Abstract
The kynurenine (KYN) pathway, which is initiated by indoleamine 2,3-dioxygenase (IDO), is a tryptophan (TRP) metabolic pathway. It shares TRP with the serotonin (5-hydroxytryptamine, 5-HT) pathway. In major depression, activation of the KYN pathway may deplete 5-HT. In the present study we investigated the influence of various risk factors for depression, such as ageing, social isolation and psychological stress, on TRP metabolism. Male ICR mice (postnatal day, PND, 21) were divided into two housing conditions, isolation and group housing, reared for 4 weeks (young adult) or 5 months (adult) and exposed to novelty stress. We measured TRP, KYN and 5-HT contents in the prefrontal cortex, hippocampus, amygdala and dorsal raphe nuclei to investigate the balance between the KYN and 5-HT pathways. Ageing decreased TRP and KYN and increased 5-HT. Thus, ageing shifted the balance to the latter. In the younger group, social isolation decreased TRP and KYN and increased the 5-HT/TRP ratio, whereas novelty stress increased TRP and KYN and decreased the 5-HT/TRP ratio. Thus, social isolation shifted the balance to the latter, whereas novelty stress shifted it to the former. In the older group, these effects were restricted to specific brain regions. Ageing and social isolation counteracted novelty stress effects on TRP metabolism.
Introduction
Tryptophan (TRP) metabolism has two main pathways: one is the kynurenine (KYN) pathway, which is initiated by the enzyme indoleamine 2,3-dioxygenase (IDO) and the other is the serotonin (5-HT) pathway, which is initiated by the enzyme tryptophan hydroxylase (TPH). These two pathways share TRP with each other, although almost all TRP, i.e. ∼99% of dietary intake, is metabolised by the former (Stone and Darlington Citation2002). Immunological challenges are known to induce IDO activity (Stone and Darlington Citation2002; Konsman et al. Citation2002; Widner et al. Citation2002; Moffett and Namboodiri Citation2003; Myint and Kim Citation2003; Wichers and Maes Citation2004). The activated IDO metabolises TRP to KYN, and may deprive TPH of its substrate, TRP. Thus, IDO activation may activate the KYN pathway and lead to the consumption of TRP as the substrate of TPH, resulting in 5-HT depletion (Konsman et al. Citation2002; Widner et al. Citation2002; Myint and Kim Citation2003; Wichers and Maes Citation2004).
The finding that depression is induced by cytokine therapy indicates a correlation between the severity of depressive symptoms and a decrease in serum TRP and/or a KYN increase (Bonaccorso et al. Citation2002; Capuron et al. Citation2002, Citation2003).
To explain the relationship between major depression and immunological activities, a macrophage theory (Smith Citation1991) indicated that activated macrophages play a role in the clinical onset and pathophysiology of major depression, and suggested that the large amount of IL-1 released from activated macrophages directly stimulates CRH release from the paraventricular nucleus (PVN) in the hypothalamus. Thus, hyperactivity in the hypothalamo-pituitary–adrenal (HPA) axis in major depression may be caused by macrophage hyperactivity. Furthermore, activated macrophages also relate to IDO activation as above-mentioned, and may inhibit brain 5-HT synthesis. Thus, this theory may explain both HPA-axis hyperactivity and 5-HT depletion, two main etiological and pathophysiological hypotheses of major depression (Konsman et al. Citation2002; Myint and Kim Citation2003).
IDO activation may also influence neurodegenerative and neuroprotective activity. Of the metabolites in the KYN pathway, 3-hydroxy kynurenine (3-OH KYN) and quinolinic acid (QUIN) have neurotoxic effects, whereas kynurenic acid (KYNA) has a neuroprotective effect (Myint and Kim Citation2003; Wichers and Maes Citation2004). Thus, the balance between neurodegenerative and neuroprotective effects might relate to hippocampal atrophy in chronically depressed patients.
