced whereas plasma corticosterone was not affected. This suggests that the developing rat adrenal gland is more sensitive to the stimulatory effect of pituitary ACTH or that corticosterone secretion is, at least in part, independent of ACTH stimulation. In these rats, pituitary POMC gene expression was not reduced, suggesting that FR50 may alter POMC processing and that peptides distinct from ACTH, but derived from the POMC molecule, may play a role in the secretion of corticosterone. Indeed, a potentiation of the adrenal steroidogenic activity of ACTH by γ3-MSH has been demonstrated in vivo in adult rats (Pedersen et al. Citation1980) and in vitro in humans (Al Dujaili et al. Citation1981). In the developing rat, pretreatment with Lys-γ3-MSH potentiates the effect of an acute ACTH injection (Oliver et al. Citation1994). Interestingly, it has previously been shown that a dramatic decrease in ACTH production resulting from a greater extent of ACTH cleavage into smaller product peptides such as α-melanocyte-stimulating hormone (α-MSH) and corticotrophin-like intermediate lobe peptide (CLIP) occurs between the second and third postnatal weeks in rat corticotroph cells (Noel and Mains Citation1991). In view of this observation, it would be tempting to speculate that maternal FR50 delays the maturation of the pituitary corticotroph cell. In weaned FR50 rats, the level of free corticosterone was raised at rest leading to increased delivery of the hormone to target tissues. However, hypothalamic CRH peptide and mRNA expression in the PVN were not affected. These observations suggest that the regulation of the CRH cell bodies in the PVN is not mature in weaned FR50 rats. This immaturity of the regulation of CRH expression does not seem to result from a defect of the neuron itself, since low doses of dexamethasone are able to down-regulate CRH gene expression in the PVN of PND7 rats (Grino et al. Citation1995), but rather to an alteration of the factors that regulate the CRH neurons. These findings imply, at this stage, disturbances of several factors such as excitatory amino acids and central biogenic amines, namely catecholamines and serotonin. These alterations could also be implicated in the increased hippocampal MR gene expression in FR50 offspring, but could also result from an increased GR exposure through the milk of FR mothers. Indeed, it was reported that offspring of mothers whose drinking water is supplemented with corticosterone, from PND1 to weaning, exhibit an increase in hippocampal corticosterone-binding sites (Catalani et al. Citation2000). Finally, the alterations of the HPA axis observed in offspring at weaning could also be programmed, in part, by significant changes in the mother-pup relationship, characterized by increased suckling, contact between mothers and pups, and time spent in the nest (Galler et al. Citation1984). Thus, these changes may modify any differences in HPA axis development thought to be attributable exclusively to undernutrition.
Programmed HPA axis in adult offspring
Few studies have examined the effects of maternal undernutrition on the HPA axis activity in the adult offspring. Using the sheep as a model, Bloomfield et al. (Citation2003), have performed an elegant study in which pregnant ewes were undernourished (75% FR) for 10 days (UN10) or 20 days (UN20) from day 105 of gestation (term 146 days). Although the birth weight of UN10 lambs was not different from ad libitum fed controls, UN20 lambs were lighter at birth, though there were no significant differences in weight at 30 months. The HPA axis activity was not altered in the 30-month-old female offspring born from UN20 mothers, both under resting conditions and in response to an insulin tolerance test or CRH/AVP challenge. In contrast, the UN10 female offspring displayed increased ACTH plasma levels in response to CRH/AVP injection without any modification in their corticosterone or CBG plasma levels. To explain the differences observed between UN10 and UN20 adult animals, the authors speculated that a brief period of severe undernutrition in late gestation leads to a down-regulation of the HPA axis as an adaptive mechanism whereas a more prolonged insult may result in secondary adaptive mechanisms resulting in up-regulation. Accordingly, another group has also shown in sheep that undernutrition for 30 days results in an elevation of maternal circulating cortisol concentrations during the first ten days (Edwards and McMillen Citation2002b). In contrast, the maternal plasma and umbilical cord plasma cortisol concentrations were reduced after 20 days of undernutrition. Nevertheless, these data provide evidence that not only duration of the insult but also the time when the maternal FR is applied is important in programming postnatal physiology irrespectively of birth weight. Using the guinea pig as a model, Lingas et al. (Citation1999) studied the effects of a very short (48 h on gestational days 50 and 51; term almost 70 days) maternal food deprivation, during a period of maximal brain growth which is also associated with rapid neuroendocrine development, on the HPA activity in young adults (Lingas and Matthews Citation2001). The authors had previously shown that, during this critical period of development of GR and MR systems, the 48 h nutrient restriction reduced both hippocampal and hypothalamic GR expression (Lingas et al. Citation1999). In nearly 3-month-old animals, HPA axis function is still altered but in a sex-specific manner (Lingas and Matthews Citation2001). Although both males and females exhibited IUGR, female offspring showed hyperactive adrenocortical function whereas males exhibited reduced HPA activity under resting conditions. Interestingly, in response to various HPA axis stimuli (CRH, ACTH and hypoglycaemic challenges) the net cortisol response was identical in control offspring and those born to FR mothers indicating that the HPA axis was responding similarly, in both males and females, but from a different baseline. However, in contrast to several other fetal programming models, the authors found no effect of maternal nutrient restriction on blood pressure or heart rate in both male and female adult offspring (Lingas and Matthews Citation2001). Nevertheless, since their experiments were performed in young adults, it remains possible that the animals were too young to develop “programmed” hypertension, since this is usually amplified as the animals get older.
Using maternal undernutrition induced by a 75% reduction of food intake on gestational days 13–20, Jezova et al. (Citation2002), studied HPA axis activity in young adult rats (3 months-old) both under resting conditions and in response to a mild stressor (brief handling). They showed that maternal undernutrition did not markedly alter basal and stress-induced ACTH or corticosterone plasma levels, although the increase in plasma ACTH concentration in response to the stress of brief handling was more pronounced in rats prenatally stressed by maternal FR compared to that in controls. They also demonstrated that hypothalamic CRH and anterior pituitary POMC mRNA levels were not affected under basal conditions by maternal undernutrition suggesting that a drastic FR performed during the last week of gestation had no clear programming effects on HPA axis activity in young adult rats. Accordingly, Chisari et al. (Citation2001) have shown that a 50% maternal FR during gestation and lactation did not modify ACTH and corticosterone plasma levels in 2-month-old male rats under resting conditions. However, the perinatally food-restricted animals showed a reduced HPA axis response to insulin-induced hypoglycemia suggesting that the deleterious effect of undernutrition, during gestation and lactation, appears to be irreversible. The differences in the results from these two studies could be a consequence of the gestational stress paradigm used or a reflection of the variability in the responses to different stress stimuli applied to the offspring to test the activity of the HPA axis.
