Abstract
The contribution of growth hormone (GH), released during acute and repeated stressful situations, to the development of stress-related disorders is often neglected. We have hypothesized that the modulation of the GH response to sequential stress exposure in humans depends mainly on the nature of the stressor. To test this hypothesis, we compared GH responses to different stressful situations, namely aerobic exercise, hypoglycemia and hyperthermia, which were applied in two sequential sessions separated by 80–150 min. In addition, administration of the dopaminergic drug apomorphine was used as a pharmacological stimulus. GH responses to submaximal exercise (bicycle ergometer, increasing work loads of 1.5, 2.0 and 2.5 W/kg, total duration 20 min) and hyperthermia in a sauna (80°C, 30 min) were prevented when preceded by the same stress stimulus. Hypoglycemia induced by insulin (0.1 IU/kg intravenously) resulted in a significant GH response also during the second of the two consecutive insulin tests, though the response was reduced. Administration of apomorphine (0.75 mg subcutaneously) or insulin prevented the increase in GH release in response to a sequential bolus of apomorphine, while hypoglycemia induced a significant elevation in GH levels even if applied after a previous treatment with apomorphine. In conclusion, the feedback inhibition of the GH response to a sequential stress stimulus depends on the stimulus used. Unlike in the case of exercise and hyperthermia, mechanisms involved in the stress response to hypoglycemia appear to overcome the usual feedback mechanisms and to re-induce the GH response when applied after another stimulus.
Introduction
For a long time, pituitary growth hormone (GH) has been known to be released during stressful situations in humans (Glick et al. Citation1965; Jezova-Repcekova et al. Citation1979). Nevertheless, the involvement of GH in stress-induced physiological changes and in relation to the consequences of repeated stress exposure in the development of stress-related somatic and mental disorders is often neglected. Negative consequences of chronic stress are mainly attributed to changes in the hypothalamic–pituitary–adrenocortical axis and to enhanced sympathetic-adrenomedullary activation.
Several recent advances in experimental and practical medicine argue for renewal of the interest in GH release during stress. Stress exposure has been found to modulate brain plasticity thus increasing vulnerability to mental and neurodegenerative disorders (Kim et al. Citation2006). GH might evoke neuroprotective effects and has been found to affect cognition and the biochemistry of the adult brain. GH is the main regulator of insulin-like growth factor-I (IGF-I), which functions as a putative regenerative agent (Arwert et al. Citation2005; Aberg et al. Citation2006; Bondanelli et al. Citation2006). Stress is known to contribute to the development of depression and anxiety disorders (Jezova Citation2005) and a higher prevalence of mental disorders has been observed in patients with GH deficiency (Bulow et al. Citation2002). Nowadays, increased attention is given to adult GH deficiency and GH replacement therapy has been shown to reverse many of the associated abnormalities including improved mood and quality of life (Deijen and Arwert Citation2006). Moreover, GH belongs to the group of immunostimulatory hormones (Meazza et al. Citation2004). Increased concentrations of GH in plasma may play an important role in counteracting immunosuppressive effects of glucocorticoids during stress.
The secretion of GH is under the influence of hypothalamic GH-releasing hormone (GH-RH) and somatostatin. Ghrelin and probably other GH-releasing peptides contribute to GH release, which is known to be pulsatile. GH regulates its own secretion via a negative autofeedback, which might operate at both the anterior pituitary and the hypothalamic level. Increased GH concentrations evoke prompt hypothalamic somatostatin release, which in turn inhibits GH-RH secretion, blocks pituitary exocytosis of GH and sensitizes somatotropes to the next GH-RH stimulus (Veldhuis et al. Citation2005). Thus, the activation of GH release by a stress stimulus is likely to modulate GH responses to sequential stimuli. Indeed, previous studies using mainly exercise stress or pharmacological stimulation have shown that GH responses are influenced by a previous stimulation but the results were equivocal, showing both attenuation and augmentation of hormone responses (Cappon et al. Citation1994; Kanaley et al. Citation1997; Ronsen et al. Citation2001; Stokes et al. Citation2002). Recent studies have shown that GH responses to sequential bouts of exercise are dependent on the time interval between the exercise sessions (Sartorio et al. Citation2005; Stokes et al. Citation2005).