Patients ordinarily become depressed by adverse life events and/or loss of social support, i.e. psychological and/or environmental factors, especially in older age without severe physical illness or immune therapy (Kendler et al. Citation1993; Paykel Citation1994). Hence an investigation of the influence of these factors on TRP metabolism is needed to clarify the above-mentioned hypothesis that a shift in the balance between the KYN and 5-HT pathways to the former explains the etiology and pathophysiology of major depression. An overlap between neurochemical changes elicited by stressors and immune challenges has been frequently noted. Because physiological and/or psychological stress as well as immunological challenges activate the inflammation response system, and then induce proinflammatory cytokines both in the periphery and in the brain, these cytokines may induce and activate peripheral and brain IDO. However, there is little evidence about the potential influence of ageing, environmental conditions and psychological stress on TRP metabolism. In our recent studies using an animal model, ageing, social environment such as social isolation and acute psychological stress such as exposure to novel environment, did alter brain monoamine turnover in rats (Miura et al. Citation2002a,Citationb, Citation2005a) and mice (Miura et al. Citation2004, Citation2005b, Citation2007). Both social isolation and exposure to novel environment are not mild, but strong stressors to mice and rats. Thus, we investigated the relationship between changes in brain TRP metabolism, activities in the KYN and 5-HT pathways, and these three risk factors of major depression using a murine model. We selected four brain regions for study: the prefrontal cortex, because it relates to behavioural motivation; the amygdala, because it relates to emotion; the hippocampus, because it regulates the HPA-axis and hyperactivity of the HPA-axis is closely related to the etiology and pathophysiology of depression; and the dorsal raphe nuclei, because they are the centre of brain 5-HT synthesis. The aim of the present study was to clarify whether acute psychological stress shifts the balance of activities between the KYN and 5-HT pathways to the former, and how ageing and social environment modulates the shifting of TRP metabolism.
Materials and methods
Animals
A total of 64 male ICR mice were used in the present experiments. At postnatal day 21 (PND 21), mice that had been housed in groups (7–9 per cage) were divided into two different groups according to housing conditions: i.e. group housing (7–9 per cage; n = 32) or isolation housing (1 per cage; n = 32; ). After being assigned to one of the two housing conditions, the mice were reared for 4 weeks (young adult group) or 5 months (adult group, ). The mice were further separated into two groups: in the stress group (n = 32), the mice were exposed to a 20-min novelty stress on the final day; and in the non-stress group (n = 32), the mice were not exposed to the novelty stress (). Finally, by combining the above conditions, the mice were divided into 8 groups: young adult, group housing, non-stress; young adult, group housing, stress; young adult, isolation housing, non-stress; young adult, isolation housing, stress; adult, group housing, non-stress; adult, group housing, stress; adult, isolation housing, non-stress; and adult, isolation housing, stress.
The cages used for the group-housing condition were 21 × 31 × 13 cm and the cages used for the isolation-housing condition were 17 × 29 × 13 cm. Cage exchange was performed twice a week for the group-housing group and once a week for the isolation-housing group. Food and water were provided ad libitum. The mice were kept on a 12-h light/dark cycle (lights on 07.00 h, off 19.00 h) and room temperature was maintained at 21–23°C. All efforts were made to minimise both the number of animals used and the degree of their suffering. All of the experiments were conducted in accordance with the European Communities Council Directive of 24 November 1986 (86/609/EEC). The study was approved by the ethical committee of Nagoya University Graduate School of Medicine.
Novelty stress test
In the stress group, a 20-min novelty stress session was performed on the final day (i.e. the mice were placed into a transparent plastic box (28 × 35 × 30 cm) that they had not previously experienced). The novelty stress test was performed between 14.00 and 18.00 h in a room that was separate from the holding room, and lit only by a single lamp above the novel cage.
Sample preparation
Mice in the stress group were killed by decapitation immediately after the 20-min stress session, whereas mice in the non-stress group were decapitated without exposure to stress; mice were decapitated under brief ether anaesthesia. The brains were removed and the prefrontal cortex, hippocampus, amygdala and dorsal raphe nuclei were dissected out as quickly as possible on glass plates over ice. The samples were weighed and treated with 1 ml of an ice-cold 0.2 M trichloroacetic acid solution containing 0.2 mM sodium pyrosulphite, 0.01% EDTA-2Na and 0.5 μM isoproterenol (ISO) and 3-nitro-l-tyrosine (3-N TYR) as an internal standard per 100 mg of wet tissue. The solution was sonicated and then centrifuged at 10,000g for 20 min at 4°C. The supernatant was filtered through a Millipore HV filter (0.45 μm pore size) and then subjected to both high-performance liquid chromatography (HPLC) with electrochemical detection (ECD) of 5-HT, and HPLC with fluorimetric detection (FD) of TRP and with ultraviolet (UV) detection of KYN. The standard solution was prepared using the above-mentioned ice-cold 0.2 M trichloroacetic acid solution containing 0.5 μM internal standards (ISO, 3-NTYR) and adjusted to 0.5 μM for 5-HT and KYN, and 10 μM for TRP.
