Abstract
Calcium (Ca2+ ) plays an essential role in lymphocyte activation and maturation. Acute and chronic stress has been shown to modulate the lymphocyte immune response; but the relationship between cytosolic free Ca2+ concentration ([Ca2+ ]i) and the immune response in lymphocytes following exposure to stress has not been examined. In the present study, we investigated the effects of acute restraint stress on [Ca2+ ]i and the proliferation of splenic lymphocytes from mice. We observed that 2 h of restraint significantly increased plasma corticosterone levels in mice. On examining [Ca2+ ]i and the proliferation ex vivo of splenic lymphocytes isolated from restraint-stressed mice using fura-2 and 3-(4,5-dimethyl-2-thiazolyl)-2,5-diphenyl-2H-tetrazolium bromide, respectively, we found that acute restraint stress caused a significant increase in resting [Ca2+ ]i and significantly enhanced the ability of concanavalin A (Con A; a T-cell-selective mitogen) to increase [Ca2+ ]i but not that of lipopolysaccharide (LPS; a B-cell-selective mitogen). In addition, acute restraint stress significantly enhanced Con A-stimulated but not LPS-stimulated lymphocyte proliferation. Overall, there was a positive correlation between [Ca2+ ]i and T-cell proliferation following acute restraint stress. The enhancements of [Ca2+ ]i and T-cell proliferation were completely suppressed by verapamil (a Ca2+ channel blocker). These results suggest that acute restraint stress enhances Con A-stimulated T-cell proliferation by increasing [Ca2+ ]i via stimulation of Ca2+ entry.
Introduction
Stress has long been known to alter the behavior and homeostatic state of animals including endocrine and immunological functions. The impact of stress exposure on the development of an immune response depends on a variety of factors, such as the duration and the type of the stressor (McEwen Citation1998). For example, acute stress has been reported to enhance immune function, whereas chronic stress has been shown to suppress it (Millan et al. Citation1996; Dhabhar and McEwen Citation1997; Dhabhar and McEwen Citation1999).
Several studies in laboratory animals have revealed that stress causes changes in cellular and humoral immunity. Exposure of rodents to acute stress increases mitogen-induced T-cell proliferation (Lysle et al. Citation1990; Bauer et al. Citation2001), the T-cell-dependent delayed-type hypersensitivity response (Dhabhar and McEwen Citation1996), and both T-cell-dependent and -independent antibody production (Okimura et al. Citation1986; Millan et al. Citation1996). Other authors, however, have reported a decrease in the response to T-cell-dependent antigens following acute stress (footshock), depending on its severity and the amount of time between immunization and application of the stress (Laudenslager et al. Citation1988; Zalcman et al. Citation1988; Zalcman and Anisman Citation1993; Fleshner et al. Citation1996; Millan et al. Citation1996). On the other hand, chronic stress exposure disrupts cellular immunity (Kusnecov and Rabin Citation1993; Dhabhar and McEwen Citation1997), reduces mitogen-induced T-cell proliferation (Silberman et al. Citation2002), causes a poor T-cell-dependent antibody response (Fukui et al. Citation1997; Tournier et al. Citation2001), and disrupts cytokine secretion (Zhang et al. Citation1998). However, the mechanisms by which these alterations occur have not been fully elucidated.
The spleen is primarily involved in dealing with blood-borne pathogens, such as encapsulated bacteria (Mond et al. Citation1995). The spleen cell population is heterogeneous, consisting mostly of lymphocytes, i.e. T- and B-cells (Arora et al. Citation1981), which play an important role in cellular and humoral immunity, respectively.
Calcium (Ca2+ ) plays an essential role in lymphocyte activation and maturation. Cross-linking of antigen or Fc receptors on T- and B-cells rapidly increases the cytosolic free Ca2+ concentration ([Ca2+ ]i) (Braun et al. Citation1979; van Leeuwen and Samelson Citation1999), which activates many signaling factors, including nuclear factor of activated T cells (NFAT), nuclear factor-kB (NF-kB) and c-Jun NH2-terminal kinase-1 (JNK1) (Dolmetsch et al. Citation1997; Feske et al. Citation2001). These factors, in turn, regulate the expression of several inducible genes that mediate diverse genetic programs, including effector immune function and cell proliferation, differentiation and death (Teague et al. Citation1999).
In aged mice, both resting and mitogen-stimulated [Ca2+ ]i are significantly lower in T-cells from those exposed to chronic overcrowding stress than in T-cells from those not subjected to such stress (Csermely et al. Citation1995). Sei et al. (Citation1991) observed that acute restraint stress suppresses mitogen-stimulated Ca2+ mobilization in CD4+ T-cells but enhances Ca2+ mobilization in CD8+ T-cells. They also noted that chronic restraint stress suppresses mitogen-stimulated Ca2+ mobilization in CD4+ T-cells and B-cells (Sei et al. Citation1991). Since immunofluorescence was the method used in this research to distinguish patterns of Ca2+ mobilization within a heterogeneous cell population (i.e. labeled with various monoclonal antibodies), accurate estimations of [Ca2+ ]i were not performed for pure mouse splenic lymphocytes, and to our knowledge, the effect of acute stress on [Ca2+ ]i in lymphocytes has not yet been investigated, nor has the relationship between [Ca2+ ]i and the immune response in lymphocytes after acute stress exposure. Therefore, in the present study, we investigated the effects of acute restraint stress on resting and mitogen-stimulated [Ca2+ ]i and proliferation of splenic lymphocytes from mice.