To circumvent this problem, we used the same model (FR50) from birth to adulthood, to study the effects of maternal undernutrition on the HPA axis activity in the rat offspring. In accordance with the previous studies (Chisari et al. Citation2001; Jezova et al. Citation2002), we found that maternal undernutrition did not affect basal ACTH nor corticosterone plasma levels in 4-month-old rats (Sebaai et al. Citation2002). In the hypothalamus, maternal FR failed to modify CRH and AVP mRNA expression. However, in FR50 animals some fine alterations were observed such as elevated hippocampal MR/GR mRNA ratio and anterior pituitary POMC level suggesting that maternal FR has long-term programming effects on the offspring HPA axis activity although it does not markedly disturb it, at least under resting conditions (). As expected, in response to a 72-h water deprivation period, the HPA axis activity was strongly stimulated in control animals (). In contrast, in FR50 animals, although the bound and free corticosterone plasma levels were increased to the same extent as in control rats, several alterations were observed. In particular, the rise in plasma ACTH, anterior pituitary POMC mRNA expression, hypothalamic AVP mRNA level and the decrease in hippocampal MR/GR ratio were not seen (). These data demonstrate that maternal FR does not markedly affect the HPA axis activity in young adult animals under resting conditions, but alters their capacity to cope well with the dehydration stress. Since several studies have shown that HPA axis activity is profoundly modified throughout the lifespan and that the aging process promotes its hyperactivity (Sapolsky et al. Citation1986; Scaccianoce et al. Citation1990; Workel et al. Citation2001), we hypothesized that the effects of maternal FR on HPA axis activity would be more pronounced during late rather than early adulthood. To test this hypothesis, we compared HPA axis activity both under basal conditions and after a 72-h water deprivation period in 8-month-old male rats malnourished during their perinatal life and in age-matched control animals (Sebaai et al. Citation2004). As previously observed in 4-month-old rats under basal conditions, 8-month-old FR50 animals showed an enhanced MR/GR mRNA levels ratio in the hippocampus, as well as increased POMC mRNA levels in the anterior pituitary. However, numerous alterations that were not observed in young adults appeared in mature rats (). Bound and free corticosterone plasma levels were very high in spite of normal ACTH levels suggesting either that the adrenal cortex is hypersensitive to the ACTH stimulation or that corticosterone secretion is, at least in part, independent from ACTH action. Using binding and perifusion experiments, we found that ACTH binding was similar between controls and FR50 rats, and that FR50 adrenal glands still secrete high levels of corticosterone in the absence of ACTH, suggesting that the excessive corticosterone secretion observed in FR50 animals does not require the action of ACTH (unpublished data). Interestingly, in spite of high plasma corticosterone levels POMC mRNA expression was increased in the FR50 anterior pituitary, indicating that the negative feedback usually exerted at the pituitary level is altered (Sebaai et al. Citation2004).
Table II. Effects of a 72-hour dehydration on HPA axis parameters in adult male control (C) and FR50 rats.
To get more insight into the altered GR feedback at the pituitary level in the 8-month-old FR50 rats, we performed corticosterone receptor binding experiments. Although GR binding was not affected by maternal FR, both binding to MR and MR mRNA expression were strikingly increased in the FR50 pituitaries when compared to controls (unpublished data). In human pathology a lack of GR feedback at the pituitary level is frequently encountered in corticotroph adenomas responsible for Cushing's disease. Using RT-PCR we found that MR is expressed in normal human pituitaries but also in a large number of corticotroph adenomas (unpublished data). Although the presence of pituitary MR has already been reported (Hugin-Flores et al. Citation2003, Citation2004), its physiological function remains poorly understood. It was postulated that increased number of MR receptors could buffer the excessive brain drive on the pituitary during chronic stress or pathological conditions associated with high GRs levels (Hugin-Flores et al. Citation2004). Our results showing MR expression in the tumoral corticotropes suggest that the increased MR/GR ratio observed in the FR50 pituitary may contribute to the altered GR feedback in these animals. In addition, the high POMC mRNA level observed in FR50 anterior pituitary was not correlated with ACTH plasma levels suggesting that maternal FR might alter ACTH secretion and/or POMC processing. Interestingly, we demonstrated that prohormone-convertase 2 (PC2) mRNA expression is significantly increased in the anterior pituitary of 8-month-old FR50 animals (Sebaai et al. Citation2004). Since the latter enzyme is involved in processing ACTH into smaller products, namely αMSH and CLIP, it could be speculated that perinatal maternal undernutrition could be responsible, in part, for a shift in anterior pituitary POMC processing similar to that observed in melanotrope cells and in pituitary corticotroph adenomas (Coates et al. Citation1989). In summary, under resting conditions, maternal FR programs hyperactivity of the HPA axis in 8-month-old male offspring.
After a 72-h water deprivation not surprisingly HPA axis activity is stimulated, but only in control animals (). It is noteworthy that corticosterone plasma levels were not affected in dehydrated FR50 rats in spite of increased circulating levels of ACTH, suggesting that the adrenal sensitivity to ACTH may have been decreased. Interestingly, FR50 rats have basal corticosterone levels comparable to those observed in controls after water deprivation. This may indicate that in FR50 rats, basal corticosterone secretion is maximal and thus could not be increased by dehydration even in the presence of elevated plasma ACTH levels, demonstrating that mature FR50 animals are unable to cope with dehydration.
Together, animal studies clearly indicate that maternal undernutrition has both short- and long-term programming effects on the HPA axis in the offspring, and that disturbances do not just affect the brain but also the anterior pituitary corticotrophs and the adrenal cortex. In fetuses, exposure to maternal undernutrition during gestation reduces HPA axis function in both the sheep and rat. In FR rat fetuses, the reduced HPA axis function is associated with both a reduction in placental 11β-HSD2 activity and a greater transplacental transfer of maternal GRs. At weaning, perinatal maternal FR reduces HPA axis activity in response to stress or to hypoglycaemia in rats. In adult animals, conflicting findings with both hypo- or hyper-activity of the HPA axis were reported, evidently arising from differences in experimental paradigms and species. In young adults, only discrete HPA axis alterations are usually observed, whereas in older animals maternal FR is frequently associated with chronic hyperactivity of this neuroendocrine axis.
