Abstract
The objective of the present study was to investigate the effect of acute exposure to electric tail shock stress (ES) and a stress witnessing procedure (SW), as models for physical and psychological stress paradigms, respectively on adherence, phagocytosis and hydrogen peroxide (H2O2) release from rat peritoneal macrophages. In addition, we studied the in vitro effects of corticosterone (CORT), neuropeptide Y (NPY) and beta-endorphin (BE) on adherence, phagocytosis and H2O2 release from macrophages isolated from control rats and from rats that had been exposed to ES or SW procedures 24 h earlier. ES and SW comparably diminished phagocytosis and H2O2 release, but did not influence macrophage adherence. In vitro treatment with CORT and NPY notably suppressed phagocytosis and potentiated H2O2 release from macrophages. BE suppressed both phagocytosis and H2O2 release from macrophages. Previous exposure to ES and SW altered the responsiveness of the isolated macrophages to their in vitro treatment with mediators of stress, making the cells less sensitive to the influence of CORT and NPY and to a lesser extent to BE. It could be concluded that changes in the local macrophage milieu induced by ES and SW 24 h earlier modify macrophage responses to subsequent in vitro exposure to the stress mimics, CORT, NPY and BE.
Introduction
Stress is associated with an altered homeostatic state of the organism, involving behavioural, endocrine and immunological changes (McEwen Citation1998). Various inputs indicative of stress converge on the final common pathways within the brain. This is illustrated by the combined roles in the central construction of the responses to stress of the corticotropin-releasing hormone (CRH) neurons of the paraventricular nucleus (PVN) of the hypothalamus and CRH neurons in the medulla, together with catecholaminergic neurons of the locus coeruleus and the medulla and pons (Whitnall Citation1993; Stratakis and Chrousos Citation1995; Chrousos Citation2000).
The final products of the activation of the hypothalamic–pituitary–adrenal (HPA) axis during stress are glucocorticoids that prepare the organism for changes in energy and metabolism required to deal with the stress (Munck et al. Citation1984; Whitnall Citation1993; Stratakis and Chrousos Citation1995). Human cortisol and rodent corticosterone (CORT) are commonly connected with stress-induced immunosuppression. However, glucocorticoids influence immune functions in a more complex manner by controlling the individual repertoire of immunological responses including the direction and magnitude of immune reactions (Wilckens Citation1995; Wilckens and De Rijk Citation1997).
Sympathetic nerve fibres that innervate various organs, including lymphoid organs, also directly contact macrophages, T-lymphocytes and mast cells from non-organised lymphoid compartments (Stevens-Felten and Bellinger Citation1997). Cells residing within lymphoid organs (Bellinger et al. Citation2001) and from non-organised lymphoid compartments (Stevens-Felten and Bellinger Citation1997) are also exposed to neuropeptide Y (NPY) co-localised and co-released with norepinephrine from noradrenergic nerve fibres upon stimulation. It was noted that in some pathological conditions, such as in septic shock, the sensitivity of dilated blood vessels to norepinephrine significantly decreases while NPY retains its vasoconstrictive properties (Hauser et al. Citation1995). NPY, a 36 amino acid peptide, is one of the most abundant and widely distributed peptides in the central and peripheral nervous system (Baker and Herkenham Citation1995). In response to acute stress, NPY mRNA expression increases within discrete regions of the brain (Conrad and McEwen Citation2000) and the adrenal medulla (Hiremagalur et al. Citation1994). The release of NPY during stress (Grundemar and Hakanson Citation1993; Puybasset et al. Citation1993) provides the basis for a role of this peptide in stress-induced immune alterations (Bedoui et al. Citation2003). Both lymphocytes and macrophages produce NPY after their activation, a process that facillitates the autocrine/paracrine action of NPY in the immune system (Schwarz et al. Citation1994; Wheway et al. Citation2005).
Versatile adaptive responses to stress also involve the release of the opioid peptide beta-endorphin (BE),a 31 amino acid peptide hormone that is cleaved from proopiomelanocortin (POMC) along with several other products with variable physiological activity via a proteolytic process (Heijnen et al. Citation1991). BE is primarily synthesised in the hypothalamus and the anterior and intermediate lobes of the pituitary (Stratakis and Chrousos Citation1995; Smith and Funder Citation1988), but also in monocytes, granulocytes, lymphocytes and macrophages (Mousa et al. Citation2004). Stress significantly increases the BE concentration in plasma and within immune cells (Sacerdote et al. Citation1994; Tsukada et al. Citation2001), which together could contribute to stress-induced immunomodulation (Hale et al. Citation2001).
We have previously shown that exposure to electric tail shock stress (ES) profoundly suppresses the humoral immune response in rats, whereas witnessing electric tail shock administration (SW) potentiates it (Stanojević et al. Citation2003). It is suggested that alterations in the immune response observed after stress could be specifically attributed to stress-induced changes in macrophage functions as these cells are intimately involved in the development of the humoral and cellular immune responses (Fleshner et al. Citation1995a; Kizaki et al. Citation1996). Therefore, we examined if acute exposure to ES and SW, representing physical and psychological stress paradigms, respectively, affected adherence, phagocytosis and H2O2 release from peritoneal macrophages.
