Abstract
Angiotensin II (Ang II), the active principle of the renin-angiotensin system (RAS), was discovered as a vasoconstrictive, fluid retentive circulating hormone. It was revealed later that there are local RAS in many organs, including the brain. The physiological receptor for Ang II, the AT1 receptor type, was found to be highly expressed in many tissues and brain areas involved in the hypothalamic-pituitary-adrenal axis response to stress and in the sympathoadrenal system. The production of circulating and local Ang II, and the expression of AT1 receptors increase during stress. Blockade of peripheral and brain AT1 receptors with receptor antagonists administered peripherally prevented the hormonal and sympathoadrenal response to isolation stress, the stress-related alterations in cortical CRF1 and benzodiazepine receptors, part of the GABAA complex, and reduced anxiety in rodents. AT1 receptor blockade prevented the ulcerations of the gastric mucosa produced by cold-restraint stress, by preservation of the gastric blood flow, prevention of the stress-induced inflammatory response of the gastric mucosa, and partial blockade of the sympathoadrenal response to the stress. Our observations demonstrate that Ang II is an important stress hormone, and that blockade of AT1 receptors could be proposed as a potentially useful therapy for stress-induced disorders.
Introduction
This review is focused on the role of brain and peripheral angiotensin II (Ang II) in stress. A participation of central and peripheral renin-angiotensin systems (RAS) in stress first emerged from studies on Ang II receptor localization (Tsutsumi and Saavedra Citation1991a) and from the finding of increased Ang II formation and receptor expression during stress (Castrén and Saavedra Citation1988; Xang et al. Citation1993; Yang et al. Citation1996). This hypothesis was firmly established with the demonstration that inhibition of Ang II receptors prevented not only the hormonal and sympathoadrenal response to stress (Armando et al. Citation2001) but also the response of higher cortical centers, reducing anxiety in rodents (Saavedra et al. Citation2006).
Circulating and local renin-angiotensin systems
Ang II, the active principle of the RAS, was originally described as a peripheral hormone (Page and Helmer Citation1940; Braun-Menéndez et al. Citation1940) involved in the regulation of blood pressure and fluid metabolism, inducing vasoconstriction, aldosterone release, sodium and water retention, and increasing fluid intake (Page Citation1987). For many years, research on the RAS was focused on these initial findings, and on the role of Ang II in cardiovascular and kidney function (Page Citation1987). Further research demonstrated an increasing complexity of the RAS. The concept of local RAS began to emerge, with local systems expressed in most organs, including the brain and functioning both in coordination and independently of the circulating RAS (Saavedra Citation1992; Paul et al. Citation2006). The recognition that most organs were capable of producing Ang II, and many tissues expressed Ang II receptors accessible to both circulating and locally formed peptide, led to the postulate that Ang II is a multitasking peptide involved in numerous regulatory functions throughout the body, including the peripheral and central response to stress (Saavedra Citation2005; Saavedra et al. Citation2005).
Angiotensinergic pathways in the brain related to the control of the stress axis
With the use of quantitative autoradiography for the localization of Ang II receptors, specific antibodies to detect the presence of Ang II, and electrophysiological methods to determine the response to Ang II of neurons localized to selective brain areas, it is now possible to demonstrate a series of brain Ang II pathways that contribute to regulate the reaction to stress. Receptors for Ang II are expressed in large quantities in all circumventricular organs including the subfornical organ (SFO) and the median eminence, in the hypothalamic paraventricular nucleus (PVN), amygdaloidal and septal nuclei, the hippocampus and the nucleus of the solitary tract (Tsutsumi and Saavedra Citation1991a,Citationb). In these areas, all Ang II receptors are of the AT1 type (Tsutsumi and Saavedra Citation1991a,Citationb). In the rat, the locus coeruleus, producing most of the forebrain norepinephrine, expresses AT2 receptors (Tsutsumi and Saavedra Citation1991a,Citationb).
The scope of the present review does not allow us to analyze in detail all Ang II pathways related to stress. The best studied of such pathways is the SFO–PVN connection (Bains and Ferguson Citation1995). Such a pathway connects neurons outside the blood brain barrier, in the SFO, with magnocellular and parvocellular, putative CRF, neurons in the PVN and via actions on dorsal PVN neurons essentially extends Ang II projections to the spinal cord (Shigematsu et al. Citation1986; Bains and Ferguson Citation1995; Latchford and Ferguson Citation2005). There is a massive AT1 receptor-expressing tract, continuous from the SFO and the organum vasculosum lamina terminalis, with direct connection to the PVN and the median eminence (Shigematsu et al. Citation1986). Two kinds of evidence confirm that Ang II is an endogenous neurotransmitter in these circuits. First, the demonstration of the actual presence of the peptide by immunocytochemical methods is in good agreement with the Ang II receptor localization (Lind et al. Citation1985). The second line of evidence is provided by a large series of elegant studies demonstrating specific neuronal stimulation by Ang II in the SFO and PVN, and by the discovery of specific Ang II connections between these areas (Shigematsu et al. Citation1986; Ferguson and Washburn Citation1998; Ferguson et al. Citation2001). The SFO–PVN connection is a central pathway of interaction between circulating Ang II with effects in SFO neurons, PVN neurons central to the HPA axis response to stress, and spinal cord neurons contributing to regulate specific motor responses. In the PVN, AT1 Ang II receptors are localized to CRF-producing parvocellular neurons (Aguilera et al. Citation1995b), and Ang II regulates parvocellular neurons directly, in contrast with indirect regulation of magnocellular neurons and neurons projecting to the spinal cord, through actions on GABA and glutamate interneurons (Latchford and Ferguson Citation2004, Citation2005; Pan Citation2004). For these reasons, the SFO–PVN pathway is central to the control of integrated responses to stress.