Human studies
To our knowledge, the evidence that an early exposure to malnutrition or famine programs the HPA axis activity in humans remains to be obtained. Although numerous contradictory results have been published, several studies have shown a close relationship between intrauterine growth disturbance and subsequent HPA axis alterations. Newborn cohort studies have shown associations between birth weight and HPA axis activity throughout the individual lifespan, from infancy to old age (Clark et al. Citation1996; Phillips et al. Citation1998, Citation2000; Phillips and Jones Citation2006). Based upon our experimental data, it can be envisioned that human fetuses could be overexposed to maternal GRs as a consequence of low placental 11β-HSD2 activity, as observed in some babies with low birth weight (Stewart et al. Citation1995), and of increased fetal cortisol levels (Goland et al. Citation1993). This exposure could initially be responsible for later dysfunctions of the HPA axis, as has been demonstrated after maternal stress or fetal synthetic GR exposure (reviewed in Andrews and Matthews Citation2004). A recent study has shown that in children aged 7–9 years old, birth weight is inversely related to salivary cortisol responses to psychological stress in boys, whereas in girls, only morning peak cortisol was inversely related to birth weight (Jones et al. Citation2006). This study demonstrates that events occurring during fetal life resulting in reduced birth weight have sex-specific consequences in pre-pubertal children. In adult men, low birth weight is usually associated with both increased basal- and ACTH-stimulated cortisol secretion (Levitt et al. Citation2000; Reynolds et al. Citation2001). Recently, this hypersecretion of cortisol was also described in response to psychosocial stress in adult subjects with low birth weight (Wust et al. Citation2005). The plasma morning cortisol level is also negatively correlated with birth weight and length (Phillips et al. Citation1998). However, studies that did not detect a clear inverse association between cortisol secretion and birth weight have also been published (Kajantie et al. Citation2003, Citation2004). For example, a reduced ACTH and cortisol response to a combined administration of dexamethasone and CRH in low birth weight subjects has been reported (Ward et al. Citation2004). Although these studies do not allow establishing a clear-cut correlation between low birth weight and HPA axis alterations, they nevertheless provide evidence of dysregulation of the HPA axis in people who were small at birth.
In humans, Cushing's syndrome, a rare disorder characterized by a mortality rate four times that of the general population, is a unique model to elucidate the physiological consequences resulting from exposure to chronic stress-level elevations of endogenous cortisol. Interestingly, the chronic adult disease symptoms encountered in Cushing's patients follow closely those observed in adults born from undernourished mothers. For example, increased blood pressure that has been shown to be programmed by prenatal maternal undernutrition in male rat offspring (Vickers et al. Citation2000; Rivière et al. Citation2005), is also one of the most discriminating features of Cushing's disease as it is present in 80% of patients (Baid and Nieman Citation2004; Sacerdote et al. Citation2005). In addition, type 2 diabetes and obesity, that are also frequently observed in fetal programming animal models (for review see Holemans et al. Citation2003; Gluckman et al. Citation2005), share many metabolic and morphological similarities with Cushing's syndrome (Chrousos Citation2000; Salehi et al. Citation2005; Summers and Nelson Citation2005).
Conclusion and perspective
There is compelling evidence coming both from animal and human studies that an early exposure to undernutrition, frequently associated with low birth weight, programs HPA axis alterations throughout the lifespan. However, as previously mentioned, the wide spectrum of experimental paradigms used to produce malnutrition in animal studies, does not allow establishing a clear-cut and consistent correlation between maternal undernutrition exposure and long-term pathological effects. Nevertheless, in humans, several recent findings have emerged associating low birth weight and increased HPA axis activity in adult life. Because GRs influence the activity of almost every cell in the body, the dysregulation of the HPA axis activity may be implicated in the aetiology of several diseases (for a recent review, see Buckingham Citation2006). Whereas it is clear that an altered HPA axis programming during the perinatal period has long term deleterious effects related to the metabolic syndrome (O'Regan et al. Citation2001; Das Citation2005), there also exist numerous results correlating the incidence of cognitive disorders such as anxiety or depression with HPA axis hyperactivity (Maccari et al. Citation2003). It has been recently shown that low birth weight is positively correlated with the prevalence of schizophrenia suggesting that some neuropsychiatric disorders may also have a developmental origin (Clarke et al. Citation2006). Interestingly several traits of metabolic syndrome have been associated with the use of newer atypical antipsychotics suggesting that patients with schizophrenia may be at greater risk for metabolic disorders (Meyer et al. Citation2005; Henderson Citation2005). In this context, future studies are clearly required to examine to what extent altered HPA axis programming may contribute to the aetiology of schizophrenia.
Moreover, GR hormones play a pivotal role in the cross-talk between the immune and neuroendocrine systems, and are implicated in both immunomodulation and inflammatory processes. It is thus conceivable that maternal perinatal undernutrition, via dysregulation of GR secretion and/or action in the offspring, may contribute to the programming of immune or inflammatory diseases (Shanks et al. Citation1998; Das Citation2005; Karrow Citation2006). Recently, it has been proposed that a common inflammatory pathway underlies the pathogenesis of both cardiovascular disease and type 2 diabetes (Koh et al. Citation2005; Haffner Citation2006). The interface of inflammation, insulin resistance and endothelial activation has become the key to the search for more effective means to treat and prevent the conditions associated with the metabolic syndrome (Wisse Citation2004). For example, increased inflammatory biomarkers in the plasma, such as the liver-derived C-reactive protein (CRP), have been shown to highly correlate with risk for acute coronary syndromes and to metabolic diseases (Lloyd-Jones et al. Citation2006). More recently (Chen et al. Citation2006), it was demonstrated, in humans and mice, that circulating CRP binds to leptin and attenuates its physiological functions. These data suggest that CRP could participate in leptin resistance, which is usually observed in obesity and metabolic diseases. We have recently measured a significant increase in CRP plasma levels in adults born from undernourished mothers (unpublished data). Such observations could open new avenues and suggest that several chronic inflammatory diseases such as asthma, rheumatic diseases, inflammatory bowel disease, psoriasis, ischemia and neurodegenerative disorders may have, at least in part, a neurodevelopmental origin.
The concept according to which several pathologies originate in insults sustained during perinatal life implies that it should be possible to identify and use biological markers that could be uncovered very early before the onset of diseases. At an early stage, the placenta represents an attractive area of research. Indeed this tissue, when associated with IUGR, has been recently shown to present an altered expression of several genes. These include both inflammatory products and imprinted genes (McMinn et al. Citation2005; Myatt Citation2006), suggesting that epigenetic mechanisms may be involved in the long-term programming effects of perinatal alterations, such as those resulting from maternal undernutrition.
It is well known and recognized that epigenetic mechanisms alter gene expression via changes in DNA methylation and/or histone post-translational modifications. Quite interestingly, epigenetic processes have been described for both POMC (Newell-Price Citation2003) and 11β-HSD2 (Alikhani-Koopaei et al. Citation2004) genes. In addition, an elegant study performed by Weaver et al. (Citation2004) has demonstrated that maternal behavior influences the offspring epigenome at a glucocorticoid receptor (GR) gene promoter in the rat hippocampus. The differences observed between the animals submitted to distinct maternal behaviors persisted into adulthood but were abolished by central infusion of a histone deacetylase inhibitor (Weaver et al. Citation2004). Accordingly, it has been shown that both folate and glycine (a factor implicated in DNA methylation) supplementation reverse the effect of a low-protein diet in pregnancy on the blood pressure and vascular function of the offspring (Brawley et al. Citation2003a,Citationb). The demonstration that environmental perturbations during perinatal life affect the epigenomic state, and the fact that several HPA axis parameters are particularly sensitive to such modifications may permit unravelling some of the pathophysiological long-lasting effects of maternal undernutrition in the offspring. More importantly, since it has been shown that these epigenomic changes are potentially reversible, one can envision that the use of agents modulating epigenetic mechanisms could constitute a very novel and exciting therapeutic approach to “deprogram” some chronic diseases.