HPLC determination of brain levels of 5-HT
The concentrations of 5-HT in the brain extracts were measured by HPLC with ECD. The system employed for HPLC–ECD consisted of a CMA/200 autosampler (CMA/Microdialysis AB, Stockholm, Sweden), a micro LC pump (BAS, West Lafayette, IN, USA), an LC-4C ECD (BAS), a Bio-Phase ODS-4 51-6034 column (4.0 × 110 mm; BAS), a CR-6A recorder (Shimadzu, Kyoto, Japan), an LC-26A vacuum degasser (BAS) and a CTO-10A column heater set at 35°C (Shimadzu). The mobile-phase solution consisted of 0.1 M tartaric acid–0.1 M sodium acetate buffer, pH 3.2, containing 0.5 mM EDTA-2Na, 555 μM sodium 1-octane sulfonate and 5% acetonitrile. The flow rate was 700 μl/min. The concentration of each compound was calculated by comparison with both the internal (ISO) and the external standards.
HPLC determination of brain levels of TRP and KYN
We measured levels of TRP and KYN according to the methods of Widner et al. (Citation1997) and those improved by Laich et al. (2002). A LC-10AD (Shimadzu) HPLC pump was used. For separation, reversed-phase column cartridges LiChroCART 55-4 filled with Purospher STAR Rp-18e (55 mm length, 3 μm grain size) together with a reverse-phase LiChroCART 4-4 precolumn filled with Purospher STAR RP-18e (5 μm grain size, Merck) were used. TRP was detected by RF-535 FD (Shimadzu) at an excitation wavelength of 285 nm and an emission wavelength of 365 nm. KYN and 3-NTYR were detected by a SPD-10A UV-detector (Shimadzu) at a wavelength of 360 nm.The detectors were connected in series to allow simultaneous measurements. The mobile-phase solution consisted of 15 mM l-acetic acid–sodium acetate buffer, pH 4.0, containing 2.7% acetonitrile. The flow rate was 900 μl/min at room temperature.
Statistical analyses
To examine differences in the concentrations of TRP, 5-HT and KYN, and in the ratios of 5-HT/TRP and KYN/TRP, three-way MANOVA (Wilks's lambda) for ageing, housing condition and novelty stress was conducted on dependent measures in each brain region, followed by the Tukey–Kramer test for ageing. To evaluate the interactions, further analyses were performed. In each age group, i.e. young adult and adult, two-way MANOVA (Wilks's lambda) for housing condition and novelty stress was conducted on dependent measures in each brain region, followed by the Tukey–Kramer test. P values less than 0.05 were accepted as significant.
Results
The measurements in each brain region are shown in Figure 2 and the corresponding ratios for KYN/TRP and for 5-HT/TRP are shown in Table 1.
Prefrontal cortex
Ageing, housing condition, and novelty stress
The results of three-way MANOVA were as follows: ageing (F (5, 52) = 6.533, P < 0.0001), housing condition (F (5, 52) = 17.115, P < 0.0001) and novelty stress (F (5, 52) = 17.358, P < 0.0001) significantly altered the dependent measures. The interactions between ageing and housing condition (F (5, 52) = 7.624, P < 0.0001) and between housing condition and novelty stress (F (5, 52) = 7.872, P < 0.0001) were significant, whereas the interaction between ageing and novelty stress (F (5, 52) = 1.894, P = 0.11) was not significant. The interaction among ageing, housing condition and novelty stress (F (5, 52) = 5.046, P = 0.0008) was significant.
The post hoc tests revealed that ageing significantly decreased TRP concentration (p < 0.01), whereas it increased the 5-HT concentration (p < 0.01) and the 5-HT/TRP ratio (p < 0.05, , ).
Figure 2 Changes in the TRP, KYN and serotonin (5-HT) concentrations elicited by ageing, housing condition, and novelty stress. Each bar indicates a group defined by age, stress and housing condition (n = 8). White bar, non-stress; black bar, stress. G, group housing; I, isolation housing. Values are shown as means ± SEM. The results of Tukey–Kramer test for ageing, housing condition, and novelty stress are shown. Between the young adult and adult groups, effects of ageing are shown: #, p < 0.05: ##, p < 0.01. For each age group, effects of housing condition are shown: *, p < 0.05; **, p < 0.01. Effects of novelty stress are shown: +, p < 0.05; ++, p < 0.01. A, prefrontal cortex; B, hippocampus; C, amygdala; D, dorsal raphe nuclei.
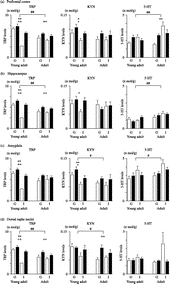
Table I. Changes in the KYN/TRP and 5-HT/TRP ratios elicited by aging, housing condition and novelty stress.