Actions of angiotensin II as a neurotransmitter to influence excitability of specific neurons
There is extensive literature demonstrating that Ang II controls neuronal excitability and firing frequency by a number of mechanisms. Ang II-induced increase in neuronal excitability includes modulation of M-type K + channels (Zaika et al. Citation2006), a non-selective cationic current (Latchford and Ferguson Citation2005) and a slowly inactivating potassium conductance (Bains et al. Citation2001). Increase in neuronal firing rate by Ang II is dependent on PKC-dependent and -independent mechanisms (Wang et al. Citation1997).
The response to stress
The response to stress is initiated with the stimulation of sensory pathways, leading to a series of adaptive central mechanisms and culminating in rapid activation of the hypothalamic-pituitary-adrenal (HPA) axis and a sympathoadrenal response (Goldstein and McEwen Citation2002). The HPA axis response includes a rapid increase in CRF release into the portal circulation, followed by increased anterior pituitary ACTH and adrenocortical glucocorticoid secretion, and increased synthesis of CRF in the PVN. The sympathoadrenal response to stress is characterized by enhanced release of predominantly adrenaline, with some noradrenaline, from the adrenal medulla and sympathetic nerve stimulation with enhanced noradrenaline output.
The role of circulating RAS in the stress response
There are many indications to propose circulating Ang II as an important regulatory hormone in stress. Acute stress, through sympathetic activation of β-adrenergic receptors, increases the formation of renin, the rate-limiting enzyme in Ang II formation, leading to enhanced Ang II generation and increased Ang II levels in the circulation (Xang et al. Citation1993; Yang et al. Citation1996). The increased circulating Ang II may contribute to the enhanced anterior pituitary ACTH, glucocorticoid and aldosterone formation during stress by stimulating Ang II receptors in the anterior pituitary and adrenal cortex (Saavedra Citation1992; Aguilera Citation1993). Enhanced circulating Ang II may also mediate the increases in catecholamine release from the adrenal medulla and sympathetic nerves that are characteristic of stress (Keller-Wood et al. Citation1983; Livett et al. Citation1990). Circulating Ang II activates stress pathways in the brain, as discussed above, by interacting with Ang II receptors located in brain areas outside the blood brain barrier, the circumventricular organs, such as the SFO, intimately connected to the PVN, a crucial site in the HPA axis (Saavedra Citation1992; Bains and Ferguson Citation1995).
Local RAS systems during stress
The stress-mediated effects of circulating Ang II are associated with increased activity of local RAS, including that in the brain (Saavedra Citation1992). Stress increases the local formation of Ang II in the hypothalamus and other parts of the brain (Xang et al. Citation1993; Yang et al. Citation1996; Peng and Phillips Citation2001), where the peptide stimulates receptors inside the blood brain barrier, including the Ang II receptors localized to the CRF-forming neurons in the PVN (Aguilera et al. Citation1995a). A significant increase in the expression of Ang II receptors in the PVN and SFO occurs during repeated immobilization stress (Castrén and Saavedra Citation1988; ).
Figure 1 Increased Ang II receptor expression in the PVN after repeated restraint stress. The figure represents a typical autoradiography image of Ang II receptor binding in a coronal section of the hypothalamus through the paraventricular nuclei, as determined after incubation in the presence of [125I]-Sarcosine1-Ang II, in control rats and in rats submitted to seven repeated sessions of 2 h restraint. Black arrow indicates the third ventricle. Binding is concentrated in the parvocellular region of the PVN (white arrow). Note the intense signal generated after repeated restraint stress. Bar is 0.5 mm (modified from Castrén and Saavedra Citation1988).
![Figure 1 Increased Ang II receptor expression in the PVN after repeated restraint stress. The figure represents a typical autoradiography image of Ang II receptor binding in a coronal section of the hypothalamus through the paraventricular nuclei, as determined after incubation in the presence of [125I]-Sarcosine1-Ang II, in control rats and in rats submitted to seven repeated sessions of 2 h restraint. Black arrow indicates the third ventricle. Binding is concentrated in the parvocellular region of the PVN (white arrow). Note the intense signal generated after repeated restraint stress. Bar is 0.5 mm (modified from Castrén and Saavedra Citation1988).](/cms/asset/3c45eee0-d8c1-427f-88e4-2df9bd4aa5b9/ists_a_234965_f0001_b.gif)
The anterior pituitary, adrenal zona glomerulosa and adrenal medulla express local RAS, and locally generated Ang II contributes to the hormone and catecholamine release during stress (Saavedra Citation1992; Hilbers et al. Citation1999; Jezova et al. Citation2003). The expression of Ang II receptors in the adrenal zona glomerulosa and medulla and in the anterior pituitary was also increased during isolation or restraint stress, although these changes were dependent on the type and duration of the stressor (Armando et al. Citation2001; Leong et al. Citation2002). The concept thus emerged of participation of peripheral and central RAS during stress, and localization studies strongly suggested that enhanced stimulation of central and peripheral Ang II receptors by circulating and locally formed Ang II could play significant roles in the integrated physiological response to stress.