We hypothesized that the modulation of the GH response to a sequential stress exposure in humans depends mainly on the nature of the stress situation and the nature of the stressor. To test this hypothesis, we have compared GH responses of healthy volunteers to three different stress situations, namely aerobic exercise, insulin-induced hypoglycaemia and hyperthermia in a sauna, which were applied in two consecutive sessions. In the attempt to approach the mechanisms involved, GH release was investigated also following exposure to dopamine receptor stimulation by apomorphine and its combination with insulin, as well as to a GHRH bolus applied under conditions of increased baseline concentrations of GH.
Subjects and methods
Subjects
Twenty eight healthy male volunteers aged 23–30 years with BMI < 25 kg/m2 participated in the study. Exclusion criteria were somatic or mental diseases, smoking or taking any medication. The subjects were university students with good physical fitness but not participating in any competitive sport. The subjects were asked to avoid intense physical activity the day before and particularly in the morning before the investigation. All investigations were carried out in the morning starting at 7.30 h, after an overnight fast. Upon arrival, the subjects rested in the supine position and an indwelling catheter was inserted into an antecubital vein. After a 30 min rest, baseline blood samples were taken. Thereafter the subjects were exposed to the stress stimulus or pharmacological treatment. A limitation of the present study is that the group of subjects participating in each trial was not the same. Each volunteer participated in 1–4 out of 7 different randomized trials separated at least by one week. The studies were conducted in accordance with the Declaration of Helsinki. The protocol was approved by the Ethical Committee of the Slovak Academy of Sciences and after explanation the subjects gave their consent to participate.
Stress stimuli and pharmacological treatments
As the stressful stimuli, exercise, insulin-induced hypoglycaemia and hyperthermia in a sauna were used. Pharmacological stimuli included apomorphine or GHRH administration. During each trial, two stimuli were applied in a sequence, separated by 120–150 min. The exception was the trial with two sequential bouts of exercise, separated by 80 min.
Exercise+Exercise (n = 8). The bicycle ergometer exercise test was performed with increasing work loads of 1.5, 2.0 and 2.5 W/kg. Each work load lasted 6 min with a 1 min rest between loads. Blood samples were taken immediately following the last load. The subjects were thereafter seated comfortably and the blood samples were drawn at 10, 30 and 60 min of rest. The second exercise test with the same work load and blood sampling protocol was initiated 80 min following the start of the first test.
Insulin+Insulin (n = 9). Hypoglycaemia was induced by intravenous (i.v.) bolus injection of insulin (Actrapid, Novo Nordisk, Denmark) at a dose of 0.1 IU/kg body weight. Blood samples were obtained at 30, 45, 60, 90 and 120 min. Following the sample at 150 min, a second bolus of insulin was injected at the same dose and the blood sampling continued for another 120 min.
Sauna+Sauna (n = 8). The subjects were seated in a sauna room (80°C) for 30 min followed by bed rest at room temperature without cooling in cold water. Blood samples were taken at 30 min intervals and the second exposure to the sauna for 30 min started at 120 min after the start of the first exposure.
Apomorphine+Apomorphine (n = 8). The dopamine receptor agonist apomophine (apomorphine hydrochloride, Apomorphin Spofa, Prague, Czech Republic) was injected subcutaneously (s.c.) at the dose of 0.75 mg. Blood sampling was performed at 15 min intervals up to 120 min, when the second injection of apomorphine at the same dose was administered. During the whole procedure, the subjects sat in an armchair.
Apomorphine+Insulin (n = 8). Hypoglycemia was induced by an i.v. insulin bolus 120 min following s.c. apomorphine injection at doses and with blood sampling as described above.
Insulin+Apomorphine (n = 9). In this trial, apomorphine was injected 150 min after an insulin bolus. The doses and blood sampling were as described above.