Several lines of evidence suggest that a previous stressful experience changes the response to a subsequent stressor via a mechanism involving multiple physiological systems (O'Connor et al. Citation2003). A previous stressful experience may lead to sensitisation (Piazza and Le Moal Citation1998) or to habituation (De Boer et al. Citation1990) of a particular response. This depends on whether the subsequent stressor is homotypic or heterotypic. Both phenomena are evident in many aspects of human stress-related disorder (Tilders and Schmidt Citation1999). The rationale behind this study was that exposure to acute stress could induce functional changes within macrophages thereby altering their response to artificial stressors as mimicked by CORT, NPY and BE in vitro 24 h later. We tested this by studying the in vitro effects of CORT, NPY and BE on adherence, phagocytosis and H2O2 release from peritoneal macrophages isolated from rats that had been exposed to ES or SW procedures 24 h earlier.
Materials and methods
Animals
Young adult male Albino Oxford (AO) rats (60 ± 3 days of age) were obtained from our breeding colony in the Immunology Research Centre “Branislav Janković” in Belgrade. Rats were housed individually in perspex-walled cages with free access to food and water. Colony conditions were maintained using 12 h light and 12 h dark cycles (lights on at 08:00). All animal procedures were approved by our Institutional Animal Care and Use Committee and followed guidelines described in the European Community's Council Directive dated 24th November 1986 (86/609/EEC).
Reagents
BE, CORT, phenol red, phorbol myristate acetate (PMA), horseradish peroxidase (EC 1.11.1.7) and zymosan A (isolated from Saccharomyces cerevisiae) were obtained from Sigma (St Louis, MO, USA). Human/rat NPY was purchased from Polypeptide Laboratories (Wolfenbuttel, Germany). Nitro blue tetrazolium chloride (NBT, 2,2′-di-p-nitrophenyl-5,5′-diphenyl-3,3′-[3,3′-dimethoxy-4,4′-diphenylene]ditetrazolium chloride) was obtained from Serva (Heidelberg, Germany). Thioglycolate medium and phenol red-free minimal essential medium (MEM) were both acquired from the “Torlak” Institute in Belgrade.
Stress procedures
Rats were intraperitoneally injected with 15 ml of thioglycolate medium in order to induce recruitment of circulatory monocytes into the peritoneum and proliferation of resident peritoneal macrophages (Eichner and Smeaton Citation1983; Melnicoff et al. Citation1989). Stress procedures were performed six days later and took place between 08:00 and 09:30. Each experimental group consisted of eight rats.
ES was delivered in a soundproof room into which the rats had been moved immediately prior to initiating the stress procedure. During the shock procedure the rats were kept in rectangular perspex-walled boxes (18 × 9.5 × 20 cm) containing a semicircular aperture on the lower part of the rear wall that allowed attachment of the electrodes to the rat's tail and prevented movements of rear paws. Electric shocks were delivered via two silver electrodes (separated by 3–4 cm) connected to the tail with the aid of electrode paste and adhesive tape. Four rats were simultaneously shocked with the same intensity (55 V, 1 mA). A shock session consisted of eighty 5 s unsignalled shocks (seven electrical impulses of 0.3 s duration and 0.6 s pauses) with an average inter-shock interval of 60 s (range 5–120 s). In this way, the total duration was 80–90 min. After the shock session, the rats were returned to their colony. As the experimental model of ES involved the exposure of rats to a series of unpredictable, inescapable and uncontrollable electric tail shocks accompanied by early and late opioid-mediated analgesia, it was regarded as both a physical and a psychological challenge that resulted in immunological changes (Maier et al. Citation1983; Shavit et al. Citation1984; Sutton et al. Citation1994). It has been shown that such a procedure induces a several-fold increase in the plasma level of CORT (Johnson et al. Citation2002).
SW involved rats witnessing the procedure of shock delivery to the rats in the ES group. Rats were moved to a soundproof room immediately prior to the stress session at the same time as the rats belonging to the ES group. SW rats remained there during the ES procedure. Specifically, SW rats were kept in their regular cages and witnessed the whole ES procedure. After the ES session, SW rats were returned to their colony. The model of witnessing the ES procedure involved exposure of caged rats to pheromones (Fanselow Citation1985) and ultrasonic vocalisation (Knutson et al. Citation2002) emitted from rats experiencing the ES procedure. Therefore, this experimental design ensures primarily psychological stress for the witnessing rats. Rats witnessing rats experiencing the ES procedure increase CORT secretion (Ottenweller et al. 1989) and experience opioid-mediated analgesia (Moynihan et al. Citation2000). This stress paradigm also produces immunological changes thereby confirming that physically stressed rats can influence non-physically stressed rats via mechanisms involving vocalisation and pheromones (Fernandes Citation2000; Moynihan et al. Citation2000).
Serving as controls were intact control (IC) rats left undisturbed in their regular cages in the colony.
Isolation of macrophages and their cell surface phenotyping
About 24 h after shock termination (on the seventh day after injection of thioglycolate medium) macrophages were obtained by peritoneal lavage with 10 ml of MEM. Seven days after injection of thioglycolate medium the majority of the cells in peritoneal exudates are macrophages (Segura et al. Citation1996). Peritoneal lavages were centrifuged (250 g, 10 min) and the resulting cell pellets were washed three times using phosphate buffer in order to prepare the fresh macrophage suspensions for the assays. This procedure allowed adequate recovery of cells (greater than 95% viable as determined by trypan blue exclusion).
The cells (1 × 107/ml) were stained with mouse anti-rat CD11b IgG-FITC (clone number ED8, Serotec, Oxford, UK) and mouse anti-rat CD68-biotin (clone number ED1, Serotec, Oxford, UK) followed by streptavidin RPE (STAR4A/B) as a second step reagent (Becton Dickinson, San Jose CA, USA). Analysis on a FACScan flow cytometer (Cell Quest software, Becton Dickinson) by means of FSC and SSC of a total of 104 flow cytometric events confirmed that the majority (75.8 ± 3.6%) of cells in the peritoneal lavages were ED1 + CD11b+ macrophages.