The role of Ang II receptor types
Ang II exerts most of its physiological actions through stimulation of AT1 receptors (Timmermans et al. Citation1993), while the function of Ang II AT2 receptors is a matter of discussion (De Gasparo and Siragy Citation1999). In the brain, AT1 receptors are concentrated in all forebrain areas belonging or related to the HPA axis, including the parvocellular PVN, the site of CRF formation (Shigematsu et al. Citation1986; Tsutsumi and Saavedra Citation1991b; Aguilera et al. Citation1995a), the median eminence, from where CRF is released to the portal circulation to stimulate pituitary ACTH secretion, and the SFO (Shigematsu et al. Citation1986; Tsutsumi and Saavedra Citation1991a). AT1 receptors are also expressed in the anterior pituitary, adrenal cortex and adrenal medulla (Tsutsumi and Saavedra Citation1991b; Israel et al. Citation1995) and in noradrenaline terminals (Lokhandwala et al. Citation1978). It is possible that AT2 receptors contribute to the effects of Ang II in the adrenal gland, since the adrenal zona glomerulosa expresses approximately equal amounts of AT1 and AT2 receptors, and in the adrenal medulla the AT2 receptor is the predominant receptor type (Israel et al. Citation1995). Adrenomedullary AT2 receptors could interact with AT1 receptors regulating not only basal catecholamine synthesis but also stress-induced release (Jezova et al. Citation2003).
Pharmacological evidence for the role of AT1 and AT2 receptors
We and others (Sumitomo et al. Citation1991; Aguilera et al. Citation1995a,Citationb; Jezova et al. Citation1998; Leong et al. Citation2002) demonstrated that it was the AT1 type of Ang II receptors located in the SFO and the PVN that increased in number during repeated immobilization stress, and that increased AT1 receptor transcription occurs earlier, during acute immobilization. AT1 receptor stimulation in parvocellular PVN CRF neurons increases CRF formation and release into the hypothalamic portal system (Sumitomo et al. Citation1991; Aguilera et al. Citation1995b), leading to stimulation of pituitary ACTH followed by corticoid formation and release. In turn, increased corticosterone secretion during stress increases AT1 receptor expression in the PVN, through stimulation of glucocorticoid response elements in the receptor promoter region (Guo et al. Citation1995). Glucocorticoids are not only able to stimulate AT1 receptor expression during stress, but they are also necessary to maintain basal AT1 receptor expression (Castrén and Saavedra Citation1989; Aguilera et al. Citation1995b). Brain Ang II also stimulates vasopressin (AVP) formation in the PVN and its release from the median eminence (Saavedra Citation1992) to participate with CRF in enhancing ACTH production (Antoni Citation1993; Volpi et al. Citation2004). In addition, during stress brain Ang II enhances central sympathetic activity with increased transcription of tyrosine hydroxylase (TH) the rate-limiting enzyme in the synthesis of catecholamines, in the locus coeruleus, the site of origin of most of the sympathetic innervation to the forebrain (Saavedra et al. Citation2006). This effect contributes to the increased adrenomedullary and peripheral sympathetic catecholamine release during stress (Saavedra Citation1992). The observation that in rats Ang II receptors in the locus coeruleus are of the AT2 type (Tsutsumi and Saavedra Citation1991a) and that AT2 receptors predominate in the adrenal medulla (Israel et al. Citation1995) suggests a participation of this receptor type in the regulation of central and peripheral sympathetic stimulation during stress (Saavedra Citation1999; Jezova et al. Citation2003). On the other hand, the PVN, rich in AT1 receptors, projects to the locus coeruleus, and AT1 receptor stimulation in the adrenal medulla is sufficient to produce adrenomedullary catecholamine release (Wong et al. Citation1990). For this reason, the relative participation of AT1 and AT2 receptors in the regulation of the reaction to stress is still a matter of discussion.
Both AT1 and AT2 receptor expression in the adrenal zona glomerulosa and medulla, and AT1 receptors in the anterior pituitary were increased during isolation or restraint stress (Armando et al. Citation2001; Leong et al. Citation2002). These observations indicated that circulating or locally formed Ang II, by stimulating AT1 receptors, could contribute to the secretion of ACTH from the pituitary gland, aldosterone from the adrenal zona glomerulosa, and catecholamines from the adrenal medulla during stress (Ganong and Murakami Citation1987; Jezova et al. Citation2003), with perhaps an accessory and still not well-characterized participation of effects mediated by AT2 receptor stimulation. Increased AT1 receptor expression in key areas of the HPA axis would potentiate the effect of Ang II.
The selective localization of Ang II receptors, the increase in circulating and brain Ang II and the enhanced expression of AT1 and AT2 receptors during stress strongly suggest the participation of Ang II in the stimulation of all components of the HPA axis, and the central and peripheral sympathetic systems during stress.