Materials and methods
Chemicals
Concanavalin A (Con A), Tris (hydroxymethyl) aminomethane and corticosterone were purchased from Wako Pure Chemicals (Osaka, Japan). Fura-2-AM and HEPES were supplied by Dojindo Laboratories (Kumamoto, Japan) and lipopolysaccharide (LPS), verapamil hydrochloride and 3-(4,5-dimethyl-2-thiazolyl)-2,5-diphenyl-2H-tetrazolium bromide (MTT) by Sigma (St Louis, MO, USA). Dulbecco's modified eagle medium (DMEM) was supplied by Invitrogen (Carlsbad, CA, USA) and fetal bovine serum by Cambrex Bio Science (Walkersville, MD, USA). Other chemicals were of reagent grade or the highest quality available.
Media
The standard incubation medium for the measurement of [Ca2+ ]i contained the following (in mM): NaCl 125, KCl 5, NaH2PO4 1.2, MgCl2 1.2, NaHCO3 5, glucose 6, CaCl2 1 and HEPES 25, with the pH adjusted to 7.4. DMEM containing 12 mM HEPES, 100 U/ml penicillin G potassium and 100 μg/ml streptomycin sulfate was used for cell culture.
Animals
Male ddY strain mice (6 weeks of age) were purchased from Japan SLC (Shizuoka, Japan). Animals were housed in standard light conditions (lights on from 6:00 am to 6:00 pm) and temperature (22 ± 2°C). Food and water were provided ad libitum. Mice were killed by decapitation after experiment. All procedures pertaining to the care and use of experimental animals were approved by the Animal Research Committee, Obihiro University, and conducted in accordance with the Declaration of Helsinki and/or the Guide for the Care and Use of Laboratory Animals as adopted and promulgated by the United States National Institutes of Health.
Stress protocol
Mice (13 weeks of age) were randomly assigned to one of two experimental groups: nonstressed group and acute restraint-stressed group. Mice were exposed to acute restraint stress as described by Sei et al. (Citation1991) and Silberman et al. (Citation2003), which involved placing them in a well-ventilated polypropylene tube (40 mm diameter × 90 mm length) for 2 h starting at 10:00 am. The mice were kept in their home cages for 15 min following the stress and then sacrificed. The nonstressed control animals were left undisturbed in their home cages prior to sacrifice. Those exposed to restraint stress were not physically compressed and did not experience pain.
Corticosterone determination
To avoid fluctuations in plasma corticosterone levels due to circadian rhythms, mice were all decapitated at 12:15 pm on the day of sacrifice. Trunk blood was collected in heparinized tubes, centrifuged and separated plasma frozen at − 80°C until analysis. Total plasma corticosterone concentrations were measured as described by Glick et al. (Citation1964). Briefly, 900 μl of isooctane was added to 200 μl of each plasma sample with thorough mixing. After centrifugation at 300g for 5 min, the top isooctane later was discarded. Following the addition of 900 μl of chloroform, each sample was mixed well and centrifuged at 300g for 5 min. The top aqueous layer was discarded, 800 μl of the chloroform was removed and the sample was added to 320 μl of acid-alcohol solution (65% H2SO4 and 35% ethanol), and this was mixed well. After centrifugation at 300g for 5 min, the bottom acid layer from each sample was transferred to a cuvette. The intensity of fluorescence of the samples was measured using a model FP-770 spectrophotofluorometer (Jasco, Hachioji, Tokyo, Japan) with excitation at 350 nm and measurement of emission at 520 nm. Corticosterone concentrations were calculated from a standard curve and expressed in ng/ml. All samples were measured in a single assay and intra-assay coefficient of variation was 7%.
Cell suspensions
Spleens were isolated from the control and stressed mice after decapitation for corticosterone measurement and passed through a 1-mm metal mesh. The spleen cells were suspended in standard medium or DMEM and then filtered through a 10-μm nylon mesh. Next, the cells were treated with pH 7.65 buffer (17 mM Tris–HCl and 0.83% ammonium chloride) to lyse erythrocytes, after which the cells were washed three times in standard medium or DMEM. For [Ca2+ ]i measurement or further cell culture, the cells were resuspended in standard medium or DMEM supplemented with 10% heat-inactivated fetal calf serum, respectively. Cell viability was greater than 80% as determined by the trypan blue exclusion test, which is consistent with that for human lymphocytes as reported by Falciola et al. (Citation1994).