Macrophage adherence
A previously published method (Oez et al. Citation1990) was followed. Briefly, 96-well flat-bottomed tissue culture plates (Linbro, ICN Biomedicals, Aurora, OH, USA) were filled with 50 μl of MEM, or CORT (2 × 10− 9–2 × 10− 5 M in MEM), NPY (2 × 10− 14–2 × 10− 6 M in MEM) or BE (2 × 10− 14–2 × 10− 6 M in MEM) together with 50 μl of individual cell samples (2.5 × 106 cells/ml, eight individual cell samples per group, in duplicate). The plates were incubated for 1 h at 37°C in 95% air–5% CO2 before being washed twice with warm (37°C) MEM. The adherent cells were fixed with methanol, stained with 0.1% methylene blue and then washed thoroughly with distilled water. The plates were dried overnight at room temperature. The dye was dissolved in 0.1 M HCl and the optical density (OD) was determined at 620 nm using an Multiskan Ascent platereader (Labsystems, Helsinki, Finland). The results were expressed as OD (mean ± SEM).
Phagocytosis assay
Phagocytosis was determined as previously described (Pick et al. Citation1981). Briefly, macrophage suspensions adjusted to 2.5 × 106 cells/ml (eight individual cell samples per experimental group, in duplicate) were plated (100 μl/well) in 96-well flat-bottomed tissue culture plates. The plates were incubated for 2 h at 37°C in 95% air–5% CO2. Non-adherent cells were removed by washing the plates twice with warm (37°C) MEM. Adherent macrophages were stimulated with 50 μl of zymosan (125 μg/ml) in the absence or presence of CORT (2 × 10− 8–2 × 10− 5 M), NPY (2 × 10− 12–2 × 10− 6 M) or BE (2 × 10− 12–2 × 10− 6 M) and in the presence of 50 μl of NBT (0.5 mg/ml in MEM) for 30 min at 37°C in 95% air–5% CO2. The cells were then fixed with methanol, the plates were air-dried and ODs were determined at 545 nm. The amount of engulfed zymosan particles was proportional to the reduction of yellow NBT to blue formazan. The results were expressed as OD (mean ± SEM).
H2O2 release assay
H2O2 release was determined according to a previously published method (Pick and Mizel Citation1981). Briefly, macrophage suspensions adjusted to 2.5 × 106 cells/ml (eight individual cell samples per group, in duplicate) were adhered to tissue culture plates for 2 h at 37°C in 95% air–5% CO2 (non-adherent cells were removed by washing the plates twice with warm MEM, as previously described). Adherent macrophages were primed for H2O2 release with 100 μl of 25 nM PMA in phenol red solution (10 mM potassium phosphate buffer pH 7, 140 mM NaCl, 5.5 mM dextrose, 0.56 mM phenol red and 19 U/ml of horseradish peroxidase) in the absence or presence of CORT (10− 9–10− 5 M), NPY (10− 14–10− 6 M) or BE (10− 14–10− 6 M). PMA was prepared from stock solution (10− 2 M in DMSO, stored at − 70°C). The 96-well plates were incubated for 1 h at 37°C in 95% air–5% CO2. Incubations were terminated with 10 μl of 0.5 M NaOH and ODs were determined at 620 nm. The concentration of H2O2 in the samples was calculated using standard concentrations of H2O2 (1–40 μM). The results were expressed as nM of H2O2 produced per mg of cell protein (mean ± SEM).
Statistical analysis
Statistical analysis of the data was performed using the StatView II computer programme. The main effects of ES and SW were analysed by one-way ANOVA. The effects of CORT, NPY and BE were analysed by one-factor ANOVA for repeated measures (factor: concentration). Fisher's PLSD test was used for post hoc analysis for the evaluation of differences between groups (indicated in the figures with asterisks, *). Differences were regarded as statistically significant if p < 0.05.
Results
The effect of stress on macrophage adherence, phagocytosis and H2O2 release
Macrophage adherence was not affected by ES or SW (F2,21 = 0.6; p = 0.5617; (A)). However, both ES and SW diminished zymosan phagocytosis (F2,21 = 13.5; p < 0.001; (B)) and reduced H2O2 release (F2,21 = 4.4; p < 0.05; (C)).
Figure 1 The effect of acute exposure to ES and a SW on: (A) adherence, (B) zymosan particle phagocytosis and (C) PMA-primed H2O2 release from peritoneal macrophages isolated 24 h after stress. The values represent the mean (n = 8) ± SEM. Statistically significant differences: *, p < 0.05; and **, p < 0.01 vs. the IC group of rats.
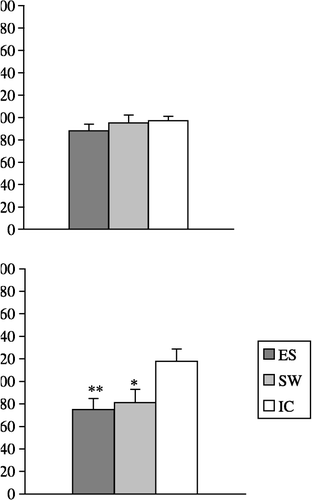
The effect of in vitro treatment with CORT, NPY and BE on macrophage adherence
CORT (10− 7–10− 5 M) slightly (but significantly) decreased IC rat macrophage adherence (F5,35 = 5.3; p < 0.001). In contrast, CORT more effectively diminished both ES and SW rat macrophage adherence (F5,35 = 10.8; p < 0.0001 and F5,35 = 4.3; p < 0.01, respectively; (A)).