To further establish the precise role of Ang II in stress, it was necessary to determine the effect of receptor blockade during stress. We asked the question whether or not the hormonal and sympathoadrenal reaction to stress was modified in rats submitted to previous AT1 receptor blockade. We studied the response to stress after sustained blockade of peripheral and brain AT1 receptors with the selective, potent, insurmountable AT1 antagonist candesartan (Morsing Citation1999; Sever Citation1999; Timmermans Citation1999). When candesartan was administered peripherally for 2 weeks, we found a significant decrease in AT1 receptor binding, not only in peripheral tissues and circumventricular organs, but also in the PVN and in the nucleus of the solitary tract (Nishimura et al. Citation2000a). This indicated that candesartan readily penetrated the blood brain barrier and could block central as well as peripheral AT1 receptors (Nishimura et al. Citation2000a).
We selected the stress of isolation, a model resulting, in rodents, from the restriction from freely regulating exposure to novel surroundings and access to familiar territory, because of its clinical relevance. We studied the effect of candesartan administered for 2 weeks before isolation. Twenty-four hours of isolation enhanced AT1 receptor expression in the PVN (Armando et al. Citation2001) to an extent similar to the increase in AT1 receptors that occurs during repeated immobilization stress (Castrén and Saavedra Citation1988), increased pituitary ACTH, decreased pituitary vasopressin and increased adrenal corticosterone, aldosterone, catecholamines and adrenal TH transcription (Armando et al. Citation2001; ). Pretreatment with candesartan blocked AT1 receptor binding after isolation in peripheral tissues and in the brain, in a manner similar to that previously observed in unstressed animals, prevented the increase in pituitary ACTH and adrenal corticosterone and the decrease in pituitary vasopressin during isolation, decreased the adrenomedullary catecholamine response, including the isolation-induced increase in adrenomedullary TH, and decreased the urinary excretion of catecholamines, corticosterone and AVP (Armando et al. Citation2001; ). These observations confirmed that Ang II AT1 receptor stimulation was necessary for the HPA axis response during stress, for the stress-induced enhanced AVP release from the posterior pituitary (Armando et al. Citation2001), and for the central and peripheral sympathetic stimulation during stress (Saavedra Citation1992).
Figure 2 Effect of pretreatment with an AT1 receptor antagonist on the stress-induced pituitary ACTH content and urinary corticosterone excretion. Isolation stress increases pituitary ACTH. Pretreatment with candesartan prevents the increase in pituitary ACTH and decreases the urinary excretion of corticosterone. *P < 0.05 vs. grouped control and isolated rats pretreated with candesartan. #P < 0.05 vs. isolated rats treated with vehicle (modified from Armando et al. Citation2001).
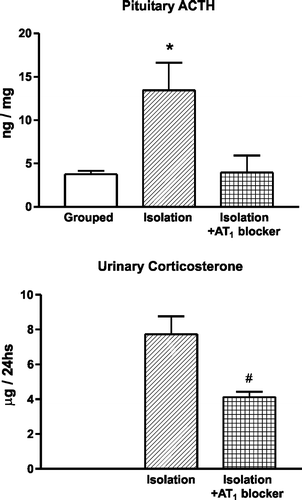
Therapeutic effect of AT1 receptor blockade
Simultaneous antagonism of peripheral and brain AT1 receptors could represent an advantage in the control of the stress reaction. To determine whether or not AT1 receptor blockade could be of therapeutic benefit, we studied the effects of candesartan on the development of a stress-induced disorder, the formation of gastric ulcers induced by a marked stress, that of cold-restraint in the rat (Bregonzio et al. Citation2003).
We found that candesartan dramatically decreased the number of ulcerations produced by cold-restraint stress (), preventing the stress-induced reduction on gastric blood flow and partially reducing sympathoadrenal stimulation (Bregonzio et al. Citation2003).
Figure 3 Prevention of gastric mucosal lesions induced by cold restraint stress by pretreatment with AT1 receptor antagonists. Microphotographs of hematoxylin-eosin-stained sections of the glandular portion of the stomach in rats submitted to cold-restraint stress treated with vehicle (left) or with candesartan (right), for 2 weeks before the stress. The lesion in the rat submitted to stress and treated with vehicle (left) involves the entire depth of the gastric mucosa. Pretreatment with candesartan for 2 weeks (right) prevented the development of stress-induced gastric ulcers. Bar is 100 μm (modified from Bregonzio et al. Citation2003).
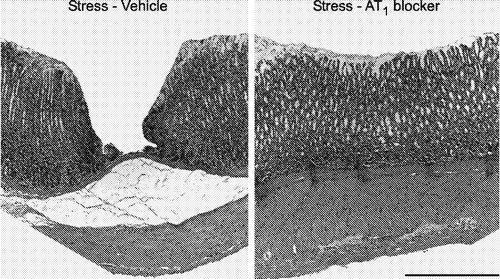
Several interrelated mechanisms are involved in the protective effect of the AT1 receptor antagonist. During stress there is a significant reduction in the blood flow to the gastric mucosa, produced by the combination of enhanced sympathetic stimulation and Ang II release with the resultant increase in activity of AT1 receptors in the gastric vasculature (Heinemann et al. Citation1999) leading to decreased blood flow and ischemia. We believe that AT1 receptor inhibition protected gastric blood flow during stress by reducing Ang II- and catecholamine-induced vasoconstriction, therefore, reducing gastric ulcer formation (Bregonzio et al. Citation2003). The protection of gastric blood flow by AT1 receptor inhibition prior to stress is similar to the protective effect on cerebral blood flow during brain ischemia (Nishimura et al. Citation2000b; Ito et al. Citation2002).