[Ca2+ ]i determination
Intracellular [Ca2+ ] concentrations were measured by monitoring the intensity of fura-2 fluorescence as described previously (Satoh et al. Citation2003). Briefly, a spleen cell suspension (107 cells/ml) was incubated for 30 min at 37°C with 5 μM fura-2-AM dissolved in dimethyl sulfoxide. The cells were washed three times and then resuspended in 5 ml of standard medium. Aliquots (0.5 ml) of the cell suspension were placed in cuvettes and incubated for 10 min before addition of mitogen. Measurement of fluorescence was initiated 4 min before addition of mitogen and continued for 8 min thereafter. Samples in the cuvette were maintained at 37°C and mixed with a magnetic stirrer. Fura-2 fluorescence was measured with a model CAF-100 spectrofluorometer (Jasco) using the ratio mode. Excitation wavelengths were 340 and 380 nm, and the emission wavelength was 500 nm. [Ca2+ ]i was calculated according to the method of Grynkiewicz et al. (Citation1985).
Proliferation assay
Proliferation was determined in 24-well plates containing 1-ml aliquots of spleen cells (4 × 106 cells per well) in DMEM supplemented with 10% heat-inactivated fetal calf serum. Wells were adjusted to 1, 2, or 4 μg/ml of Con A or 5, 10, or 25 μg/ml of LPS by adding 0.5 ml of the respective test solution. Control cultures received 0.5 ml of culture medium without Con A or LPS. The cells were then cultured for 42 h at 37°C in an atmosphere containing 5% CO2. Mitogenic activity was measured by mitochondrial conversion of MTT detected at 570 nm as described by Mosmann (Citation1983) using a model Ubest-35 spectrophotometer (Jasco).
Statistical analysis
All data are expressed as the means ± SEM. Student's t-test for unpaired values was used to determine the level of significance for normally distributed data. Mitogen-stimulated [Ca2+ ]i and proliferation data were analyzed by two-way analysis of variance, using a repeated measures design. The main factors were “group” (control and acute restraint-stressed mice) and “mitogen concentration”. Tukey's HSD test was used for post-hoc analysis. Differences between means were considered significant at p < 0.05.
Results
Plasma corticosterone concentration
We measured plasma corticosterone concentrations under basal conditions and after acute restraint stress, which significantly increased corticosterone level. The plasma corticosterone concentrations in nonstressed and stressed mice were 217 ± 23 and 320 ± 25 ng/ml, respectively (t(1, 10) = 3.015, p < 0.05). The corticosterone level in the nonstressed control group was consistent with values reported by Silberman et al. (Citation2002, Citation2003, Citation2004) and Stark et al. (Citation2001).
Resting [Ca2+ ]i
We also measured resting [Ca2+ ]i in the mouse splenic lymphocytes under basal conditions and after exposure to acute restraint stress, which produced a significant increase in resting [Ca2+ ]i. The resting [Ca2+ ]i levels in nonstressed and stressed mice were 75 ± 2.6 and 87 ± 3.6 nM, respectively (t(1, 23) = 2.713, p < 0.05). The resting level in the nonstressed control group was consistent with that reported by Grinstein and Dixon (Citation1989).
Mitogen-stimulated [Ca2+ ]i
Next, we examined the changes in [Ca2+ ]i induced by T- or B-cell selective mitogens in splenic lymphocytes from nonstressed and acute restraint-stressed mice. Treatment with either mitogen caused a gradual increase in [Ca2+ ]i that plateaued within 8 min ((a) and (a)). Con A (1–4 μg/ml), the T-cell selective mitogen, caused a dose-dependent increase in [Ca2+ ]i in the splenic lymphocytes (F(2, 39) = 138.81, p < 0.0001, (b)). This increase in [Ca2+ ]i was enhanced significantly by exposure of the mice to acute restraint stress (F(1, 39) = 36.41, p < 0.0001, (a),(b)). Enhancement was significant at 1 μM (p < 0.01), 2 μM (p < 0.01) and 4 μM (p < 0.05) of Con A ((b)). However, no significant interaction between group and mitogen concentration was observed. Pretreatment of splenic lymphocytes with verapamil (a Ca2+ channel blocker; 100 μM) abolished Con A-induced increases in [Ca2+ ]i for both nonstressed and stressed mice ((b)). The inhibitory effect of verapamil in nonstressed mice agreed with the finding for human T lymphocytes reported by Nakabayashi et al. (Citation1992).
Figure 1 (a) Time course of changes in [Ca2 + ]i in response to Con A (2 μg/ml) in splenic lymphocytes from control and acute restraint-stressed mice; (b) effects of Con A and Con A plus verapamil on [Ca2 + ]i in splenic lymphocytes from control (n = 7 per group) and acute restraint-stressed (n = 9 per group) mice. Fura-2-loaded lymphocytes were incubated for 8 min with or without Con A. Verapamil was added 10 min before exposure to Con A. The net Δ[Ca2 + ]i is the difference in the increase in [Ca2 + ]i 8 min after addition of Con A or Con A plus verapamil, vs. the vehicle. Data are presented as group means ± SEM. Statistically significant differences from control are indicated as: *P < 0.05, **P < 0.01.