Figure 2 The effect of in vitro treatment with: (A) CORT, 10− 9–10− 5 M; (B) NPY, 10− 14–10− 6 M; and (C) BE, 10− 14–10− 6 M on the adherence of macrophages isolated from IC rats, from rats exposed to acute ES and from rats exposed to a SW 24 h earlier. The values represent the mean (n = 8) ± SEM. Statistically significant differences: *, p < 0.05; **, p < 0.01; and ***, p < 0.001 vs. 0.
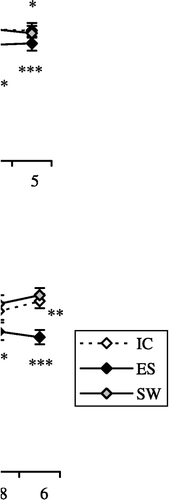
Only the highest concentration of NPY (10− 6 M) increased IC rat macrophage adherence (F5,35 = 6.4; p < 0.001). However, a wide range of NPY concentrations decreased both ES and SW rat macrophage adherence (10− 12–10− 6 M; F5,35 = 6.9; p < 0.0001 and 10− 14–10− 12 M; F5,35 = 6.1; p < 0.001, respectively, (B)).
BE decreased ES rat macrophage adherence (F5,35 = 2.7; p < 0.05) but failed to have any effect on both IC and SW rat macrophage adherence (F5,35 = 0.6; p = 0.7036 and F5,35 = 2; p = 0.0958, respectively; (C)).
The effect of in vitro treatment with CORT, NPY and BE on macrophage phagocytosis
CORT (10− 8–10− 5 M) strongly suppressed the phagocytic ability of macrophages isolated from both IC (F4,28 = 48.7; p < 0.0001) and SW rats (F4,28 = 30.7; p < 0.0001) but failed to influence the phagocytic ability of macrophages isolated from ES rats (F4,28 = 2.5; p = 0.0673; (A)).
Figure 3 The effect of in vitro treatment with: (A) CORT, 10− 8–10− 5 M; (B) NPY, 10− 12–10− 6 M; and (C) BE, 10− 12–10− 6 M on zymosan phagocytosis by macrophages isolated from IC rats, from rats exposed to acute ES and from rats exposed to a SW 24 h earlier. The values represent the mean (n = 8) ± SEM. Statistically significant differences: *, p < 0.01; and **, p < 0.001 vs. 0.
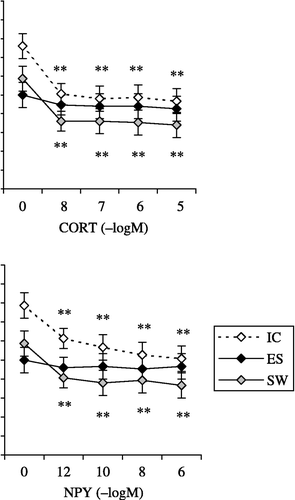
NPY (10− 12–10− 6 M) suppressed the phagocytic ability of macrophages isolated from both IC (F4,28 = 23.6; p < 0.0001) and SW rats (F4,28 = 21.4; p < 0.0001) but did not affect the phagocytic ability of macrophages isolated from ES rats (F4,28 = 2.5; p = 0.0785), in comparison with phagocytosis of zymosan in non-treated macrophages from IC group ((B)).
BE significantly decreased the phagocytic ability of macrophages isolated from all three experimental groups of rats (IC, F4,28 = 69.3; p < 0.0001; ES, F4,28 = 44.1; p < 0.0001 and SW, F4,28 = 36.5; p < 0.0001; (C)).
The effect of in vitro treatment with CORT, NPY and BE on macrophage H2O2 release
A low concentration of CORT (10− 9 M) significantly increased IC rat macrophage H2O2 release whereas a higher concentration (10− 5 M) significantly suppressed macrophage H2O2 release (F5,35 = 15.5; p < 0.0001; (A)). CORT (only at 10− 5 M) decreased ES rat macrophage H2O2 release (F5,25 = 8.2; p < 0.0001). Lower CORT concentrations (ranging from 10− 8 to 10− 5 M) strongly inhibited SW rat macrophage H2O2 release (F5,35 = 23.5; p < 0.0001).
Figure 4 The effect of in vitro treatment with: (A) CORT, 10− 9–10− 5 M; (B) NPY, 10− 14–10− 6 M; and (C) BE, 10− 14–10− 6 M on PMA-primed H2O2 release from macrophages isolated from IC rats, from rats exposed to acute ES and from rats exposed to a SW 24 h earlier. The values represent the mean (n = 6–8) ± SEM. Statistically significant differences: *, p < 0.05; **, p < 0.01; and ***, p < 0.0001 vs. 0.
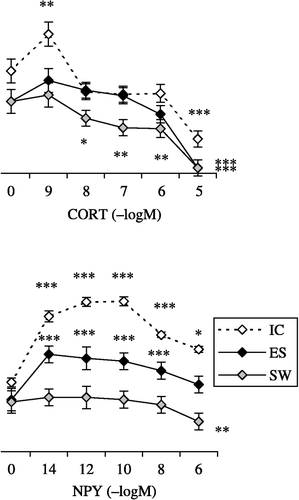
NPY (at all tested concentrations) strongly increased IC rat macrophage H2O2 release (F5,30 = 14.6; p < 0.0001). The effect of NPY on H2O2 release from macrophages isolated from ES rats was less intense (F5,25 = 11; p < 0.0001). NPY decreased H2O2 release from SW rat macrophages (F5,25 = 4.7; p < 0.01; (B)).