Finally, recent encouraging data have shown that dietary supplementation may provide an alternate therapeutic option for preventing “programmed” diseases in humans. For example, the use of several dietary approaches that promote oxidant scavenging or increase long-chain omega-3 fatty acids levels attenuate the adverse programming outcomes in rats (Noyan-Ashraf et al. Citation2006; Wyrwoll et al. Citation2006). Moreover, the “quality” of the early environment could also represent a therapeutic strategy. For example, it has been shown that postnatal handling is able to reverse the deleterious effects of prenatal stress on adult brain cell proliferation in hamsters (Kippin et al. Citation2004), and that an enriched housing during adolescence (Morley-Fletcher et al. Citation2003) can counteract the consequences of prenatal stress on play behaviour and HPA axis reactivity in rats.
Acknowledgements
We would like to thank the students and research assistants who have contributed to the studies outlined in this review. We are very grateful to Dr Claude Lazure (Clinical Research Institute of Montreal, Canada) for critical reading of the manuscript. Work performed by our group was supported by grants from the French Ministry of Education and grants of the Conseil Régional du Nord-Pas de Calais.
References
- Al-Dujaili EA, Hope J, Estivariz FE, Lowry PJ, Edwards CR. Circulating human pituitary pro-gamma-melanotropin enhances the adrenal response to ACTH. Nature 1981; 291: 156–159
- Alikhani-Koopaei R, Fouladkou F, Frey FJ, Frey BM. Epigenetic regulation of 11 beta-hydroxysteroid dehydrogenase type 2 expression. J Clin Invest 2004; 114: 1146–1157
- Andrews MH, Matthews SG. Programming of the hypothalamo–pituitary–adrenal axis: Serotonergic involvement. Stress 2004; 7: 15–27
- Baid S, Nieman LK. Glucocorticoid excess and hypertension. Curr Hypertens Rep 2004; 6: 493–499
- Baram TZ, Schultz L. CRH gene expression in the fetal rat is not increased after pharmacological adrenalectomy. Neurosci Lett 1992; 142: 215–218
- Barker DJP. Mothers, babies and health in later life2nd ed. Churchill Livingstone, Edinburgh 1998
- Barker DJP, Clark PM. Fetal undernutrition and disease in later life. Rev Reprod 1997; 2: 105–112
- Beitins IZ, Bayard F, Ances IG, Kowarski A, Migeon CJ. The metabolic clearance rate, blood production, interconversion and transplacental passage of cortisol and cortisone in pregnancy near term. Pediatr Res 1973; 7: 509–519
- Belda X, Ons S, Carrasco J, Armario A. The effects of chronic food restriction on hypothalamic–pituitary–adrenal activity depend on morning versus evening availability of food. Pharmacol Biochem Behav 2005; 81: 41–46
- Bell AW, Ehrhardt RA. Regulation of placental nutrient transport and implications for fetal growth. Nutr Res Rev 2002; 15: 211–230
- Benediktsson R, Lindsay RS, Noble J, Seckl JR, Edwards CR. Glucocorticoid exposure in utero: New model for adult hypertension. Lancet 1993; 341: 339–341
- Bertram CE, Hanson MA. Prenatal programming of postnatal endocrine responses by glucocorticoids. Reproduction 2002; 124: 459–467
- Björntorp P. Insulin resistance: The consequence of neuroendocrine disturbance?. Int J Obes 1995; 19(Suppl 1)S6–S10
- Bloomfield FH, Oliver MH, Giannoulias CD, Gluckman PD, Harding JE, Challis JR. Brief undernutrition in late-gestation sheep programs the hypothalamic–pituitary–adrenal axis in adult offspring. Endocrinology 2003; 144: 2933–2940
- Brawley L, Poston L, Hanson MA. Mechanisms underlying the programming of small artery dysfunction: Review of the model using low protein diet in pregnancy in the rat. Arch Physiol Biochem 2003a; 111: 23–35
- Brawley L, Torrens C, Anthony FW, Itoh S, Wheeler T, Jackson AA, Clough GF, Poston L, Hanson MA. Glycine rectifies vascular dysfunction induced by dietary protein imbalance during pregnancy. J Physiol 2003b; 554: 497–504
- Brooks AN, Hagan DM, Howe DC. Neuroendocrine regulation of pituitary–adrenal function during fetal life. Eur J Endocrinol 1996; 135: 153–165
- Buckingham JC. Glucocorticoids: Exemplars of multi-tasking. Br J Pharmacol 2006; 147: S258–S268
- Catalani A, Casolini P, Scaccianoce S, Patacchioli FR, Spinozzi P, Angelucci L. Maternal corticosterone during lactation permanently affects brain corticosteroid receptors, stress response and behaviour in rat progeny. Neuroscience 2000; 100: 319–325
- Chen K, Li F, Li J, Cai H, Strom S, Bisello A, Kelley DE, Friedman-Einat M, Skibinski GA, McCrory MA, Szalai AJ, Zhao AZ. Induction of leptin resistance through direct interaction of C-reactive protein with leptin. Nat Med 2006; 12: 425–432
- Chisari AN, Giovambattista A, Perello M, Spinedi E. Impact of maternal undernutrition on hypothalamo–pituitary–adrenal axis and adipocyte functions in male rat offspring. Endocrine 2001; 14: 375–382
- Chrousos GP. The role of stress and the hypothalamic–pituitary–adrenal axis in the pathogenesis of the metabolic syndrome: Neuro-endocrine and target tissue-related causes. Int J Obes Relat Metab Disord 2000; 24: S50–S55
- Clark PM. Programming of the hypothalamo–pituitary–adrenal axis and the fetal origins of adult disease hypothesis. Eur J Pediatr 1998; 1: 7–10
- Clark PM, Hindmarsh PC, Shiell AW, Law CM, Honour JW, Barker DJ. Size at birth and adrenocortical function in childhood. Clin Endocrinol 1996; 45: 721–726
- Clarke AS, Wittwer DJ, Abbott DH, Schneider ML. Long-term effects of prenatal stress on HPA axis activity in juvenile rhesus monkeys. Dev Psychobiol 1994; 27: 257–269
- Clarke MC, Harley M, Cannon M. The role of obstetric events in schizophrenia. Schizophr Bull 2006; 32: 3–8
- Coates PJ, Doniach I, Wells C, Hale AC, Rees LH, Besser GM. Peptides related to alpha-melanocyte-stimulating hormone are commonly produced by human pituitary corticotroph adenomas: No relationship with pars intermedia origin. J Endocrinol 1989; 120: 531–536
- Dallman MF, Engeland WC, Rose JC, Wilkinson CW, Shinsako J, Siedenburg F. Nycthemeral rhythm in adrenal responsiveness to ACTH. Am J Physiol 1978; 235: 210–218