![Figure 1 (a) Time course of changes in [Ca2 + ]i in response to Con A (2 μg/ml) in splenic lymphocytes from control and acute restraint-stressed mice; (b) effects of Con A and Con A plus verapamil on [Ca2 + ]i in splenic lymphocytes from control (n = 7 per group) and acute restraint-stressed (n = 9 per group) mice. Fura-2-loaded lymphocytes were incubated for 8 min with or without Con A. Verapamil was added 10 min before exposure to Con A. The net Δ[Ca2 + ]i is the difference in the increase in [Ca2 + ]i 8 min after addition of Con A or Con A plus verapamil, vs. the vehicle. Data are presented as group means ± SEM. Statistically significant differences from control are indicated as: *P < 0.05, **P < 0.01.](/cms/asset/11e99651-8e54-4750-a62c-c5562dbc7367/ists_a_209523_f0001_b.gif)
Figure 2 (a) Time course of changes in [Ca2 + ]i in response to LPS (25 μg/ml) in splenic lymphocytes from control and acute restraint-stressed mice; (b) effect of LPS on [Ca2 + ]i in splenic lymphocytes from control (n = 6 per group) and acute restraint-stressed (n = 7 per group) mice. Fura-2-loaded lymphocytes were incubated for 8 min with or without LPS. The net Δ[Ca2 + ]i is the difference in the increase in [Ca2 + ]i 8 min after addition of LPS vs. vehicle. Data are presented as group means ± SEM.
![Figure 2 (a) Time course of changes in [Ca2 + ]i in response to LPS (25 μg/ml) in splenic lymphocytes from control and acute restraint-stressed mice; (b) effect of LPS on [Ca2 + ]i in splenic lymphocytes from control (n = 6 per group) and acute restraint-stressed (n = 7 per group) mice. Fura-2-loaded lymphocytes were incubated for 8 min with or without LPS. The net Δ[Ca2 + ]i is the difference in the increase in [Ca2 + ]i 8 min after addition of LPS vs. vehicle. Data are presented as group means ± SEM.](/cms/asset/4c112b5a-8f83-4e90-a11a-2760eb702917/ists_a_209523_f0002_b.gif)
LPS (5–25 μg/ml), the B-cell selective mitogen, also caused a dose-dependent increase in [Ca2+ ]i in lymphocytes from nonstressed and acute restraint-stressed mice (F(2, 32) = 24.17, p < 0.0001, (b)), but exposure to acute restraint stress failed to enhance the LPS-induced increase in [Ca2+ ]i ((a),(b)). Moreover, no significant interaction between group and mitogen concentration was observed.
Mitogen-stimulated proliferation
We further examined the proliferative responses to T-cell- or B-cell-selective mitogens in splenic lymphocytes derived from nonstressed and acute restraint-stressed mice. Con A (1–4 μg/ml) caused a dose-dependent increase in the proliferation of lymphocytes from nonstressed and acute restraint-stressed mice (F(3, 48) = 22.35, p < 0.0001, ). The increases in lymphocyte proliferation were enhanced significantly by exposure of mice to acute restraint stress (F(1, 48) = 15.72, p < 0.001, ). Enhancement was significant at 1 μM (p < 0.05), 2 μM (p < 0.01) and 4 μM (p < 0.01) of Con A (). However, no significant interaction between group and mitogen concentration was observed. Pretreatment of splenic lymphocytes with verapamil (100 μM) completely suppressed Con A-stimulated lymphocyte proliferation in nonstressed and stressed mice (). The inhibitory effect of verapamil in nonstressed mice agreed with the findings for human T lymphocytes reported by Birx et al. (Citation1984) and Nakabayashi et al. (Citation1992). At the concentration used in the present study, it had no effect on cell viability as assessed by the trypan blue exclusion test (data not shown).
Figure 3 Proliferative effects of Con A and Con A plus verapamil on splenic lymphocytes from control (n = 7 per group) and acute restraint-stressed (n = 7 per group) mice. Lymphocytes were stimulated for 42 h with Con A or Con A plus verapamil, and proliferation was measured by MTT assay. Verapamil was added 10 min before exposure to Con A. Data are presented as group means ± SEM. Statistically significant differences vs. control are indicated as: *P < 0.05, **P < 0.01. OD570, optical density at 570 nm.