GHRH test (n = 7). Synthetic GHRH (Kabi-Pharmacia, Stockholm, Sweden) was injected i.v. at a dose of 1 μg/kg body weight. The test was performed twice, namely at room temperature (22°C) and at increased (55°C) ambient temperature (dry heat). The ambient temperature was selected to increase body temperature by less than 1°C (Jezova et al. Citation1998). The subjects were exposed to normal or increased ambient temperature for 45 min before GHRH administration. The tests were separated by at least 7 days.
Measurements
Blood samples (5 ml) containing heparin as anticoagulant were immediately cooled on ice and centrifuged at 4°C. Aliquots of plasma were kept frozen until analyzed. GH was measured using commercial radioimmunoassay kits (Immunotech, Prague, Czech Republic). All samples from one trial were analyzed in one assay. Intra-assay coefficient of variation was below 5% and the inter-assay coefficient of variation was below 14% with analytical sensitivity of 0.6 ng/ml.
Statistical analysis
Group values are presented as means ± SEM Data were analyzed by two-way repeated measures ANOVA with time and treatment (the 1st vs. 2nd stimulus applied) as factors. ANOVA was followed by Student-Newman–Keuls post hoc tests for pairwise multiple comparisons, when appropriate.
Results
Statistical analysis of plasma GH concentrations in response to two consecutive exercise bouts showed significant main effects for factors time (F = 9.00, p < 0.001), treatment (F = 9.03, p < 0.05) as well as for treatment × time interaction (F = 9.72, p < 0.001). In response to the first exercise bout, GH release was increased at all time intervals (p < 0.001) and remained elevated at 80 min (p < 0.05), when the 2nd exercise started. No elevations of GH levels above the pre-exercise values were found in response to the 2nd exercise test (). GH levels in response to the 2nd exercise test were significantly lower compared to those in response to the 1st exercise at 30 and 50 min (p < 0.001).
Figure 1 Growth hormone response to two consecutive stress stimuli: (A) exercise followed by exercise (n = 8), (B) insulin-induced hypoglycemia followed by insulin-induced hypoglycemia (n = 9), and (C) hyperthermia followed by hyperthermia (n = 8). The vertical bars (1A) symbolize the work loads during the bicycle ergometer exercise. The horizontal bars (1C) represent the exposure to a sauna. Two way RM ANOVA results are listed in the graphs. NS not significant, *p < 0.05, **p < 0.01 and ***p < 0.001 for timepoint vs. the respective baseline (start of the stimulus) values.

Plasma GH concentrations increased in response to insulin-induced hypoglycemia. There was a significant main effect of time (F = 16.76, p < 0.001), no effect of treatment but a significant treatment × time interaction (F = 11.01, p < 0.001). During the first insulin test, GH release was increased at all time intervals starting at 45 min (p < 0.001) and remained elevated at 150 min (p < 0.05), when the 2nd insulin treatment started. During the 2nd insulin test, plasma GH concentrations increased significantly (p < 0.001) compared to those before the 2nd insulin injection at 60 min (). GH levels in response to the 2nd insulin test were significantly lower compared to those in response to the 1st insulin test at 90 and 120 min (p < 0.05). As to the stress stimulus itself, the hypoglycemia induced by the 2nd insulin injection was slightly less pronounced at 30 and 90 (p ≤ 0.05) min ().
Table I. Plasma glucose concentrations during two sequential insulin boluses (0.1 IU/kg i.v.) separated by 150 min.
The analysis of plasma GH concentrations in response to two sequential exposures to hyperthermia in a sauna showed significant main effects for factors time (F = 17.56, p < 0.001), treatment (F = 14.47, p < 0.01) as well as for treatment × time interaction (F = 12.77, p < 0.001). In response to the first sauna bath, GH release was increased at all time intervals (p < 0.001) up to 90 min (p < 0.01). GH concentrations had returned to control values at 120 min, when the 2nd sauna exposure started. No elevations of GH levels above the values before the sauna bath were found in response to the 2nd sauna exposure (). GH levels in response to the 2nd stay in sauna were significantly lower compared to those in response to the 1st sauna exposure at 30 and 60 min (p < 0.001).