- Das UN. Pathophysiology of metabolic syndrome X and its links to the perinatal period. Nutrition 2005; 21: 762–773
- Diaz R, Brown RW, Seckl JR. Distinct ontogeny of glucocorticoid and mineralocorticoid receptor and 11beta-hydroxysteroid dehydrogenase types I and II mRNAs in the fetal rat brain suggest a complex control of glucocorticoid actions. J Neurosci 1998; 18: 2570–2580
- Edwards LJ, McMillen IC. Maternal undernutrition increases arterial blood pressure in the sheep fetus during late gestation. J Physiol 2001b; 533: 561–570
- Edwards LJ, McMillen IC. Impact of maternal undernutrition during the periconceptional period, fetal number, and fetal sex on the development of the hypothalamo–pituitary adrenal axis in sheep during late gestation. Biol Reprod 2002b; 66: 1562–1569
- Edwards LJ, Coulter CL, Symonds ME, McMillen IC. Prenatal undernutrition, glucocorticoids and the programming of adult hypertension. Clin Exp Pharmacol Physiol 2001a; 28: 938–941
- Edwards LJ, Bryce AE, Coulter CL, McMillen IC. Maternal undernutrition throughout pregnancy increases adrenocorticotrophin receptor and steroidogenic acute regulatory protein gene expression in the adrenal gland of twin fetal sheep during late gestation. Mol Cell Endocrinol 2002a; 196: 1–10
- FAO/WHO. Global teach in will help children understand and fight hunger and malnutrition. 2001, Rome:FAO and Geneva:WHO
- Fichter MM, Pirke KM. Effect of experimental and pathological weight loss upon the hypothalamo–pituitary–adrenal axis. Psychoneuroendocrinology 1986; 11: 295–305
- Fowden AL, Apatu RS, Silver M. The glucogenic capacity of the fetal pig: Developmental regulation by cortisol. Exp Physiol 1995; 80: 457–467
- Freemark M. Editorial: The fetal adrenal and the maturation of the growth hormone and prolactin axes. Endocrinology 1999; 140: 1963–1965
- Galler JR, Ross RN. Malnutrition and mental development. Textbook of pediatric nutrition2nd ed., RM Suskind, L Lewinter-Suskin. Raven Press, New York, NY 1993; 173–179
- Galler JR, Ricutti HN, Crawford MA, Kucharski LT. The role of mother-infant interaction in nutritional disorders. Humana malnutrition: A comprehensive treatise, JR Galler. Plenum press, New York 1984; Vol. 5: 269–304, Nutrition and Behavior
- Galler JR, Shumsky JS, Morgane PJ. Malnutrition and brain development. Nutrition in pediatrics2nd ed., WA Walker, 1997; 196–212, Chapter 14
- Gluckman PD, Hanson MA. Maternal constraint of fetal growth and its consequences. Semin Fetal Neonatal Med 2004a; 9: 419–425
- Gluckman PD, Hanson MA. Living with the past: Evolution, development and patterns of disease. Science 2004b; 305: 1733–1736
- Gluckman PD, Hanson MA, Spencer HG, Bateson P. Environmental influences during development and their later consequences for heath and disease: Implications for the interpretation of empirical studies. Proc R Soc B 2005; 272: 671–677
- Goland RS, Jozak S, Warren WB, Conwell IM, Stark RI, Tropper PJ. Elevated levels of umbilical cord plasma corticotropin-releasing hormone in growth-retarded fetuses. J Clin Endocrinol Metab 1993; 77: 1174–1179
- Gold PW, Gwirtsman H, Avgerinos PC, Nieman LK, Gallucci WT, Kaye W, Jimerson D, Ebert M, Rittmaster R, Loriaux DL, et al. Abnormal hypothalamic–pituitary–adrenal function in anorexia nervosa. Pathophysiologic mechanisms in underweight and weight-corrected patients. N Engl J Med 1986; 4: 1335–1342
- Grino M, Young WS, Burgunder JM. Ontogeny of expression of the corticotropin-releasing factor gene in the hypothalamic paraventricular nucleus and of the proopiomelanocortin gene in rat pituitary. Endocrinology 1989; 124: 60–68
- Grino M, Paulmyer-Lacroix O, Anglade G, Oliver C. Molecular aspects of the regulation of the hypothalamo–pituitary–adrenal axis during development in the rat. Ann NY Acad Sci 1995; 771: 339–351
- Haffner SM. The metabolic syndrome: Inflammation, diabetes mellitus, and cardiovascular disease. Am J Cardiol 2006; 97: 3–11
- Han ES, Evans TR, Nelson JF. Adrenocortical responsiveness to adrenocorticotropic hormone is enhanced in chronically food-restricted rats. J Nutr 1998; 128: 1415–1420
- Hawkins P, Steyn C, McGarrigle HH, Saito T, Ozaki T, Stratford LL, Noakes DE, Hanson MA. Effect of maternal nutrient restriction in early gestation on responses of the hypothalamic–pituitary–adrenal axis to acute isocapnic hypoxaemia in late gestation fetal sheep. Exp Physiol 2000a; 85: 85–96
- Hawkins P, Steyn C, McGarrigle HH, Calder NA, Saito T, Stratford LL, Noakes DE, Hansona MA. Cardiovascular and hypothalamic–pituitary–adrenal axis development in late gestation fetal sheep and young lambs following modest maternal nutrient restriction in early gestation. Reprod Fertil Dev 2000b; 12: 443–456
- Hawkins P, Hanson MA, Matthews SG. Maternal undernutrition in early gestation alters molecular regulation of the hypothalamic–pituitary–adrenal axis in the ovine fetus. J Neuroendocrinol 2001; 13: 855–861
- Henderson DC. Schizophrenia and comorbid metabolic disorders. J Clin Psychiatry 2005; 6: 11–20
- Henry C, Kabbaj M, Simon H, Le Moal M, Maccari S. Prenatal stress increases the hypothalamo–pituitary–adrenal axis response in young and adult rats. J Neuroendocrinol 1994; 6: 341–345
- Hoet JJ, Hanson MA. Intrauterine nutrition: Its importance during critical periods for cardiovascular and endocrine development. J Physiol 1999; 514: 617–627
- Holemans K, Aerts L, Van Assche FA. Fetal growth restriction and consequences for the offspring in animal models. J Soc Gynecol Investig 2003; 10: 392–399
- Hooper SB, Young IR. Endocrine maturation of the fetus. Endocrine maturation of the fetus, FW Bazer. Humana press, Totowa, NJ 1998; 353–386
- Hugin-Flores ME, Steimer T, Schulz P, Vallotton MB, Aubert ML. Chronic corticotropin-releasing hormone and vasopressin regulate corticosteroid receptors in rat hippocampus and anterior pituitary. Brain Res 2003; 976: 159–170
- Hugin-Flores ME, Steimer T, Aubert ML, Schulz P. Mineralo- and glucocorticoid receptor mrnas are differently regulated by corticosterone in the rat hippocampus and anterior pituitary. Neuroendocrinology 2004; 79: 174–184