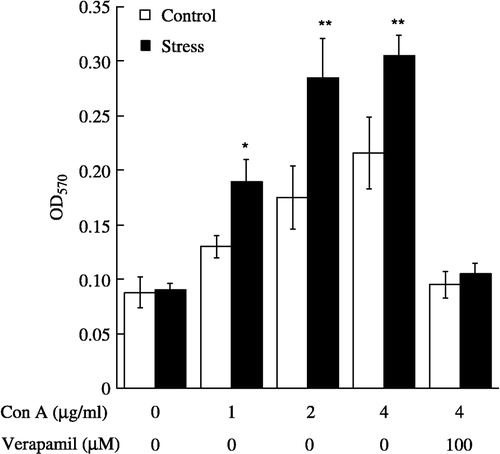
Though LPS (5–25 μg/ml) also caused a dose-dependent increase in the proliferation of lymphocytes from nonstressed and acute restraint-stressed mice (F(3, 48) = 25.53, p < 0.0001, ), exposure to acute restraint stress failed to enhance the LPS-stimulated proliferation (). Moreover, no significant interaction between group and mitogen concentration was observed. The dose-response curves of Con A- and LPS-stimulated proliferation in nonstressed mouse lymphocytes were similar to those reported by Lysle et al. (Citation1990), Mosmann (Citation1983) and Silberman et al. (Citation2002). Finally, the proliferation of resting lymphocytes was not affected by acute restraint stress.
Figure 4 Proliferative effect of LPS on splenic lymphocytes from control (n = 7 per group) and acute restraint-stressed (n = 7 per group) mice. Lymphocytes were stimulated for 42 h with various concentrations of LPS, and proliferation was measured by MTT assay. Data are presented as group means ± SEM. OD570, optical density at 570 nm.
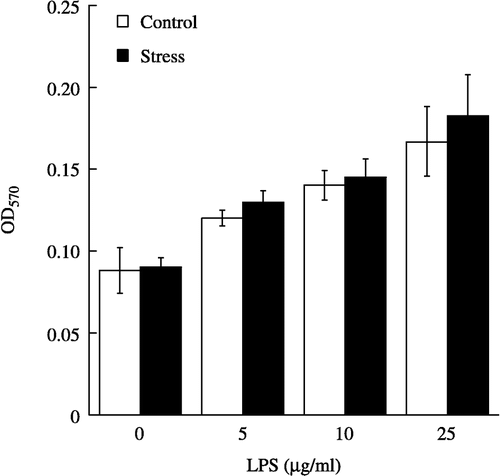
Discussion
In the present study, we observed that acute restraint stress significantly increases the plasma corticosterone concentration in ddY strain male mice. Physiological responses induced by stress involve activation of both the hypothalamic-pituitary-adrenal (HPA) axis and autonomic nervous system, with consequent increments in plasma glucocorticoids and catecholamines, respectively (Kvetnansky et al. Citation1971; Keim and Sigg Citation1976). Therefore, the acute restraint conditions in the present study were sufficient to induce a stress response in the mice and may have also increased plasma adrenaline/noradrenaline concentrations.
We demonstrated for the first time that acute restraint stress significantly increases the resting [Ca2+ ]i in lymphocytes. Three transport systems, i.e. the Ca2+ -ATPase of the plasma membrane, the Ca2+ -ATPase of the endoplasmic reticulum, and the Na+/Ca2+ exchanger, act in concert to regulate Ca2+ homeostasis in lymphocytes (Balasubramanyam et al. Citation1993; Lewis Citation2001), and we assume that one, two or all of these transport systems was/were modified by the acute restraint stress. To our knowledge, however, there is no report describing that glucocorticoid or adrenaline/noradrenaline modify calcium fluxes in lymphocytes.
Con A and LPS increase [Ca2+ ]i in T- and B-cells, respectively (Tsien et al. Citation1982; Rosoff and Cantley Citation1985; Proust et al. Citation1987). We demonstrated for the first time that prior acute restraint stress enhances a Con A-induced but not a LPS-induced increase in [Ca2+ ]i in mouse splenic lymphocytes, which indicates that acute restraint stress enhances the increase in [Ca2+ ]i in T-cells in particular.
Proust et al. (Citation1987) and Tsien et al. (Citation1982) reported that an increase in [Ca2+ ]i in mouse T-cells induced by Con A was largely inhibited by removal of extracellular Ca2+ . We obtained the same result (data not shown). The finding that verapamil abolished Con A-induced increases in [Ca2+ ]i in lymphocytes from both nonstressed and acute restraint-stressed mice in the present study suggests that acute restraint stress enhances the Con A-induced increase in [Ca2+ ]i by stimulating Ca2+ entry via a verapamil-sensitive pathway. However, Velbinger et al. (Citation2000) observed that acute immobilization stress did not influence a phytohemagglutinin (PHA)-induced increase in [Ca2+ ]i in splenic T-lymphocytes of rats. This disagreement with our finding could be due to various differences between the studies, such as rodent species, sex, stress conditions, housing conditions and light–dark cycle.
Lysle et al. (Citation1990) and Silberman et al. (Citation2002) observed that Con A and LPS stimulate the proliferation of mouse splenic lymphocytes, more specifically the proliferation of T- and B-cells, respectively. In the present study, we observed that acute restraint stress enhances Con A-stimulated but not LPS-stimulated lymphocyte proliferation, indicating that acute restraint stress further enhances T-cell proliferation. Bauer et al. (Citation2001) and Lysle et al. (Citation1990) also reported that exposure of rodents to acute stress increases mitogen-stimulated T-cell proliferation.