Following two consecutive apomorphine injections, statistical analysis of plasma GH concentrations showed significant main effects for factors time (F = 11.79, p < 0.001), treatment (F = 18.11, p < 0.01) as well as for treatment × time interaction (F = 7.90, p < 0.001). In response to the first apomorphine injection, GH release was increased at all time intervals (p < 0.001) starting at 30 min and returned to baseline levels at 90 min. Thus, GH concentrations were low at 120 min, when the 2nd apomorphine injection was administered. No elevations of GH levels above the control values were found in response to the 2nd apomorphine treatment (). GH levels in response to the 2nd injection of apomorphine were significantly lower compared to those in response to the 1st injection at 15 (p < 0.05), 30, 45, 60 (p < 0.001) and 75 min (p < 0.01).
Figure 2 Growth hormone response to two consecutive stimuli: (A) apomorphine administration followed by apomorphine administration (n = 8), (B) insulin-induced hypoglycemia followed by apomorphine administration (n = 9), and (C) apomorphine administration followed by insulin-induced hypoglycemia (n = 8). Two way RM ANOVA results are listed in the graphs. NS not significant, *p < 0.05, **p < 0.01 and ***p < 0.001 for time-point vs. the respective baseline (start of the stimulus) values.
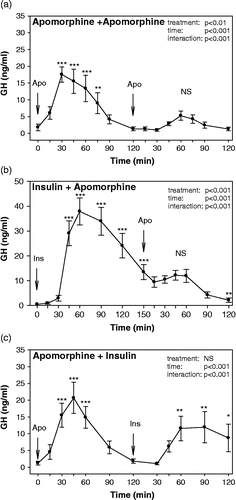
Apomorphine administration failed to induce GH release if preceded by an insulin-induced hypoglycemia. The analysis of GH levels in response to insulin-induced hypoglycemia followed by apomorphine injection showed significant main effects for factors time (F = 20.22, p < 0.001), treatment (F = 37.88, p < 0.001) as well as for treatment × time interaction (F = 27.34, p < 0.001). In response to hypoglycemia, GH release was increased at all time intervals starting at 45 min (p < 0.001) and remained elevated at 150 min (p < 0.001), when the injection of apomorphine was performed. No elevations of GH levels above the pre-injection values were found in response to apomorphine treatment (). At 120 min after apomorphine, GH levels were even significantly lower (p < 0.01) compared to those before the injection.
Insulin-induced hypoglycemia evoked GH release even if preceded by administration of apomorphine. The analysis of GH levels in response to apomorphine injection followed by the insulin test showed significant main effects for factors time (F = 10.79, p < 0.001), and for treatment × time interaction (F = 10.67, p < 0.001). In response to apomorphine injection, GH release was increased at all time intervals (p < 0.001) starting at 30 min and returned to baseline levels at 90 min. Thus, GH concentrations were low at 120 min, when the insulin test started. During the insulin test, GH levels increased significantly (p < 0.01) compared to those before insulin injection at 60 and 90 min ().
On two different days, GHRH tests were performed under conditions of normal and increased ambient temperature with the aim to increase basal GH levels. Statistical analysis showed significant main effects for factors time (F = 49.01, p < 0.001), treatment (F = 6.17, p < 0.05) as well as for treatment × time interaction (F = 2.56, p < 0.05). Under normal ambient temperature, GH release was increased at 15, 30, 45 and 60 min (p < 0.001). Under increased ambient temperature, GH levels increased significantly (p < 0.001) at the same time intervals as during the test under normal temperature (). GH levels before the test were enhanced (p = 0.052) by the previous 45 min exposure to the increased environmental temperature. Plasma concentrations of GH during the GHRH test performed at increased ambient temperature were significantly higher compared to those obtained after a GHRH bolus under normal temperature at 15, 45, 60 and 30 min (p < 0.05 and p < 0.01, respectively). Exposure to increased ambient temperature resulted in no significant change in body temperature (data not shown).
Figure 3 Growth hormone response to GHRH administration under conditions associated with normal (room temperature, 22°C closed circles) and elevated (increased ambient temperature, 55°C open circles) baseline GH levels (n = 7). The horizontal bar represents the exposure to increased ambient temperature. Two way RM ANOVA results are listed in the graphs. *p < 0.05, **p < 0.01 and ***p < 0.001 for time-point vs. baseline (GHRH administration),+p < 0.05 and ++p < 0.01 room temperature vs. increased ambient temperature.