- Jacobson L, Zurakowski D, Majzoub JA. Protein malnutrition increases plasma adrenocorticotropin and anterior pituitary proopiomelanocortin messenger ribonucleic acid in the rat. Endocrinology 1997; 138: 1048–1057
- Jaffe RB, Mesiano S, Smith R, Coulter CL, Spencer SJ, Chakravorty A. The regulation and role of fetal adrenal development in human pregnancy. Endocr Res 1998; 24: 919–926
- Jezova D, Skultetyova I, Makatsori A, Moncek F, Duncko R. Hypothalamo–pituitary–adrenocortical axis function and hedonic behavior in adult male and female rats prenatally stressed by maternal food restriction. Stress 2002; 5: 177–183
- Jones A, Godfrey KM, Wood P, Osmond C, Goulden P, Phillips DI. Fetal growth and the adrenocortical response to psychological stress. J Clin Endocrinol Metab 2006; 91: 1868–1871
- Kajantie E, Eriksson J, Barker DJ, Forsen T, Osmond C, Wood PJ, Andersson S, Dunkel L, Phillips DI. Birthsize, gestational age and adrenal function in adult life: Studies of dexamethasone suppression and ACTH1-24 stimulation. Eur J Endocrinol 2003; 149: 569–575
- Kajantie E, Eriksson J, Osmond C, Wood PJ, Forsen T, Barker DJ, Phillips DI. Size at birth, the metabolic syndrome and 24-h salivary cortisol profile. Clin Endocrinol 2004; 60: 201–207
- Kapoor A, Matthews SG. Short periods of prenatal stress affect growth, behaviour and hypothalamo–pituitary–adrenal axis activity in male guinea pig offspring. J Physiol 2005; 566: 967–977
- Karrow NA. Activation of the hypothalamic–pituitary–adrenal axis and autonomic nervous system during inflammation and altered programming of the neuroendocrine-immune axis during fetal and neonatal development: Lessons learned from the model inflammagen, lipopolysaccharide. Brain Behav Immun 2006; 20: 144–158
- Kawahata A, Sakamoto H. Some observations on sweating of the Aino. Jpn J Physiol 1951; 2: 166–169
- King JC. The risk of maternal nutritional depletion and poor outcomes increases in early or closely spaced pregnancies. J Nutr 2003; 133: 1732–1736
- Kippin TE, Cain SW, Masum Z, Ralph MR. Neural stem cells show bidirectional experience-dependent plasticity in the perinatal mammalian brain. J Neurosci 2004; 24: 2832–2836
- Koehl M, Darnaudery M, Dulluc J, Van Reeth O, Le Moal M, Maccari S. Prenatal stress alters circadian activity of hypothalamo–pituitary–adrenal axis and hippocampal corticosteroid receptors in adult rats of both gender. J Neurobiol 1999; 40: 302–315
- Koh KK, Han SH, Quon MJ. Inflammatory markers and the metabolic syndrome: Insights from therapeutic interventions. J Am Coll Cardiol 2005; 46: 1978–1985
- Kumarasamy V, Mitchell MD, Bloomfield FH, Oliver MH, Campbell ME, Challis JR, Harding JE. Effects of periconceptional undernutrition on the initiation of parturition in sheep. Am J Physiol 2005; 288: 67–72
- Langley-Evans SC, Phillips GJ, Benediktsson R, Gardner DS, Edwards CR, Jackson AA, Seckl JR. Protein intake in pregnancy, placental glucocorticoid metabolism and the programming of hypertension in the rat. Placenta 1996; 17: 169–172
- Langley-Evans SC. Hypertension induced by fœtal exposure to a maternal low-protein diet, in the rat, is prevented by pharmacological blockade of maternal glucocorticoid synthesis. J Hypertens 1997; 15: 537–544
- Leonhardt M, Lesage J, Dufourny L, Dickes-Coopman A, Montel V, Dupouy JP. Perinatal maternal food restriction induces alterations in hypothalamo–pituitary–adrenal axis activity and in plasma corticosterone-binding globulin capacity of weaning rat pups. Neuroendocrinology 2002; 75: 45–54
- Lesage J, Blondeau B, Grino M, Breant B, Dupouy JP. Maternal undernutrition during late gestation induces fetal overexposure to glucocorticoids and intrauterine growth retardation, and disturbs the hypothalamo–pituitary adrenal axis in the newborn rat. Endocrinology 2001; 142: 1692–1702
- Lesage J, Dufourny L, Laborie C, Bernet F, Blondeau B, Avril I, Breant B, Dupouy JP. Perinatal malnutrition programs sympathoadrenal and hypothalamic–pituitary–adrenal axis responsiveness to restraint stress in adult male rats. J Neuroendocrinol 2002a; 14: 135–143
- Lesage J, Hahn D, Leonhardt M, Blondeau B, Breant B, Dupouy JP. Maternal undernutrition during late gestation-induced intrauterine growth restriction in the rat is associated with impaired placental GLUT3 expression, but does not correlate with endogenous corticosterone levels. J Endocrinol 2002b; 174: 37–43
- Lesage J, Del-Favero F, Leonhardt M, Louvart H, Maccari S, Vieau D, Darnaudery M. Prenatal stress induces intrauterine growth restriction and programmes glucose intolerance and feeding behaviour disturbances in the aged rat. J Endocrinol 2004; 181: 291–296
- Levine S. Maternal and environmental influences on the adrenocortical response to stress in weanling rats. Science 1957; 156: 258–260
- Levitt NS, Lindsay RS, Holmes MC, Seckl JR. Dexamethasone in the last week of pregnancy attenuates hippocampal glucocorticoid gene expression and elevates blood pressure in the adult offspring in the rat. Neuroendocrinology 1996; 64: 412–418
- Levitt NS, Lambert EV, Woods D, Hales CN, Andrew R, Seckl JR. Impaired glucose tolerance and elevated blood pressure in low birth weight, nonobese, young south african adults: Early programming of cortisol axis. J Clin Endocrinol Metab 2000; 85: 4611–4618
- Li J, Owens JA, Owens PC, Saunders JC, Fowden AL, Gilmour RS. The ontogeny of hepatic growth hormone receptor and insulin-like growth factor I gene expression in the sheep fetus during late gestation: Developmental regulation by cortisol. Endocrinology 1996; 137: 1650–1657
- Lindsay RS, Lindsay RM, Waddell BJ, Seckl JR. Prenatal glucocorticoid exposure leads to offspring hyperglycaemia in the rat: Studies with the 11 beta-hydroxysteroid dehydrogenase inhibitor carbenoxolone. Diabetologia 1996a; 39: 1299–1305
- Lindsay RS, Lindsay RM, Edwards CR, Seckl JR. Inhibition of 11-beta-hydroxysteroid dehydrogenase in pregnant rats and the programming of blood pressure in the offspring. Hypertension 1996b; 27: 1200–1204
- Lingas RI, Matthews SG. A short period of maternal nutrient restriction in late gestation modifies pituitary–adrenal function in adult guinea pig offspring. Neuroendocrinology 2001; 73: 302–311