BE exerted interesting effects on macrophage H2O2 release. BE (10− 10–10− 6 M) decreased IC rat macrophage H2O2 release (F5,35 = 40.1; p < 0.0001), both increased (at 10− 12 M) and decreased (at 10− 8 and 10− 6 M) SW rat macrophage H2O2 release (F5,35 = 19.4; p < 0.0001) and at concentrations from 10− 14 to 10− 8 M significantly increased ES rat macrophage H2O2 release (F5,35 = 14.3; p < 0.0001; (C)).
Discussion
Both ES and SW diminished macrophage phagocytotic activity and H2O2 release without affecting macrophage adherence. The diminished response to stress mediators in the early phases of macrophage activation and suppressive influence of stress on later activation steps suggest that after ES and SW macrophages retain the ability to leave their local microenvironment and to adhere to smooth surfaces of both tissue membranes and target cells whilst being unable to mount full inflammatory response. The physiological purpose of such a regulatory mechanism induced by stress could be beneficial, since it reduces inflammation. However, since local inflammation restricts spreading of an infection throughout the body, anti-inflammatory effect of stress could also be detrimental.
Our findings that high concentrations of CORT diminished macrophage adherence while a low concentration increased H2O2 release together support the general view that the physiological role of endogenous glucocorticoids is to maintain and optimise the immune response (Fleshner et al. Citation2001), since low concentrations of CORT produce immunoenhancement while higher concentrations are immunosuppressive (Dhabhar and McEwen Citation1999). However, CORT diminished zymosan engulfment at all concentrations tested. Phagocytotic capability and H2O2 release represent two key macrophage parameters, but both indicate oxidative products after the respiratory burst (Pick and Mizel Citation1981; Pick et al. Citation1981). The main difference emanates from the divergence in the route of macrophage activation since zymosan stimulates the respiratory burst by phagocytosis via beta-glucan and CR3 complement receptors (Giaimis et al. Citation1993; Le Cabec et al. Citation2000) while PMA directly activates protein kinase C (PKC) and stimulates the oxidative burst without phagocytosis (Johnston and Kitagawa Citation1985; Chanok et al. Citation1994). In view of this difference, it may be that by decreasing macrophage adherence CORT allows in vivo a greater number of cells to leave the bloodstream. However, by diminishing phagocytosis CORT tends to restrict the likelihood of tissue damage from the subsequent respiratory burst and H2O2 spillover.
In contrast, NPY increased macrophage adherence in line with the finding that NPY increased the adhesion of human neutrophils and a monocytic cell line to endothelial cells (Sung et al. Citation1991). It has been demonstrated that NPY also increases adherence of lipopolysaccharide (LPS)-stimulated macrophages that, besides the cardiovascular actions of NPY (Hauser et al. Citation1995), could in part contribute to its ability to improve survival in experimental endotoxemia (Nave et al. Citation2004). NPY diminished zymosan particle phagocytosis, measured using an assay relying on NBT reduction. However, NPY strongly potentiated the respiratory burst and H2O2 release from macrophages stimulated with PMA (which activates PKC) and this is in line with the ability of NPY to activate PKC causing its translocation from the cytosol to plasma membrane (De la Fuente et al. Citation1993). We have previously shown that NPY augments H2O2 release from macrophages isolated from inbred Dark Agouti (DA) rats (Dimitrijević et al. Citation2005).
BE suppressed zymosan phagocytosis and H2O2 release without any influence on macrophage adherence. This result mimicked to some extent the effects of ES and SW. The lack of influence of BE on macrophage adherence is in line with the findings of Ortega et al. (Citation1996). Furthermore, we have recently shown that the suppressive effect of BE on inflammatory cells in vitro paralleled its anti-inflammatory effect in vivo (Stanojević et al. Citation2006).
The second part of our study sought to determine if exposure to ES or SW paradigms (which exerted comparable effects on adherence, phagocytosis and H2O2 release from peritoneal macrophages) could differentially alter macrophage responses to subsequent in vitro treatment with CORT, NPY and BE 24 h later. Our results revealed that ES neutralised the diminishing effect of CORT on zymosan phagocytosis. Moreover, ES and SW counteracted the stimulatory effect of low concentration CORT on PMA-primed H2O2 release. It has been reported that chronic social stress induces a state of glucocorticoid resistance in stimulated macrophages (Quan et al. Citation2003). Interestingly, exposure to acute inescapable stress is sufficient to induce resistance of LPS-induced HPA axis activation to the suppressive effect of dexamethasone 24 h after shock termination (O'Connor et al. Citation2003), implying long term alterations in the response to glucocorticoids after a single stress episode. A long-lasting increase in the level of CORT coupled with a decrease in corticosteroid-binding globulin after acute stress exposure probably contribute to these observed effects (Fleshner et al. Citation1995b). Although our study could not establish the precise mechanisms implicated in the changes of reactivity of macrophages isolated from stressed rats to low dose CORT, it could be that acute stress exposure accompanied by a second stimulatory signal, such as zymosan or PMA in vitro, rendered macrophages less sensitive to in vitro treatment with CORT due to stress-induced changes at the level of glucocorticoid receptors or post-receptor signalling events.