Con A and LPS mobilize Ca2+ by activating T- and B-cell receptors, respectively (Tsien et al. Citation1982; Rosoff and Cantley Citation1985; Proust et al. Citation1987) and an increase in [Ca2+ ]i constitutes an essential triggering signal for T- and B-cell proliferation (Klaus et al. Citation1986; Crabtree Citation1989). In the present study, there was a positive correlation between [Ca2+ ]i and T-cell proliferation following acute restraint stress, and verapamil completely suppressed the proliferative response to Con A in lymphocytes from both nonstressed and acute restraint-stressed mice. These results suggest that acute restraint stress enhances Con A-stimulated T-cell proliferation by increasing [Ca2+ ]i via stimulation of Ca2+ entry. Verapamil also significantly suppresses interleukin-2 (IL-2; a cytokine essential for T-cell proliferation) release by human peripheral T-cells (Drach et al. Citation1996). However, since an increase in [Ca2+ ]i is essential for IL-2 production by T-cells (Negulescu et al. Citation1994), it is unlikely that verapamil inhibits T-cell proliferation by this means.
Exposure to acute footshock stress has been reported to enhance the induction phase of the immune response (Wood et al. Citation1993). In addition, Millan et al. (Citation1996) found that short-term restraint stress (2 h on two consecutive days) enhances the primary serum T-cell-dependent antibody response to sheep red blood cells. Other authors, however, have reported a decreased response to a T-cell-dependent antigen following acute exposure to footshock (Laudenslager et al. Citation1988; Fleshner et al. Citation1996). These contradictory results can be explained by dependence of the response of the immune system to stress on several factors, including the intensity and/or the duration of the stress, the antigenic challenge, and how much time has passed between the application of the stressor and immunization (Zalcman et al. Citation1988; Zalcman and Anisman Citation1993; Millan et al. Citation1996).
Bauer et al. (Citation2001) suggested that increased splenic lymphocyte proliferation following acute restraint stress exposure might have important biological implications. Since the spleen is primarily involved in dealing with blood-borne pathogens, such as encapsulated bacteria (Mond et al. Citation1995), stress-induced activation of splenocyte function could enhance protection against blood-borne pathogens.
In the present study, an increase in splenic lymphocyte proliferation caused by Con A was enhanced by the exposure of mice to acute restraint stress. Bauer et al. (Citation2001) observed that acute restraint stress increases PHA-stimulated splenic lymphocyte proliferation and suppresses peripheral blood lymphocyte (PBL) proliferation in rats, and studies prior to theirs had demonstrated that footshock and immobilization stress decrease PBL proliferation in rats (Rinner et al. Citation1992; Shurin et al. Citation1994). Taken together with our finding that exposure to acute restraint stress enhances Con A-induced splenic lymphocyte proliferation in mice, this suggests that stress-induced immune changes are compartment-specific.
In conclusion, the present study demonstrates that acute restraint stress enhances Con A-stimulated T-cell proliferation by increasing [Ca2+ ]i via stimulation of Ca2+ entry. The influences of stress on immunity are mediated not only by glucocorticoids but also by catecholamines, endogenous opioids and pituitary hormones such as growth hormone (Dantzer and Kelley Citation1989). These substances could also play a role in mediating the effects of stress on lymphocytes. Additional studies are now needed to further clarify how acute restraint stress enhances Ca2+ mobilization and T-cell proliferation.
Acknowledgements
This study was supported by grants from the 21st Century COE Program (A-1) of the Ministry of Education, Culture, Sports, Science, and Technology of Japan and the Research Foundation of Obihiro University of Agriculture and Veterinary Medicine.