- Lingas R, Dean F, Matthews SG. Maternal nutrient restriction (48 hours), modifies brain corticosteroid receptors expression and endocrine function in the fetal guinea pig. Brain Res 1999; 846: 236–242
- Liu L, Li A, Matthews SG. Maternal glucocorticoid treatment programs HPA regulation in adult offspring: Sex specific effects. Am J Physiol Endocrinol Metab 2001; 280: 729–739
- Lloyd-Jones DM, Liu K, Tian L, Greenland P. Narrative review: Assessment of C-reactive protein in risk prediction for cardiovascular disease. Ann Intern Med 2006; 145: 35–42
- Loirdighi N, Menard D, Delvin D, Levy E. Selective effects of hydro-cortisone on intestinal lipoprotein and apolipoprotein synthesis in the human fetus. J Cell Biochem 1997; 66: 65–76
- Maccari S, Darnaudery M, Morley-Fletcher S, Zuena AR, Cinque C, Van Reeth O. Prenatal stress and long-term consequences: Implications of glucocorticoid hormones. Neurosci Biobehav Rev 2003; 27: 119–122
- Matthews SG. Dynamic changes in glucocorticoid and mineralocorticoid receptor mRNA in the developing guinea pig brain. Dev Brain Res 1998; 107: 123–132
- Matthews SG. Early programming of the hypothalamo–pituitary–adrenal axis. Trends Endocrinol Metab 2002; 13: 373–380
- McCormick CM, Smythe JW, Sharma S, Meaney MJ. Sex-specific effects of prenatal stress on hypothalamic–pituitary–adrenal responses to stress and brain glucocorticoid receptor density in adult rats. Brain Res Dev Brain Res 1995; 84: 55–61
- McMillen IC, Schwartz J, Coulter CL, Edwards LJ. Early embryonic environment, the fetal pituitary–adrenal axis and the timing of parturition. Endocr Res 2004; 30: 845–850
- McMinn J, Wei M, Schupf N, Cusmai J, Johnson EB, Smith AC, Weksberg R, Thaker HM, Tycko B. Unbalanced placental expression of imprinted genes in human intrauterine growth restriction. Placenta 2005; 27: 540–549
- Meyer J, Koro CE, L'Italien GJ. The metabolic syndrome and schizophrenia: A review. Int Rev Psychiatry 2005; 17: 173–180
- Morley-Fletcher S, Rea M, Maccari S, Laviola G. Environmental enrichment during adolescence reverses the effects of prenatal stress on play behaviour and HPA axis reactivity in rats. Eur J Neurosci 2003; 18: 3367–3374
- Murphy BE, Clark SJ, Donald IR, Pinsky M, Vedady D. Conversion of maternal cortisol to cortisone during placental transfer to the human fetus. Am J Obstet Gynecol 1974; 118: 538–541
- Myatt L. Placental adaptive responses and fetal programming. J Physiol 2006; 572: 25–30
- Newell-Price J. Proopiomelanocortin gene expression and DNA methylation: Implications for Cushing's syndrome and beyond. J Endocrinol 2003; 177: 365–372
- Noel G, Mains RE. Plasticity of peptide biosynthesis in corticotropes: Independent regulation of different steps in processing. Endocrinology 1991; 129: 1317–1325
- Noyan-Ashraf MH, Wu L, Wang R, Juurlink BH. Dietary approaches to positively influence fetal determinants of adult health. FASEB J 2006; 20: 371–373
- Nyirenda MJ, Lindsay RS, Kenyon CJ, Burchell A, Seckl JR. Glucocorticoid exposure in late gestation permanently programs rat hepatic phosphoenolpyruvate carboxykinase and glucocorticoid receptor expression and causes glucose intolerance in adult offspring. J Clin Invest 1998; 101: 2174–2181
- O'Regan D, Welberg LL, Holmes MC, Seckl JR. Glucocorticoid programming of pituitary–adrenal function: Mechanisms and physiological consequences. Semin Neonatol 2001; 6: 319–329
- Ogilvie KM, Rivier C. Prenatal alcohol exposure results in hyperactivity of the hypothalamic–pituitary–adrenal axis of the offspring: Modulation by fostering at birth and postnatal handling. Alcohol Clin Exp Res 1997; 21: 424–429
- Oliver C, Boudouresque F, Lacroix O, Anglade G, Grino M. Effect of POMC-derived peptides on corticosterone secretion during the stress hypo-responsive period in rat. Endocr Regul 1994; 28: 67–72
- Ordyan NE, Pivina SG, Rakitskaya VV, Shalyapina VG. The neonatal glucocorticoid treatment-produced long-term changes of the pituitary–adrenal function and brain corticosteroid receptors in rats. Steroids 2001; 66: 883–888
- Pedersen RC, Brownie AC, Ling N. Pro-adrenocorticotropin/endorphin-derived peptides: Coordinate action on adrenal steroidogenesis. Science 1980; 208: 1044–1046
- Phillips DI. Fetal growth and programming of the hypothalamo–pituitary–adrenal axis. Clin Exp Pharmacol Physiol 2001; 28: 967–970
- Phillips DI, Jones A. Fetal programming of autonomic and HPA function: Do people who were small babies have enhanced stress responses?. J Physiol 2006, (in press)
- Phillips DI, Barker DJ, Fall CH, Seckl JR, Whorwood CB, Wood PJ, Walker BR. Elevated plasma cortisol concentrations: A link between low birth weight and the insulin resistance syndrome?. J Clin Endocrinol Metab 1998; 83: 757–760
- Phillips DI, Walker BR, Reynolds RM, Flanagan DE, Wood PJ, Osmond C, Barker DJ, Whorwood CB. Low birth weight predicts elevated plasma cortisol concentrations in adults from 3 populations. Hypertension 2000; 35: 1301–1306
- Phillips ID, Simonetta G, Owens JA, Robinson JS, Clarke IJ, McMillen IC. Placental restriction alters the functional development of the pituitary–adrenal axis in the sheep fetus during late gestation. Pediatr Res 1996; 40: 861–866
- Phillips ID, Anthony RV, Houghton DC, McMillen IC. The regulation of prolactin receptor messenger ribonucleic acid levels in the sheep liver before birth: Relative roles of the fetal hypothalamus, cortisol, and the external photoperiod. Endocrinology 1999; 140: 1966–1971
- Rassin DK. Protein nutrition in the neonate: Assessment and implications for brain development. Current topics in nutrition disease, DK Rassin, 1987; Vol. 16: 19–39
- Reinisch JM, Simon NG, Karow WG, Gandelman R. Prenatal exposure to prednisone in humans and animals retards intrauterine growth. Science 1978; 202: 436–438
- Resko JA, Roselli CE. Prenatal hormones organize sex differences of the neuroendocrine reproductive system: Observations on guinea pigs and nonhuman primates. Cell Mol Neurobiol 1997; 17: 627–648