Both ES and SW negatively regulated the effects of NPY on all three measured macrophage functions. In contrast, ES and SW only affected H2O2 release by BE. From our observations one could speculate that stress exposure readily produces a state of NPY “resistance” in macrophages. To the best of our knowledge, there is no previously available literature concerning stress-induced changes in the sensitivity to NPY and BE of cells within the immune system despite both peptides being involved in the modulation of the immune response during stress. It has been previously demonstrated that the basal rat plasma level of NPY (around 195 fM) increases (by approximately 32%) on handling and increases further during electric shock (Castagne et al. Citation1987). In addition, it has been reported that foot-shock stress induces a significantly greater increase in the level of BE (up to 800 fM in plasma) than a milder form of psychological stress (up to 300 fM) (Tsukada et al. Citation2001). Both peptides bind to specific receptors that are members of the seven transmembrane domain/G-protein coupled receptor family. However, significant differences in the downstream signal transduction mechanisms of BE (Shahabi et al. Citation2003) and NPY (Selbie et al. Citation1995) probably contribute to their differential sensitivity to the impact of the preceding stress.
During stress stimulation, neuroendocrine mediators released from central and peripheral sites make contact with peritoneal macrophages via the circulation and neural projections (Friedman and Irwin Citation1995). However, macrophages express all the necessary pathway components to regulate the synthesis, the processing and the release of BE (Mousa et al. Citation2004). Furthermore, stress significantly increases BE concentration in immune system cells (Sacerdote et al. Citation1994). It has also been suggested that NPY could be produced by activated macrophages, providing an important autocrine signal for the regulation of macrophage function (Wheway et al. Citation2005). Thus, stress mediators released from multiple sites could converge on macrophages and modify their functions. Therefore, the in vitro addition of peptides/hormones to macrophages that have already been functionally altered due to their previous exposure to increased levels of stress mediators 24 h earlier could interfere with macrophage activity at the level of membrane receptors or at the level of post-receptor signalling pathways.
In summary, our current study has clearly demonstrated that exposure to acute physical and psychological stressors results in similar effects and that in vitro treatment with CORT, NPY and BE exerts differential effects on macrophage function. Prior exposure to ES and SW affected macrophage responses to chemical mediators of stress responses, namely CORT, NPY and BE in vitro, 24 h later. It can be concluded that macrophages may participate in mechanisms supporting the phenomenon of stress-induced alterations of the immune system's response to subsequent stressors.
Acknowledgements
The technical assistance of Mrs Zuzana Tomaš is gratefully acknowledged. This work was supported by the Ministry of Science and Environmental Protection of the Republic of Serbia (project number 145049).
References
- Baker RA, Herkenham M. Arcuate nucleus neurons that project to the hypothalamic paraventricular nucleus: Neuropeptidergic identity and consequences of adenalectomy on mRNA levels in the rat. J Comp Neurol 1995; 358: 518–530
- Bedoui S, Kawamura N, Straub RH, Pabst R, Yamamura T, von Horsten S. Relevance of neuropeptide Y for the neuroimmune crosstalk. J Neuroimmunol 2003; 134: 1–11
- Bellinger DL, Lorton D, Lubahn C, Felten DL. Innervation of lymphoid organs—association of nerves with cells of the immune sytem and their implications in disease. Psychoneuroimmunology3rd ed., R Ader, DL Felten, N Cohen. Academic Press, New York 2001; 55–111
- Castagne V, Corder R, Gaillard R, Mormede P. Stress-induced changes of circulating neruopeptide Y in the rat: Comparison with catecholamines. Regul Pept 1987; 19: 55–63
- Chanok SJ, El Benna J, Smith RM, Babior BM. The respiratory burst oxidase. J Biol Chem 1994; 269: 24519–24522
- Chrousos G. The stress response and immune function: Clinical implications: The 1999 Novera Herbert Spector lecture. Ann NY Acad Sci 2000; 917: 38–67
- Conrad CD, McEwen BS. Acute stress increases neuropeptide Y mRNA within the arcuate nucleus and hilus of the dentate gyrus. Brain Res Mol Brain Res 2000; 79: 102–109
- De Boer SF, Koopmans SJ, Slangen JL, Van der Gugten J. Plasma catecholamine, corticosterone and glucose responses to repeated stress in rats: Effect on interstressor interval length. Physiol Behav 1990; 47: 1117–1124
- De la Fuente M, Bernaez I, Del Rio M, Hernanz A. Stimulation of murine peritoneal macrophage functions by neuropeptide Y and peptide YY. Involvement of protein kinase C. Immunology 1993; 80: 259–265
- Dhabhar FS, McEwen BI. Enhancing versus suppressive effects of stress hormones on skin immune function. Proc Natl Acad Sci USA 1999; 96: 1059–1064