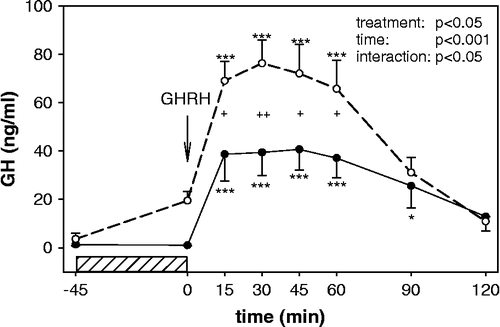
Discussion
The present study shows that the GH response to exercise and hyperthermia in healthy men can be prevented when preceded by the same stress stimulus applied 80–120 min earlier. On the other hand, hypoglycemia still induced significant—albeit diminished—GH responses during the second of two consecutive insulin stimuli. Administration of the dopaminergic drug apomorphine or insulin prevented the increase in GH release in response to a subsequent bolus of apomorphine, while hypoglycemia induced significant elevation in GH levels even if applied after previous treatment with apomorphine. Thus, the stress of hypoglycemia seems to involve different mechanisms related to the control of GH release compared to other stressors such as hyperthermia and exercise. These findings support the hypothesis that the modulation of GH responses to a sequential stress exposure in humans depends mainly on the nature of the stress stimulus.
The abolition of a GH response to the second bout of exercise observed in this study is in agreement with the results of Stokes et al. (Citation2005) who used two short sprints on a cycle ergometer separated by 60 min. These authors studied different recovery periods between the bouts of exercise and concluded that repeated bouts of sprint cycling on the same day resulted in an attenuation or even ablation of the exercise-induced increase in GH, depending on the recovery interval between sprints. In this study, similar results were obtained with repeated exposure to the sauna. Hyperthermia is a well known stimulus to GH release, reflecting activation of one of the hormonal systems responding to experiencing a sauna (Vigas et al. Citation1984; Leppaluoto et al. Citation1986; Kukkonen-Harjula and Kauppinen Citation1988). Previous studies on endocrine changes during repeated sauna exposure are scarce. Leppaluoto et al. (Citation1986) demonstrated a decline in GH response to hyperthermia on the third day of repeated exposure to sauna bathing. There are no studies evaluating hormone responses to repeated sauna exposure separated by short time intervals. Results of the present study show that there was no significant GH response to hyperthermia during a second sauna exposure just 120 min after the first. Thus, the pattern of GH response to repeated sauna exposure and repeated exercise were similar. These two stressful situations appear to operate via similar mechanisms resulting in an inhibition of GH release. It was shown previously (Ghigo et al. Citation1989) that the GH response to the second of two consecutive GHRH boluses (separated by 120 min) is markedly inhibited. It seems likely that exercise and hyperthermia induce GHRH release and somatotrope unresponsiveness to the repeated exposure due to subsequent activation of somatostatin secretion.
The data presented suggest that more complex mechanisms are associated with repeated hypoglycemia. Changes in GH release in response to hypoglycemia below the “glycemic threshold” are solely due to central signaling of glucosensitive neurons in the ventromedial hypothalamus (Vigas et al. Citation1990; Borg et al. Citation1997). GH responses could result either from GHRH release or from acute decline in somatostatin secretion. It is still not clear which hypothalamic hormone mainly participates in hypoglycemia-induced GH secretion. Activation of GHRH secretion is required for a full GH response to hypoglycemia, as demonstrated for example by administration of a GHRH antagonist (Jaffe et al. Citation1996). The possibility that stimulation of GH secretion during hypoglycemia is not mediated solely by GHRH has considered previously (Wakabayashi et al. Citation1986). Indeed, several studies indicate that hypoglycemia stimulates GH secretion through a reduction in somatostatin release (Shibasaki et al. Citation1985; Vance et al. Citation1986; Cordido et al. Citation1990; Hanew and Utsumi Citation2002).