- Reul JM, Stec I, Wiegers GJ, Labeur MS, Linthorst AC, Arzt E, Holsboer F. Prenatal immune challenge alters the hypothalamic–pituitary–adrenocortical axis in adult rats. J Clin Invest 1994; 93: 2600–2607
- Reynolds RM, Walker BR, Syddall HE, Andrew R, Wood PJ, Whorwood CB, Phillips DI. Altered control of cortisol secretion in adult men with low birth weight and cardiovascular risk factors. J Clin Endocrinol Metab 2001; 86: 245–250
- Rivière G, Michaud A, Breton C, VanCamp G, Laborie C, Enache M, Lesage J, Deloof S, Corvol P, Vieau D. Angiotensin-converting enzyme 2 (ACE2), and ACE activities display tissue-specific sensitivity to undernutrition-programmed hypertension in the adult rat. Hypertension 2005; 46: 1169–1174
- Sacerdote A, Weiss K, Tran T, Rokeya Noor B, McFarlane SI. Hypertension in patients with Cushing's disease: Pathophysiology, diagnosis, and management. Curr Hypertens Rep 2005; 7: 212–218
- Salehi M, Ferenczi A, Zumoff B. Obesity and cortisol status. Horm Metab Res 2005; 37: 193–197
- Sapolsky RM, Krey LC, McEwen BS. The neuroendocrinology of stress and aging; the glucocorticoid cascade. Endocr Rev 1986; 7: 284–301
- Scaccianoce S, Di Sciullo A, Angelucci L. Age-related changes in hypothalamo–pituitary–adrenal axis activity in the rat: In vitro studies. Neuroendocrinology 1990; 52: 150–155
- Sebaai N, Lesage J, Vieau D, Alaoui A, Dupouy JP, Deloof S. Altered control of the hypothalamo–pituitary–adrenal axis in adult male rats exposed perinatally to food deprivation and/or dehydration. Neuroendocrinology 2002; 76: 243–253
- Sebaai N, Lesage J, Breton C, Vieau D, Deloof S. Perinatal food deprivation induces marked alterations of the hypothalamo–pituitary–adrenal axis in 8-month old male rats both under basal conditions and after a dehydration period. Neuroendocrinology 2004; 79: 163–173
- Seckl JR. 11 beta-hydroxysteroid dehydrogenase isoforms and their implications for blood pressure regulation. Eur J Clin Invest 1993; 23: 589–601
- Seckl JR. Glucocorticoids, feto-placental 11 beta-hydroxysteroid dehydrogenase type 2, and the early life origins of adult disease. Steroids 1997; 62: 89–94
- Seckl JR, Meaney MJ. Glucocorticoid programming. Ann N Y Acad Sci 2004; 1032: 63–84
- Shanks N, Harbuz MS, Jessop DS, Perks P, Moore PM, Lightman SL. Inflammatory disease as chronic stress. Ann N Y Acad Sci 1998; 840: 599–607
- Stewart J, Meaney MJ, Aitken D, Jensen L, Kalant N. The effects of acute and life-long food restriction on basal and stress-induced serum corticosterone levels in young and aged rats. Endocrinology 1988; 123: 1934–1941
- Stewart PM, Rogerson FM, Mason JI. Type 2 11 beta-hydroxysteroid dehydrogenase messenger ribonucleic acid and activity in human placenta and fetal membranes: Its relationship to birth weight and putative role in fetal adrenal steroidogenesis. J Clin Endocrinol Metab 1995; 80: 885–890
- Summers SA, Nelson DH. A role for sphingolipids in producing the common features of type 2 diabetes, metabolic syndrome, and Cushing's syndrome. Diabetes 2005; 54: 591–602
- Szitanyi P, Janda J, Poledne R. Intrauterine undernutrition and programming as a new risk of cardiovas cular disease in later life. Physiol Rev 2003; 52: 389–395
- Ugrumov MV, Ivanova IP, Mitskevich MS, Liposits Z, Setalo G, Flerko B. Axovascular relationships in developing median eminence of perinatal rats with special reference to luteinizing hormone-releasing hormone projections. Neuroscience 1985; 16: 897–906
- Vallee M, Maccari S, Dellu F, Le Moal M, Simon H, Mayo W. Long term effects of prenatal stress and postnatal handling on age-related glucocorticoid secretion and cognitive performance. A longitudinal study in the rat. Eur J Neurosci 1999; 11: 2906–2916
- Vickers MH, Breier BH, Cutfield WS, Hofman PL, Gluckman PD. Fetal origins of hyperphagia, obesity and hypertension and postnatal amplification by hypercaloric nutrition. Am J Physiol Endocrinol Metab 2000; 279: 83–87
- Ward AM, Syddall HE, Wood PJ, Chrousos GP, Phillips DI. Fetal programming of the hypothalamic–pituitary–adrenal (HPA), axis: Low birth weight and central HPA regulation. J Clin Endocrinol Metab 2004; 89: 1227–1233
- Weaver IC, Cervoni N, Champagne FA, D'Alessio AC, Sharma S, Seckl JR, Dymov S, Szyf M, Meaney MJ. Epigenetic programming by maternal behavior. Nat Neurosci 2004; 7: 847–854
- Weinstock M, Matlina E, Maor GI, Rosen H, McEwen BS. Prenatal stress selectively alters the reactivity of the hypothalamic–pituitary–adrenal system in the female rat. Brain Res 1992; 595: 195–200
- Whorwood CB, Firth KM, Budge H, Symonds ME. Maternal undernutrition during early to midgestation programs tissue-specific alterations in the expression of the glucocorticoid receptor, 11beta-hydroxysteroid dehydrogenase isoforms, and type 1 angiotensin 2 receptor in neonatal sheep. Endocrinology 2001; 142: 2854–2864
- Wisse BE. The inflammatory syndrome: The role of adipose tissue cytokines in metabolic disorders linked to obesity. J Am Soc Nephrol 2004; 15: 2792–2800
- Woods LL, Weeeks DA. Prenatal programming of adult blood pressure: Role of maternal corticosteroids. Am J Physiol Regul Integr Comp Physiol 2005; 289: 955–962
- Workel JO, Oitzl MS, Fluttert M, Lesscher H, Karssen A, de Kloet ER. Differential and age-dependent effects of maternal deprivation on the hypothalamo–pituitary–adrenal axis of brown Norway rats from youth to senescence. J Neuroendocrinol 2001; 13: 569–580
- Wu G, Bazer FW, Cudd TA, Meininger CJ, Spencer TE. Maternal nutrition and fetal development. J Nutr 2004; 134: 2169–2172
- Wust S, Entringer S, Federenko IS, Schlotz W, Hellhammer DH. Birth weight is associated with salivary cortisol responses to psychosocial stress in adult life. Psychoneuroendocrinology 2005; 30: 591–598
- Wyrwoll CS, Mark PJ, Mori TA, Puddey IB, Waddell BJ. Prevention of programmed hyperleptinemia and hypertension by postnatal dietary omega-3 fatty acids. Endocrinology 2006; 147: 599–606
- Zhang X, Sliwowska JH, Weinberg J. Prenatal alcohol exposure and fetal programming: Effects on neuroendocrine and immune function. Exp Biol Med 2005; 230: 376–388