We also found that cold restraint stress produced a marked inflammatory reaction in the gastric mucosa, with increased expression of the proinflammatory cytokine tumor necrosis factor α (TNF-α), the adhesion molecule intercellular adhesion molecule-1 (ICAM-1) and the number of infiltrating neutrophils in the gastric mucosa (Bregonzio et al. Citation2003). Such an inflammatory reaction is known to play a crucial role in the progression of gastric injury (Hamaguchi et al. Citation2001). Pretreatment with candesartan prevented the gastric inflammation, revealing an anti-inflammatory effect of AT1 receptor blockade (Bregonzio et al. Citation2003). Conversely, AT1 blockade did not prevent the increase in adrenal corticosterone produced by cold-restraint stress (Bregonzio et al. Citation2003). Preservation of the glucocorticoid response during stress may contribute to the therapeutic effect of candesartan, because corticoids have been proposed to protect against gastric ulceration (Filaretova et al. Citation1998). Thus, our experiments demonstrate a clear protective, antistress effect of candesartan in an acute stress-induced disorder.
Effect of AT1 blockade on higher centers
The regulation of the stress response by AT1 receptors includes regulatory effects at higher central levels. Since AT1 receptor blockade profoundly affected hypothalamic CRF, we studied the effect of candesartan on cortical CRF systems. CRF acts as a modulator, predominantly through CRF1 receptor stimulation, in centers higher than the hypothalamus, such as the cortex, to influence and integrate stress-induced behaviors (Brunson et al. Citation2002). Isolation stress decreases cortical CRF1 receptor expression in the cortex, an effect prevented by AT1 receptor inhibition prior to stress (Saavedra et al. Citation2006; ). The stress-related decrease in receptor binding can be interpreted as decreased expression or alternatively as increased receptor occupancy due to enhanced CRF release during stress as suggested earlier (Keck and Holsboer Citation2001; Brunson et al. Citation2002). These changes are prevented by prior AT1 receptor blockade. Isolation stress also decreased flunitrazepam binding in the frontal and parietal cortex, a characteristic response to stress (Medina et al. Citation1983; Bremner et al. Citation2000) and an effect also prevented by prior AT1 receptor blockade. The benzodiazepine binding site is part of the GABAA receptor complex, regulating, in higher centers, the response to anxiety (Serra et al. Citation1999; Smith Citation2001). We postulated that AT1 Ang II receptors may be involved in higher regulatory mechanisms controlling the behavioral and cognitive responses to stress and anxiety. We tested this hypothesis in an elevated plus-maze. Candesartan treatment significantly enhanced the time spent in the open arms, indicating a clear anxiolytic effect of the AT1 receptor antagonist (Saavedra et al. Citation2006; ). Hyperactivity of the HPA axis and of CRF neurons regulating higher brain centers are confirmed findings in anxiety and in stress-related affective disorders (Keck and Holsboer Citation2001). Our observations indicated that antagonism of brain Ang II AT1 receptors () could open a new lead in the treatment of anxiety and other stress-related psychiatric conditions.
Figure 4 Effect of AT1 receptor blockade on expression of CRF1 receptor binding in the cerebral cortex of rats submitted to isolation stress. Figures represent autoradiographic images of cortical sections incubated in the presence of 0.2 nM of [125I]-sauvagine to reveal CRF receptors. Arrows point to cortex, layer IV. Bar is 3 mm. Columns are quantitative autoradiographic measurements of CRF1 receptor binding, specifically displaceable by addition of 13 nM antalarmin. Results are means ± SEM, for groups of six rats measured individually. *P < 0.05 vs. control grouped and isolated, candesartan-treated rats, one-way ANOVA followed by Neuman Keul's test (modified from Saavedra et al. Citation2006).
![Figure 4 Effect of AT1 receptor blockade on expression of CRF1 receptor binding in the cerebral cortex of rats submitted to isolation stress. Figures represent autoradiographic images of cortical sections incubated in the presence of 0.2 nM of [125I]-sauvagine to reveal CRF receptors. Arrows point to cortex, layer IV. Bar is 3 mm. Columns are quantitative autoradiographic measurements of CRF1 receptor binding, specifically displaceable by addition of 13 nM antalarmin. Results are means ± SEM, for groups of six rats measured individually. *P < 0.05 vs. control grouped and isolated, candesartan-treated rats, one-way ANOVA followed by Neuman Keul's test (modified from Saavedra et al. Citation2006).](/cms/asset/80e0f9d0-06c6-423c-ba2f-25a6b47f0940/ists_a_234965_f0004_b.gif)
Figure 5 Anxiolytic effect of candesartan. The figure shows the increase in the time spent in the open arms, the number of open arm entries and the number of closed arm entries in an elevated plus-maze by rats pretreated for 14 days with 0.5 mg/kg/day of candesartan (AT1 blocker), administered subcutaneously by means of an osmotic minipump. Increased number of open arm entries and increased time spent in the open arm are signs of decreased anxiety. The total time on the plus-maze was 5 min. *P < 0.05 vs. vehicle-treated rats (modified from Saavedra et al. Citation2006).
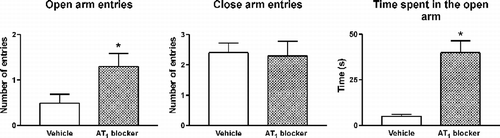
Figure 6 Sites of effect of AT1 receptor antagonists on the regulation of peripheral and brain components of the stress reaction. Left black arrows: AT1 receptor antagonists block AT1 receptor stimulation in the adrenal and pituitary glands, the median eminence, PVN, amygdala, hippocampus and cerebral cortex. Right white arrows: sites of glucocorticoid feedback regulation during stress in the pituitary gland, the median eminence, PVN, hippocampus and cortex.