Unlike other stressors used in this study, insulin-induced hypoglycemia resulted in a significant GH response even if the insulin bolus was repeated. We therefore evaluated GH responses to different combinations of insulin and/or apomorphine, a dopaminergic agonist known to induce GH release (Jezova and Vigas Citation1988) mediated via activation of GHRH secretion (Brambilla et al. Citation1997). Consistent with known feedback mechanisms (Muller et al. Citation1999), there was a lack of GH response to the second of two consecutive administrations of apomorphine. The GH response to apomorphine injection was abolished also by prior hypoglycemia suggesting a sufficient induction of feedback mechanisms. However, apomorphine failed to inhibit GH response to sequential hypoglycemia. These findings are consistent with the results of Shibasaki et al. (Citation1985), who used direct GHRH administration. They demonstrated a GH response to hypoglycemia after a GHRH bolus and a lack of response when GHRH was administered after insulin. The same group of authors showed that GH secretion caused by insulin-induced hypoglycemia was reduced but still present after administration of a somatostatin analog (Masuda et al. Citation1990). Prolonged hypoglycemia was found to be accompanied by sustained elevation of GH levels (Barbetti et al. Citation1990). Thus, the present study provides additional evidence that some factor/s other than GHRH and somatostatin are involved in the activation of GH release during the stress of hypoglycemia. As for other factors, ghrelin is not likely to play any regulatory role in GH response to hypoglycemia (Flanagan et al. Citation2003; Broglio et al. Citation2004; Huber et al. Citation2007).
It may be argued that GH levels just before the second insulin and the exercise tests were increased, which might have influenced hormone responses to the second stimulus. To approach this problem, we performed a GHRH test under conditions associated with elevated baseline GH levels before the test. Elevation of GH levels was induced by increasing the ambient temperature. For comparison, the GHRH test was performed also at room temperature and the tests were separated by 7 days. The results show that the GH response to a GHRH bolus at increased ambient temperature was even greater than that at normal room temperature and lower basal GH levels. This is consistent with the results of our previous study (Jezova et al. Citation1998) showing that increased basal plasma GH concentrations induced in healthy subjects at increased ambient temperature did not reduce the GH response to hypoglycemia.
In conclusion, the feedback inhibition of the GH response to a sequential stress stimulus applied at relatively short time intervals (80–150 min) is dependent on the nature of the stressor. Repeated exposure to stress stimuli involving both central and peripheral signaling pathways (Kjaer et al. Citation1989; Koska et al. Citation2003), namely exercise and hyperthermia, results in a lack of a GH response to a second homeotypic stimulus. On the other hand, the GH response to hypoglycemia, which triggers GH release via central mechanisms, is not attenuated by previous exposure to the same stressor or to dopaminergic activation by apomorphine injection. We suggest that factor/s other than GHRH and somatostatin participate in the mediation of GH release during the stress of hypoglycemia. Unlike in the case of exercise and hyperthermia, mechanisms involved in the stress response to hypoglycemia appear to overcome usual feedback mechanisms and to re-induce the GH response when applied after another stimulus.
Acknowledgements
This study was supported by grants of VEGA 5064, 6157 and European Social Fund. We thank the colleagues Dr M. Vlcek and Dr K. Ondicova for their kind help.