References
- Arora PK, Miller HC, Aronson LD. Protease inhibitor regulation of B-cell differentiation. Cell Immunol 1981; 60: 155–167
- Balasubramanyam M, Kimura M, Aviv A, Gardner JP. Kinetics of calcium transport across the lymphocyte plasma membrane. Am J Physiol 1993; 265: C321–C327
- Bauer ME, Perks P, Lightman SL, Shanks N. Restraint stress is associated with changes in glucocorticoid immunoregulation. Physiol Behav 2001; 73: 525–532
- Birx DL, Berger M, Fleisher TA. The interference of T cell activation by calcium channel blocking agents. J Immunol 1984; 133: 2904–2909
- Braun J, Sha'afi RI, Unanue ER. Crosslinking by ligands to surface immunoglobulin triggers mobilization of intracellular 45Ca2+ in B lymphocytes. J Cell Biol 1979; 82: 755–766
- Crabtree GR. Contingent genetic regulatory events in T lymphocyte activation. Science 1989; 243: 355–361
- Csermely P, Penzes I, Toth S. Chronic overcrowding decreases cytoplasmic free calcium levels in T lymphocytes of aged CBA/CA mice. Experientia 1995; 51: 976–979
- Dantzer R, Kelley KW. Stress and immunity: An integrated view of relationships between the brain and the immune system. Life Sci 1989; 44: 1995–2008
- Dhabhar FS, McEwen BS. Stress-induced enhancement of antigen-specific cell-mediated immunity. J Immunol 1996; 156: 2608–2615
- Dhabhar FS, McEwen BS. Acute stress enhances while chronic stress suppresses cell-mediated immunity in vivo: A potential role for leukocyte trafficking. Brain Behav Immun 1997; 11: 286–306
- Dhabhar FS, McEwen BS. Enhancing versus suppressive effects of stress hormones on skin immune function. Proc Natl Acad Sci USA 1999; 96: 1059–1064
- Dolmetsch RE, Lewis RS, Goodnow CC, Healy JI. Differential activation of transcription factors induced by Ca2+ response amplitude and duration. Nature 1997; 386: 855–858
- Drach J, Gsur A, Hamilton G, Xhao S, Angerler J, Fiegl M, Zojer N, Raderer M, Haberi I, Andreeff M, Huber H. Involvement of P-glycoprotein in the transmembrane transport of interleukin-2 (IL-2), IL-4, and interferon-γ in normal human T lymphocytes. Blood 1996; 88: 1747–1754
- Falciola J, Volet B, Anner RM, Moosmayer M, Lacotte D, Anner BM. Role of cell membrane Na+, K+-ATPase for survival of human lymphocytes in vitro. Biosci Rep 1994; 14: 189–204
- Feske S, Giltnane J, Dolmetsch R, Staudt LM, Rao A. Gene regulation mediated by calcium signals in T lymphocytes. Nat Immunol 2001; 2: 316–324
- Fleshner M, Brennan FX, Nguyen K, Watkins LR, Maier SF. RU-486 blocks differentially suppressive effect of stress on in vivo anti-KLH immunoglobulin response. Am J Physiol 1996; 271: R1344–R1352
- Fukui Y, Sudo N, Yu X-N, Nukina H, Sogawa H, Kubo C. The restraint stress-induced reduction in lymphocyte cell number in lymphoid organs correlates with the suppression of in vivo antibody production. J Neuroimmunol 1997; 79: 211–217
- Glick D, von Redlich D, Levine S. Fluorometric determination of corticosterone and cortisol in 0.02–0.05 milliliters of plasma or submilligram samples of adrenal tissue. Endocrinol 1964; 74: 653–655
- Grinstein S, Dixon SJ. Ion transport, membrane potential, and cytoplasmic pH in lymphocytes: Changes during activation. Physiol Rev 1989; 69: 417–481
- Grynkiewicz G, Poenie M, Tsien RY. A new generation of Ca2+ indicators with greatly improved fluorescence properties. J Biol Chem 1985; 260: 3440–3450
- Keim K, Sigg E. Physiological and biochemical concomitants of restraint stress in rats. Pharmacol Biochem Behav 1976; 4: 289–297
- Klaus GG, O'Garra A, Bijsterbosch MK, Holman M. Activation and proliferation signals in mouse B cells. VIII. Induction of DNA synthesis in B cells by a combination of calcium ionophores and phorbol myristate acetate. Eur J Immunol 1986; 16: 92–97
- Kusnecov AW, Rabin BS. Inescapable footshock exposure differentially alters antigen- and mitogen-stimulated spleen cell proliferation in rats. J Neuroimmunol 1993; 44: 33–42
- Kvetnansky R, Weise V, Kopin I. Synthesis of adrenal catecholamines in rats during and after immobilization. Endocrinol 1971; 89: 46–49
- Laudenslager ML, Fleshner M, Hofstadter P, Held PE, Simons L, Maier SF. Suppression of specific antibody production by inescapable shock: Stability under varying conditions. Brain Behav Immun 1988; 2: 445–452
- Lewis RS. Calcium signaling mechanisms in T lymphocytes. Annu Rev Immunol 2001; 19: 497–521
- Lysle D, Cunnick J, Rabin B. Stressor-induced alteration of lymphocyte proliferation in mice: Evidence for enhancement of mitogenic responsiveness. Brain Behav Immun 1990; 4: 269–277
- McEwen BS. Protective and damaging effects of stress mediators: Allostasis and allostatic load. N Engl J Med 1998; 338: 171–179