- Dimitrijević M, Stanojević S, Vujić V, Beck-Sickinger A, von Hoersten S. Neuropeptide Y and its receptor subtypes specifically modulate rat peritoneal macrophage functions in vitro: Counter regulation through Y1 and Y2/5 receptors. Regul Pept 2005; 124: 163–172
- Eichner RD, Smeaton TC. Agar accumulates in rat peritoneal macrophages elicited with thioglycollate broth. Scand J Immunol 1983; 18: 259–263
- Fanselow MS. Odors released by stressed rats produce opioid analgesia in unstressed rats. Behav Neurosci 1985; 99: 589–592
- Fernandes GA. Immunological stress in rats induces bodily alteration in saline-treated conspecifics. Physiol Behav 2000; 69: 221–230
- Fleshner M, Bellgrau D, Watkins LR, Laudenslager ML, Maier SF. Stress-induced reduction in the rat mixed lymphocyte reaction is due to macrophages and not to changes in T cell phenotypes. J Neuroimmunol 1995a; 56: 45–52
- Fleshner M, Deak T, Spencer RL, Laudenslager ML, Watkins LR, Maier SF. A long-term increase in basal levels of corticosterone and a decrease in corticosteroid-binding globulin after acute stressor exposure. Endocrinology 1995b; 136: 5336–5342
- Fleshner M, Deak T, Nguyen K, Watkins LR, Maier SF. Endogenous glucocorticoids play a positive regulatory role in the anti-keyhole limpet hemocyanin in vivo antibody response. J Immunol 2001; 166: 3813–3819
- Friedman EM, Irwin MR. A role for CRH and the sympathetic nervous system in stress-induced immunosupression. Stress: Basic mechanisms and clinical implications, GP Chrousos, R McCarty, K Pacak, G Cizza, E Sternberg, P Gold. Annals of the New York Academy of Sciences, New York 1995; Vol 771: 396–418
- Giaimis J, Lombard Y, Fonteneau P, Muller CD, Levy R, Makaya-Kumba M, Lazdins J, Poindron P. Both mannose and beta-glucan receptors are involved in phagocytosis of unopsonized, heat killed Saccharomyces cerevisiae by murine macrophages. J Leukoc Biol 1993; 54: 564–571
- Grundemar L, Hakanson R. Multiple neuropeptide Y receptors are involved in cardiovascular regulation. Peripheral and central mechanisms. Gen Pharmacol 1993; 24: 785–796
- Hale KD, Ghanta VK, Gauthier DK, Hiramoto RN. Effects of rotational stress of different duration on NK cell activity, proinflammatory cytokines, and POMC-derived peptides in mice. Neuroimmunomodulation 2001; 9: 34–40
- Hauser GJ, Dayao EK, Zukowska-Grojec Z. Effect of Neuropeptide Y on endotoxin-induced suppression of the response to various agonists in conscious rats. Life Sci 1995; 57: 235–244
- Heijnen CJ, Kavelaars A, Ballieux RE. Beta-endorpin: Cytokine and neuropeptide. Immunol Rev 1991; 119: 42–63
- Hiremagalur B, Kvetnansky R, Nankova B, Fleicher J, Geertman R, Fukuhara K, Viskupic E, Sabban EL. Stress elicits trans-synaptic activation of adrenal neuropeptide Y gene expression. Brain Res Mol Brain Res 1994; 27: 138–144
- Johnson JD, O'Connor KA, Deak T, Spencer RL, Watkins LR, Maier SF. Prior stressor exposure primes the HPA axis. Psychoneuroendocrinology 2002; 27: 353–365
- Johnston RB, Kitagawa S. Molecular basis for the enhanced respiratory burst of activated macrophages. Fed Proc 1985; 44: 2927–2932
- Kizaki T, Oh-ishi S, Ohno H. Acute cold stress induces suppressor macrophages in mice. J Appl Physiol 1996; 81: 393–399
- Knutson B, Burgdof J, Panksepp J. Ultrasonic vocalizations as indices of affective states in rats. Psychol Bull 2002; 128: 961–977
- Le Cabec V, Cols C, Maridonneau-Parini I. Nonopsonic phagocytosis of zymosan and mycobacterium kansasii by CR3 (CD11b/CD18) involves distinct molecular determinants and is or is not coupled with NADPH oxidase activation. Infect Immun 2000; 68: 4734–4744
- Maier SF, Sherman JE, Lewis JW, Terman GW, Liebskind JC. The opioid/nonopioid nature of stress-induced analgesia and learned helplessness. J Exp Psychol Anim Behav Proc 1983; 9: 80–90
- McEwen BS. Protective and damaging effects of stress mediators: Allostasis and allostatic load. N Engl J Med 1998; 338: 171–179
- Melnicoff MJ, Horan PK, Morahan PS. Kinetics of changes in peritoneal cell population following acute inflammation. Cell Immunol 1989; 118: 178–191
- Mousa SA, Shakibaei M, Sitte N, Schäfer M, Stein C. Subcellular pathways of beta-endorphin synthesis, processing and release from immunocytes in inflammatory pain. Endocrinology 2004; 145: 1331–1334
- Moynihan JA, Karp JD, Cohen N, Ader R. Immune deviation following stress odor exposure: Role of endogenous opioids. J Immunol 2000; 102: 145–153
- Munck A, Guyre PM, Holbrook NJ. Physiological functions of glucocorticoids in stress and their relation to pharmacological actions. Endocr Rev 1984; 5: 25–55
- Nave H, Bedoui S, Moenter F, Steffens J, Felies M, Gebhardt T, Straub RH, Pabst R, Dimitrijević M, Stanojević S, von Hoersten S. Reduced tissue immigration of monocytes by neuropeptide Y during endotoxemia is associated with Y2 receptor activation. J Neuroimmunol 2004; 155: 1–12
- O'Connor KA, Johnson JD, Hammack SE, Brooks LM, Spencer RL, Watkins LR, Maier SF. Inescapable shock induces resistance to the effects of dexamethasone. Psychoneuroendocrinology 2003; 28: 481–500