References
- Aberg ND, Brywe KG, Isgaard J. Aspects of growth hormone and insulin-like growth factor-I related to neuroprotection, regeneration, and functional plasticity in the adult brain. Sci World J 2006; 6: 53–80
- Arwert LI, Veltman DJ, Deijen JB, van Dam PS, Delemarre-van deWaal HA, Drent ML. Growth hormone deficiency and memory functioning in adults visualized by functional magnetic resonance imaging. Neuroendocrinology 2005; 82: 32–40
- Barbetti F, Crescenti C, Negri M, Leonetti F, Grossi A, Tamburrano G. Growth hormone does not inhibit its own secretion during prolonged hypoglycemia in man. J Clin Endocrinol Metab 1990; 70: 1371–1374
- Bondanelli M, Ambrosio MR, Onofri A, Bergonzoni A, Lavezzi S, Zatelli MC, Valle D, Basaglia N, Degli Uberti EC. Predictive value of circulating insulin-like growth factor I levels in Ischemic stroke outcome. J Clin Endocrinol Metab 2006; 91: 3928–3934
- Borg MA, Sherwin RS, Borg WP, Tamborlane WV, Shulman GI. Local ventromedial hypothalamus glucose perfusion blocks counterregulation during systemic hypoglycemia in awake rats. J Clin Invest 1997; 99: 361–365
- Brambilla F, Bellodi L, Perna G, Arancio C, Bertani A. Dopamine function in obsessive-compulsive disorder: Growth hormone response to apomorphine stimulation. Biol Psychiatry 1997; 42: 889–897
- Broglio F, Gottero C, Prodam F, Destefanis S, Gauna C, Me E, Riganti F, Vivenza D, Rapa A, Martina V, Arvat E, Bona G, van der Lely AJ, Ghigo E. Ghrelin secretion is inhibited by glucose load and insulin-induced hypoglycaemia but unaffected by glucagon and arginine in humans. Clin Endocrinol 2004; 61: 503–509
- Bulow B, Hagmar L, Orbaek P, Osterberg K, Erfurth EM. High incidence of mental disorders, reduced mental well-being and cognitive function in hypopituitary women with GH deficiency treated for pituitary disease. Clin Endocrinol 2002; 56: 183–193
- Cappon J, Brasel JA, Mohan S, Cooper DM. Effect of brief exercise on circulating insulin-like growth factor I. J Appl Physiol 1994; 76: 2490–2496
- Cordido F, Dieguez C, Casanueva FF. Effect of central cholinergic neurotransmission enhancement by pyridostigmine on the growth hormone secretion elicited by clonidine, arginine, or hypoglycemia in normal and obese subjects. J Clin Endocrinol Metab 1990; 70: 1361–1370
- Deijen JB, Arwert LI. Impaired quality of life in hypopituitary adults with growth hormone deficiency: Can somatropin replacement therapy help?. Treat Endocrinol 2006; 5: 243–250
- Flanagan DE, Evans ML, Monsod TP, Rife F, Heptulla RA, Tamborlane WV, Sherwin RS. The influence of insulin on circulating ghrelin. Am J Physiol Endocrinol Metab 2003; 284: 313–316
- Ghigo E, Goffi S, Mazza E, Arvat E, Procopio M, Bellone J, Muller EE, Camanni F. Repeated GH-releasing hormone administration unravels different GH secretory patterns in normal adults and children. Acta Endocrinol 1989; 120: 598–601
- Glick SM, Roth J, Yalow RS, Berson SA. The regulation of growth hormone secretion. Recent Prog Horm Res 1965; 21: 241–283
- Hanew K, Utsumi A. The role of endogenous GHRH in arginine-, insulin-, clonidine- and l-dopa-induced GH release in normal subjects. Eur J Endocrinol 2002; 146: 197–202
- Huber J, Reiterer EE, Sudi K, Gallistl S, Friedl K, Weinhandl G, Aigner R, Borkenstein MH. Ghrelin does not regulate the GH response to insulin-induced hypoglycaemia in children but could be involved in the regulation of cortisol secretion. Clin Endocrinol (Oxf) 2007; 66: 143–147
- Jaffe CA, DeMott-Friberg R, Barkan AL. Endogenous growth hormone (GH)-releasing hormone is required for GH responses to pharmacological stimuli. J Clin Invest 1996; 97: 934–940
- Jezova D. Control of ACTH secretion by excitatory amino acids: Functional significance and clinical implications. Endocrine 2005; 28: 287–294