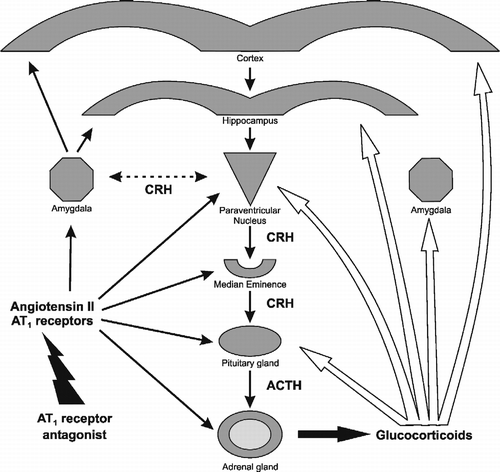
Conclusions
Local RAS systems are expressed in brain areas, pituitary and adrenal gland, and stress produces enhanced circulating and locally formed Ang II and increased AT1 receptor expression. This suggests that Ang II should be considered a potentially important factor in stress.
Prior peripheral and central AT1 receptor inhibition prevents the hormonal and sympathoadrenal response to isolation stress. This treatment also protects the gastric mucosa from ulceration during intense cold-restraint stress, by a combination of gastric blood flow protection and anti-inflammatory actions with preservation of protective glucocorticoid effects.
AT1 receptor inhibition prevents the cortical CRF and GABAA response to isolation, and reduces anxiety.
AT1 receptor inhibition with receptor blockers capable of inhibiting not only peripheral but also central AT1 receptors has a potential therapeutic use in stress-related disorders.
Acknowledgements
This study was supported by the Division of Intramural Research Programs, National Institute of Mental Health, National Institutes of Health, Department of Health and Human Services, USA.
References
- Aguilera G. Factors controlling steroid biosynthesis in the zona glomerulosa of the adrenal. J Steroid Biochem Mol Biol 1993; 45: 147–151
- Aguilera G, Kiss A, Luo X. Increased expression of type1 angiotensin II receptors in the hypothalamic paraventricular nucleus following stress and glucocorticoid administration. J Neuroendocrinol 1995a; 7: 775–783
- Aguilera G, Young WS, Kiss A, Bathia A. Direct regulation of hypothalamic corticotropin-releasing-hormone neurons by angiotensin II. Neuroendocrinology 1995b; 61: 437–444
- Antoni FA. Vasopressinergic control of pituitary adrenocorticotropin secretion comes of age. Front Neuroendocrinol 1993; 14: 76–122
- Armando I, Carranza A, Nishimura Y, Hoe KL, Barontini M, Terrón JA, Falcón-Neri A, Ito T, Juorio AV, Saavedra JM. Peripheral administration of an angiotensin II AT1 receptor antagonist decreases the hypothalamic-pituitary-adrenal response to isolation stress. Endocrinology 2001; 142: 3880–3889
- Bains JS, Ferguson AV. Paraventricular nucleus neurons projecting to the spinal cord receive excitatory input from the subfornical organ. Am J Physiol 1995; 268: R625–R633
- Bains JS, Follwell MJ, Latchford KJ, Anderson JW, Ferguson AV. Slowly inactivating potassium conductance (I(D)): A potential target for stroke therapy. Stroke 2001; 32: 2624–2634
- Braun-Menéndez E, Fasciolo JC, Leloir LF, Muñoz JM. The substance causing renal hypertension. J Physiol (Lond) 1940; 98: 283–298
- Bregonzio C, Armando I, Ando H, Jezova M, Baiardi G, Saavedra JM. Antiinflammatory effects of angiotensin II AT1 receptor antagonism prevent stress-induced gastric injury. Am J Physiol Gastrointest Liver Physiol 2003; 285: G414–G423
- Bremner JD, Innis RB, Southwick SM, Staib L, Zoghbi S, Charney DS. Decreased benzodiazepine receptor binding in prefrontal cortex in combat-related posttraumatic stress disorder. Am J Psychiatry 2000; 157: 1120–1126
- Brunson KL, Grigoriadis DE, Lorang MT, Baram TZ. Corticotropin-releasing hormone (CRH) downregulates the function of its receptor (CRF1) and induces CRF1 expression in hippocampal and cortical regions of the immature rat brain. Exp Neurol 2002; 176: 75–86
- Castrén E, Saavedra JM. Repeated stress increases the density of angiotensin II binding sites in rat paraventricular nucleus and subfornical organ. Endocrinology 1988; 122: 370–372
- Castrén E, Saavedra JM. Angiotensin II receptors in paraventricular nucleus, subfornical organ, and pituitary gland of hypophysectomized, adrenalectomized, and vasopressin-deficient rats. Proc Natl Acad Sci USA 1989; 86: 725–729
- De Gasparo M, Siragy HM. The AT2 receptor: Fact, fancy and fantasy. Regul Pept 1999; 81: 11–24
- Ferguson AV, Washburn DLS. Angiotensin II: A peptidergic neurotransmitter in central autonomic pathways. Prog Neurobiol 1998; 54: 169–192
- Ferguson AV, Washburn DLS, Latchford KJ. Hormonal and neurotransmitter roles for angiotensin in the regulation of central autonomic function. Exp Biol Med 2001; 226: 85–96
- Filaretova LP, Filaretov AA, Makara GB. Corticosterone increase inhibits stress-induced gastric erosions in rats. Am J Physiol 1998; 274: G1024–G1030