- Oez S, Welte E, Platzer E, Kalden JR. A simple assay for quantifying the inducible adherence of neutrophils. Immunobiology 1990; 180: 308–315
- Ortega E, Forner MA, Barriga C. Effect of beta-endorphin on adherence, chemotaxis and phagocytosis of Candida Albicans by peritoneal macrophages. Comp Immunol Microbiol Infect Dis 1996; 19: 267–274
- Ottenweller NE, Natelson BJ, Pitman DL, Drastal SD. Adrenocortical and behavioural responses to repeated stressors: toward an animal model of chronic stress and stress-related mental illness. Bio Psychiatry 1989; 26: 829–841
- Piazza PV, Le Moal M. The role of stress in drug self-administration. Trends Pharmacol Sci 1998; 19: 67–74
- Pick E, Mizel D. Rapid microassays for the measurement of superoxide and hydrogen peroxide production by macrophages in culture using an automatic enzyme immunoassay reader. J Immunol Methods 1981; 46: 211–226
- Pick E, Charon J, Mizel DA. Rapid densitometric microassay for nitrobluetetrazolium reduction and application of the microassay to macrophages. J Reticuloend Soc 1981; 30: 581–593
- Puybasset L, Lacolley P, Laurent S, Mignon F, Billaus E, Cuche JL, Comoy E, Safar M. Effects of clonidine on plasma catecholamines and neuropeptide Y in hypertensive patients at rest and during stress. J Cardiovasc Pharmacol 1993; 21: 912–919
- Quan N, Avitsur R, Stark JL, He L, Lai W, Dhabhar F, Sheridan JF. Molecular mechanisms of glucocorticoid resistance in splenocytes of socially stressed male mice. J Neuroimmunol 2003; 137: 51–58
- Sacerdote P, Manfredi B, Bianchi M, Panerai AE. Intermittent but not continuous inescapable footshock stress affects immune responses and immunocyte beta-endorphin concentration in the rat. Brain Behav Immun 1994; 8: 251–260
- Schwarz H, Villiger PM, Kempis J, Lotz M. Neuropeptide Y is an inducible gene in the human immune system. J Neuroimmunol 1994; 51: 53–56
- Segura JJ, Guerrero JM, Pozo D, Calvo JR. Expression of vasoactive intestinal peptide binding sites in rat peritoneal macrophages is stimulated by inflammatory stimulus. J Neuroimmunol 1996; 64: 1–7
- Selbie LA, Darby K, Schmitz-Peiffer C, Browne CL, Herzog HD, Shine J, Biden TJ. Synergistic interaction of Y1-neuropeptide Y and alpha 1b-adrenergic receptors in the regulation of phospholipase C, protein kinase C, and arachidonic acid production. J Biol Chem 1995; 270: 11789–11796
- Shahabi NA, McAllen K, Sharp BM. Phosphorylation of activating transcription factor in murine splenocytes through delta opioid receptors. Cell Immunol 2003; 221: 122–127
- Shavit Y, Lewis W, Terman GW, Gale RP, Liebskind JC. Opioid peptides mediated the suppressive effect of stress on natural-killer cell cytotoxicity. Science 1984; 223: 188–190
- Smith AI, Funder JW. Proopioimelantocortin processing in the pituitary, central nervous system, and peripheral tissues. Endocr Rev 1988; 9: 159–179
- Stanojević S, Dimitrijević M, Kovačević-Jovanović V, Miletić T, Vujić V, Radulović J. Stress applied during primary immunization affects the secondary humoral immune response in the rat: Involvement of opioid peptides. Stress 2003; 6: 247–258
- Stanojević S, Mitić K, Vujić V, Kovačević-Jovanović V, Dimitrijević M. β-Endorphin differentially affects inflammation in two inbred rat strains. Eur J Pharmacol 2006; 549: 157–165
- Stevens-Felten SY, Bellinger DL. Noradrenergic and peptidergic innervation of lymphoid organs. Chem Immunol 1997; 69: 99–131
- Stratakis CA, Chrousos GP. Neuroendocrinology and pathophysiology of the stress system. Stress: Basic mechanisms and clinical implications, CP Chrousos, R McCarty, K Pacak, G Cizza, E Sternberg, P Gold, R Kvetnansky. Annals of the New York Academy of Sciences, New York 1995; Vol 771: 1–19
- Sung CP, Arleth AJ, Feuerstein GZ. Neuropeptide Y upregulates the adhesiveness of human endothelial cells for leukocytes. Circ Res 1991; 68: 314–318
- Sutton LC, Fleshner M, Mazzeo R, Maier SF, Watkins LR. A permissive role of corticosterone in an opioid form of stress-induced analgesia: Blockade of opiate analgesia is not due to stress-induced hormone release. Brain Res 1994; 663: 19–29
- Tilders FJ, Schmidt ED. Cross-sensitization between immune and non-immune stressors. A role in the ethiology of depression?. Adv Exp Med Biol 1999; 461: 179–197
- Tsukada F, Ohuchi Y, Terunuma T, Sugawara M, Kohno H, Ohkubo Y. Activation of μ-opioid patway is associated with the canceling effect of footschock stimulus on the restraint stress-induced inhibition of small intestinal motility in rats. Biol Pharm Bull 2001; 24: 1332–1334
- Wheway J, Mackay CR, Newton RA, Sainsbury A, Boey D, Herzog H, Mackay F. A fundamental bimodal role for neuropeptide Y1 receptor in the immune system. J Exp Med 2005; 202: 1527–1538
- Whitnall MH. Regulation of the hypopthalamic corticotropin-releasing hormone neurosecretory system. Prog Neurobiol 1993; 40: 573–629
- Wilckens T. Glucocorticoids and immune function: Physiological relevance and pathogenic potential of hormonal dysfunction. TiPS 1995; 16: 193–197
- Wilckens T, De Rijk R. Glucocorticoids and immune function: Unknown dimensions and new frontiers. Immunol Today 1997; 18: 418–423