- Jezova D, Vigas M. Apomorphine injection stimulates beta-endorphin, adrenocorticotropin, and cortisol release in healthy man. Psychoneuroendocrinology 1988; 13: 479–485
- Jezova-Repcekova D, Klimes I, Jurcovicova J, Vigas M. Effect of adrenergic receptor blockade on cortisol and GH response to insulin-induced hypoglycemia in man. Int J Clin Pharmacol Biopharm 1979; 17: 64–67
- Jezova D, Kvetnansky R, Nazar K, Vigas M. Enhanced neuroendocrine response to insulin tolerance test performed under increased ambient temperature. J Endocrinol Invest 1998; 21: 412–417
- Kanaley JA, Weltman JY, Veldhuis JD, Rogol AD, Hartman ML, Weltman A. Human growth hormone response to repeated bouts of aerobic exercise. J Appl Physiol 1997; 83: 1756–1761
- Kim JJ, Song EY, Kosten TA. Stress effects in the hippocampus: Synaptic plasticity and memory. Stress 2006; 9: 1–11
- Kjaer M, Secher NH, Bach FW, Sheikh S, Galbo H. Hormonal and metabolic responses to exercise in humans: Effect of sensory nervous blockade. Am J Physiol 1989; 257: 95–101
- Koska J, Rovensky J, Zimanova T, Vigas M. Growth hormone and prolactin responses during partial and whole body warm-water immersions. Acta Physiol Scand 2003; 178: 19–23
- Kukkonen-Harjula K, Kauppinen K. How the sauna affects the endocrine system. Ann Clin Res 1988; 20: 262–266
- Leppaluoto J, Huttunen P, Hirvonen J, Vaananen A, Tuominen M, Vuori J. Endocrine effects of repeated sauna bathing. Acta Physiol Scand 1986; 28: 467–470
- Masuda A, Shibasaki T, Hotta M, Yamauchi N, Ling N, Demura H, Shizume K. Insulin-induced hypoglycemia, l-dopa and arginine stimulate GH secretion through different mechanisms in man. Regul Pept 1990; 31: 53–64
- Meazza C, Pagani S, Travaglino P, Bozzola M. Effect of growth hormone (GH) on the immune system. Pediatr Endocrinol Rev 2004; 1: 490–495
- Muller EE, Locatelli V, Cocchi D. Neuroendocrine control of growth hormone secretion. Physiol Rev 1999; 79: 511–607
- Ronsen O, Haug E, Pedersen BK, Bahr R. Increased neuroendocrine response to a repeated bout of endurance exercise. Med Sci Sports Exerc 2001; 33: 568–575
- Sartorio A, Agosti F, Marinone PG, Proietti M, Lafortuna CL. Growth hormone responses to repeated bouts of aerobic exercise with different recovery intervals in cyclists. J Endocrinol Invest 2005; 28: 11–14
- Shibasaki T, Hotta M, Masuda A, Imaki T, Obara N, Demura H, Ling N, Shizume K. Plasma GH responses to GHRH and insulin-induced hypoglycemia in man. J Clin Endocrinol Metab 1985; 60: 1265–1267
- Stokes KA, Nevill ME, Hall GM, Lakomy HK. Growth hormone responses to repeated maximal cycle ergometer exercise at different pedaling rates. J Appl Physiol 2002; 92: 602–608
- Stokes K, Nevill M, Frystyk J, Lakomy H, Hall G. Human growth hormone responses to repeated bouts of sprint exercise with different recovery periods between bouts. J Appl Physiol 2005; 99: 1254–1261
- Vance ML, Kaiser DL, Rivier J, Vale W, Thorner MO. Dual effects of growth hormone (GH)-releasing hormone infusion in normal men: Somatotroph desensitization and increase in releasable GH. J Clin Endocrinol Metab 1986; 62: 91–94
- Veldhuis JD, Iranmanesh A, Bowers CY. Joint mechanisms of impaired growth-hormone pulse renewal in aging men. J Clin Endocrinol Metab 2005; 90: 4177–4183
- Vigas M, Jurcovicova J, Jezova D, Kvetnansky R, Palat M. Endocrine reactions during hyperthermia in healthy persons. Bratisl Lek Listy 1984; 82: 1041–1048
- Vigas M, Tatar P, Jurcovicova J, Jezova D. Glucoreceptors located in different areas mediate the hypoglycemia-induced release of growth hormone, prolactin, and adrenocorticotropin in man. Neuroendocrinology 1990; 51: 365–368
- Wakabayashi I, Shibasaki T, Ling N. A divergence of plasma growth hormone response between growth hormone-releasing factor and insulin-induced hypoglycaemia among middle-aged healthy male subjects. Clin Endocrinol 1986; 24: 279–283