- Ganong WF, Murakami K. The role of angiotensin II in the regulation of ACTH secretion. Ann N Y Acad Sci 1987; 512: 176–186
- Goldstein DS, McEwen B. Allostasis, homeostats, and the nature of stress. Stress 2002; 5: 55–58
- Guo DF, Uno S, Ishihata A, Nakamura N, Inagami T. Identification of a cis-acting glucocorticoid responsive element in the rat angiotensin II type 1A promoter. Circ Res 1995; 77: 249–257
- Hamaguchi M, Watanabe T, Higuchi K, Tominaga K, Fujiwara Y, Arakawa T. Mechanisms and roles of neutrophil infiltration in stress-induced gastric injury in rats. Dig Dis Sci 2001; 46: 2708–2715
- Heinemann A, Sattler V, Jocic M, Wienen W, Holzer P. Effect of angiotensin II and telmisartan, an angiotensin1 receptor antagonist, on rat gastric mucosal blood flow. Aliment Pharmacol Ther 1999; 13: 347–355
- Hilbers U, Peters J, Bornstein SR, Correa FM, Jöhren O, Saavedra JM, Ehrhart-Bornstein M. Local renin-angiotensin system is involved in K+-induced aldosterone secretion from human adrenocortical NCI-H295 cells. Hypertension 1999; 33: 1025–1030
- Israel A, Strömberg C, Tsutsumi K, Garrido MDR, Torres M, Saavedra JM. Angiotensin II receptor subtypes and phosphoinositide hydrolysis in rat adrenal medulla. Brain Res Bull 1995; 38: 441–446
- Ito T, Yamakawa H, Bregonzio C, Terrón JA, Falcón-Neri A, Saavedra JM. Protection against ischemia and improvement of cerebral blood flow in genetically hypertensive rats by chronic pretreatment with an angiotensin II AT1 antagonist. Stroke 2002; 33: 2297–2303
- Jezova D, Ochedalski T, Kiss A, Aguilera G. Brain angiotensin II modulates sympathoadrenal and hypothalamic pituitary adrenocortical activation during stress. J Neuroendocrinol 1998; 10: 67–72
- Jezova M, Armando I, Bregonzio C, Yu Z-X, Qian S, Ferrans VJ, Imboden H, Saavedra JM. Angiotensin II AT1 and AT2 receptors contribute to maintain basal adrenomedullary norepinephrine synthesis and tyrosine hydroxylase transcription. Endocrinology 2003; 144: 2092–2101
- Keck ME, Holsboer F. Hyperactivity of CRH neuronal circuits as a target for therapeutic interventions in affective disorders. Peptides 2001; 22: 835–844
- Keller-Wood ME, Shinsako J, Dallman MF. Inhibition of the adrenocorticotropin and corticosteroid responses to hypoglycemia after prior stress. Endocrinology 1983; 113: 491–496
- Latchford KJ, Ferguson AV. Ang II-induced excitation of paraventricular nucleus magnocellular neurons: A role for glutamate interneurons. Am J Physiol Regul Integr Comp Physiol 2004; 286: R894–R902
- Latchford KJ, Ferguson AV. Angiotensin depolarizes parvocellular neurons in paraventricular nucleus through modulation of putative nonselective cationic and potassium conductances. Am J Physiol Regul Integr Comp Physiol 2005; 289: R52–R58
- Leong DS, Terrón JA, Falcón-Neri A, Armando I, Ito T, Jöhren O, Tonelli LH, Hoe K-L, Saavedra JM. Restraint stress modulates brain, pituitary and adrenal expression of angiotensin II AT1A, AT1B and AT2 receptors. Neuroendocrinology 2002; 75: 227–240
- Lind RW, Swanson LW, Ganten D. Organization of angiotensin II immunoreactive cells and fibers in the rat central nervous system. An immunohistochemical study Neuroendocrinology 1985; 40: 2–24
- Livett BG, Marley PD, Wan DC, Zhou XF. Peptide regulation of adrenal medullary function. J Neural Transm Suppl 1990; 29: 77–89
- Lokhandwala MF, Amelang E, Buckley JP. Facilitation of cardiac sympathetic function by angiotensin II: Role of presynaptic angiotensin receptors. Eur J Pharmacol 1978; 52: 405–409
- Medina JH, Novas ML, Wolfman CNV, De Stein ML, DeRobertis E. Benzodiazepine receptors in rat cerebral cortex and hippocampus undergo rapid and reversible changes after acute stress. Neuroscience 1983; 9: 331–335
- Morsing P. Candesartan: A new generation angiotensin II AT1 receptor blocker: Pharmacology, antihypertensive efficacy, renal function, and renoprotection. J Am Soc Nephrol 1999; 10: S248–S254
- Nishimura Y, Ito T, Hoe K-L, Saavedra JM. Chronic peripheral administration of the angiotensin II AT1 receptor antagonist candesartan blocks brain AT1 receptors. Brain Res 2000a; 871: 29–38
- Nishimura Y, Ito T, Saavedra JM. Angiotensin II AT1 blockade normalizes cerebrovascular autoregulation and reduces cerebral ischemia in spontaneously hypertensive rats. Stroke 2000b; 31: 2478–2486