- Millan S, Gonzalez-Quijano MI, Giordano M, Sato L, Martin AL, Lopez-Calderon A. Short and long restraint differentially affect humoral and cellular immune functions. Life Sci 1996; 59: 1431–1442
- Mond J, Lees A, Shapper C. T cell-independent antigens type 2. Annu Rev Immunol 1995; 13: 655–692
- Mosmann T. Rapid colorimetric assay for cellular growth and survival: Application to proliferation and cytotoxicity assays. J Immunol Methods 1983; 65: 55–63
- Nakabayashi H, Komada H, Yoshida T, Takanari H, Izutsu K. Lymphocyte calmodulin and its participation in the stimulation of T lymphocytes by mitogenic lectins. Biol Cell 1992; 75: 55–59
- Negulescu PA, Shastri N, Cahalan MD. Intracellular calcium dependence of gene expression in single T lymphocytes. Proc Natl Acad Sci USA 1994; 91: 2873–2877
- Okimura T, Ogawa M, Yamauchi T, Sasaki Y. Stress and immune responses. IV. Adrenal involvement in the alteration of antibody responses in restraint-stressed mice. Jpn J Pharmacol 1986; 41: 237–245
- Proust JJ, Filburn CR, Harrison SA, Buchholz MA, Nordin AA. Age-related defect in signal transduction during lectin activation of murine T lymphocytes. J Immunol 1987; 139: 1472–1478
- Rinner I, Schauenstein K, Mangge H, Porta S, Kvetnansky R. Opposite effects of mild and severe stress on in vitro activation of rat peripheral blood lymphocytes. Brain Behav Immun 1992; 6: 130–140
- Rosoff PM, Cantley LC. Lipopolysaccharide and phorbol esters induce differentiation but have opposite effects on phosphatidylinositol turnover and Ca2+ mobilization in 70Z/3 pre-B lymphocytes. J Biol Chem 1985; 260: 9209–9215
- Satoh E, Ishii T, Nishimura M. Palytoxin-induced increase in cytosolic-free Ca2+ in mouse spleen cells. Eur J Pharmacol 2003; 465: 9–13
- Sei Y, McIntyre T, Skolnick P, Arora PK. Stress modulates calcium mobilization in immune cells. Life Sci 1991; 49: 671–676
- Shurin M, Zhou D, Kusnecov A, Rassnick S, Rabin B. Effect of one or more footshocks on spleen and blood lymphocyte proliferation in rats. Brain Behav Immun 1994; 8: 57–65
- Silberman DM, Wald M, Genaro AM. Effects of chronic mild stress on lymphocyte proliferative response. Participation of serum thyroid hormones and corticosterone. Int Immunopharmacol 2002; 2: 487–497
- Silberman DM, Wald MR, Genaro AM. Acute and chronic stress exert opposing effects on antibody responses associated with changes in stress hormone regulation of T-lymphocyte reactivity. J Neuroimmunol 2003; 144: 53–60
- Silberman DM, Ayelli-Edgar V, Zorrilla-Zubilete M, Zieher LM, Genaro AM. Impaired T-cell dependent humoral response and its relationship with T lymphocyte sensitivity to stress hormones in a chronic mild stress model of depression. Brain Behav Immun 2004; 18: 81–90
- Stark JL, Avitsur R, Padgett DA, Campbell KA, Beck FM, Sheridan JF. Social stress induces glucocorticoid resistance in macrophages. Am J Physiol 2001; 280: R1799–R1805
- Teague TK, Hildeman D, Kedl RM, Mitchell T, Rees W, Schaefer BC, Bender J, Kappler J, Marrack P. Activation changes the spectrum but not the diversity of genes expressed by T cells. Proc Natl Acad Sci USA 1999; 96: 12691–12696
- Tournier JN, Mathieu J, Mailfert Y, Multon E, Drouet C, Jouan A, Drouet E. Chronic restraint stress induces severe disruption of the T-cell specific response to tetanus toxin vaccine. Immunol 2001; 102: 87–93
- Tsien RY, Pozzan T, Rink TJ. T-cell mitogens cause early changes in cytoplasmic free Ca2+ and membrane potential in lymphocytes. Nature 1982; 295: 68–71
- van Leeuwen JE, Samelson LE. T cell antigen-receptor signal transduction. Curr Opin Immunol 1999; 11: 242–248
- Velbinger K, De Vry J, Jentzsch K, Eckert A, Henn F, Muller WE. Acute stress induced modifications of calcium signaling in learned helpless rats. Pharmacopsychiatry 2000; 33: 132–137
- Wood PG, Karol MH, Kusnecov AW, Rabin BS. Enhancement of antigen-specific humoral and cell-mediate immunity by electric footshock stress in rats. Brain Behav Immun 1993; 7: 121–134
- Zalcman S, Anisman H. Acute and chronic stressor effects on the antibody response to sheep red blood cells. Pharmacol Biochem Behav 1993; 46: 445–452
- Zalcman S, Minkiewics-Janda A, Richter M, Anisman H. Critical periods associated with stressor effects on antibody titers and on the plaque-forming cell response to sheep red blood cells. Brain Behav Immun 1988; 2: 254–266
- Zhang D, Kishihara K, Wang B, Mizobe K, Kubo C, Nomoto K. Restraint stress-induced immunosuppression by inhibiting leukocyte migration and Th1 cytokine expression during intraperitoneal infection of Listeria monocytogens. J Neuroimmunol 1998; 92: 139–151