- Page IH. Hypertension mechanisms. Grune & Stratton, New York 1987; 1102
- Page IH, Helmer OM. A crystalline pressor substance (angiotensin) resulting from the reaction between renin and renin activator. J Exp Med 1940; 71: 29–42
- Pan HL. Brain angiotensin II and synaptic transmission. Neuroscientist 2004; 10: 422–431
- Paul M, Poyan Mehr A, Kreutz R. Physiology of local renin-angiotensin systems. Physiol Rev 2006; 86: 747–803
- Peng JF, Phillips MI. Opposite regulation of brain angiotensin type1 and type 2 receptors in cold-induced hypertension. Regul Pept 2001; 97: 91–102
- Saavedra JM. Brain and pituitary angiotensin. Endocrinol Rev 1992; 13: 329–380
- Saavedra JM. Emerging features of brain angiotensin receptors. Regul Pept 1999; 85: 31–45
- Saavedra JM. Brain angiotensin II: New developments, unanswered questions and therapeutic opportunities. Cell Mol Neurobiol 2005; 25: 485–512
- Saavedra JM, Ando H, Armando I, Baiardi G, Bregonzio C, Juorio A, Macova M. Anti-stress and anti-anxiety effects of centrally acting angiotensin II AT1 receptor antagonists. Regul Pept 2005; 128: 227–238
- Saavedra JM, Armando I, Bregonzio C, Juorio A, Macova M, Pavel J, Sanchez-Lemus E. A centrally acting, anxiolytic angiotensin II AT1 receptor antagonist prevents the isolation stress-induced decrease in cortical CRF1 receptor and benzodiazepine binding. Neuropsychopharmacology 2006; 31: 1123–1134
- Serra M, Concas A, Mostallino MC, Chessa MF, Stomati M, Petraglia F, Genazzani AR, Biggio G. Antagonism by pivagabine of stress-induced changes in GABAA receptor function and corticotropin-releasing factor concentrations in rat brain. Psychoneuroendocrinology 1999; 24: 269–284
- Sever PS. Key features of candesartan cilexetil and a comparison with other angiotensin II receptor antagonists. J Hum Hypertens 1999; 13(Suppl. 1)S3–S10
- Shigematsu K, Saavedra JM, Plunkett LM, Kurihara M, Correa FMA. Angiotensin II binding sites in the anteroventral-third ventricle (AV3V) area and related structures of the rat brain. Neurosci Lett 1986; 67: 37–41
- Smith TA. Type A gamma-aminobutyric acid (GABAA) receptor subunits and benzodiazepine binding: Significance to clinical syndromes and their treatment. Br J Biomed Sci 2001; 58: 111–121
- Sumitomo T, Suda T, Nakano Y, Tozawa F, Yamada M, Demura H. Angiotensin II increases the corticotropin-releasing factor messenger ribonucleic acid levels in the rat hypothalamus. Endocrinology 1991; 128: 2248–2252
- Timmermans PB. Pharmacological properties of angiotensin II receptor antagonists. Can J Cardiol 1999; 15(Suppl. F)26 F–28 F
- Timmermans PBMWM, Wong PC, Chiu AT, Herblin WF, Benfield P, Carini DJ, Lee RJ, Wexler RR, Saye JAM, Smith RD. Angiotensin II receptors and angiotensin II receptors antagonists. Pharmacol Rev 1993; 45: 205–251
- Tsutsumi K, Saavedra JM. Characterization and development of angiotensin II receptor subtypes (AT1 and AT2) in rat brain. Am J Physiol 1991a; 261: R209–R216
- Tsutsumi K, Saavedra JM. Angiotensin II receptor subtypes in median eminence and basal forebrain areas involved in the regulation of pituitary function. Endocrinology 1991b; 129: 3001–3008
- Volpi S, Rabadan-Diehl C, Aguilera G. Vasopressinergic regulation of the hypothalamic pituitary adrenal axis and stress adaptation. Stress 2004; 7: 75–83
- Wang D, Gelband CH, Sumners C, Posner P. Mechanisms underlying the chronotropic effect of angiotensin II on cultured neurons from rat hypothalamus and brain stem. J Neurophysiol 1997; 78: 1013–1020
- Wong PC, Hart SD, Zaspel AM, Chiu AT, Ardecky RJ, Smith RD, Timmermans PB. Functional studies of nonpeptide angiotensin II receptor subtype-specific ligands: DuP 753 (AII-1) and PD 123177 (AII-2). J Pharmacol Exp Ther 1990; 255: 584–592
- Xang G, Xi ZX, Wan Y, Wang H, Bi G. Changes in circulating and tissue angiotensin II during acute and chronic stress. Biol Signals 1993; 2: 166–172
- Yang G, Wan Y, Zhu Y. Angiotensin II—an important stress hormone. Biol Signals 1996; 5: 1–8
- Zaika O, Lara LS, Gamper N, Helgemann DW, Jaffe DB, Shapiro MS. Angiotensin II regulates neuronal excitability via phosphatidylinositol 4,5-bisphosphate-dependent modulation of Kv7 (M-type) K+ channels. J Physiol 2006; 575: 49–67