Young adult group
The results of two-way MANOVA for housing condition and novelty stress were as follows: housing condition (F (24, 5) = 30.209, P < 0.0001) and novelty stress (F (24, 5) = 21.698, P < 0.0001) significantly altered the dependent measures. The interaction between housing condition and novelty stress (F (24, 5) = 16.958, P < 0.0001) was significant.
The post hoc tests revealed that social isolation significantly decreased TRP (p < 0.01) and KYN (p < 0.05) levels, whereas it increased the KYN/TRP (p < 0.05) and 5-HT/TRP (p < 0.01) ratios (, ). Novelty stress significantly increased the TRP (p < 0.01) and KYN (p < 0.05) levels, and decreased the 5-HT/TRP ratio (p < 0.01, , ).
Adult group
The results of two-way MANOVA for housing condition and novelty stress were as follows: housing condition (F (24, 5) = 3.318, P = 0.02) and novelty stress (F (24, 5) = 4.075, P = 0.008) significantly altered the dependent measures. The interaction between housing condition and novelty stress (F (24, 5) = 1.874, P = 0.14) was not significant.
The post hoc tests revealed that social isolation significantly increased the 5-HT level (p < 0.01) and 5-HT/TRP ratio (p < 0.01, , ). Novelty stress significantly increased the TRP level (p < 0.01, , ).
Summary
Ageing decreased the TRP concentration and increased the 5-HT concentration, thus increasing the 5-HT/TRP ratio in the prefrontal cortex. Ageing shifted the balance between the KYN and 5-HT pathways to the latter. In the younger group, novelty stress increased both the TRP and KYN levels, but decreased the 5-HT/TRP ratio. Thus, novelty stress shifted the balance between the KYN and 5-HT pathways to the former, although the stress did not directly attenuate the 5-HT level. Social isolation decreased the TRP and KYN levels but increased the KYN/TRP and 5-HT/TRP ratios. In the older group, novelty stress increased the TRP level, whereas it altered neither the KYN nor 5-HT level. Thus, novelty stress did not shift the balance between the KYN and 5-HT pathways. Social isolation increased the 5-HT level and 5-HT/TRP ratio. Hence, social isolation shifted the balance between the KYN and 5-HT pathways to the latter.
Hippocampus
Ageing, housing condition, and novelty stress
Ageing (F (5, 52) = 9.240, P < 0.0001), housing condition (F (5, 52) = 8.596, P < 0.0001) and novelty stress (F (5, 52) = 13.178, P < 0.0001) significantly altered the dependent measures. The interactions between ageing and housing condition (F (5, 52) = 4.025, P = 0.004), between ageing and novelty stress (F (5, 52) = 4.613, P = 0.001) and between housing condition and novelty stress (F (5, 52) = 2.423, P = 0.048) were significant. The interaction among ageing, housing condition and novelty stress (F (5, 52) = 3.508, P = 0.008) was significant.
The post hoc tests revealed that ageing significantly decreased the TRP level (p < 0.01) but increased the 5-HT level (p < 0.01) and the 5-HT/TRP ratio (p < 0.01, , ).
Young adult group
The results of two-way MANOVA for housing condition and novelty stress were as follows: housing condition (F (24, 5) = 14.800, P < 0.0001) and novelty stress (F (24, 5) = 14.210, P < 0.0001) significantly altered dependent measures. The interaction between housing condition and novelty stress (F (24, 5) = 11.194, P < 0.0001) was significant.
The post hoc tests revealed that social isolation significantly decreased the TRP (p < 0.01) level and increased the 5-HT/TRP (p < 0.01) ratio (, ). Novelty stress significantly increased the TRP (p < 0.01) and KYN (p < 0.05) levels and decreased the 5-HT/TRP ratio (p < 0.01, , ).
Adult group
The results of two-way MANOVA for housing condition and novelty stress were as follows: housing condition (F (24, 5) = 1.208, P = 0.33) did not significantly alter the dependent measures, whereas novelty stress (F (24, 5) = 7.063, P = 0.0003) did. The interaction between housing condition and novelty stress (F (24, 5) = 0.826, P = 0.54) was not significant. The post hoc test revealed that novelty stress significantly increased the TRP (p < 0.01) level and decreased the KYN/TRP (p < 0.05) ratio (, ).
Summary
Ageing decreased the TRP concentration and increased the 5-HT concentration, thus increasing the 5-HT/TRP ratio in the hippocampus. Ageing shifted the balance between the KYN and 5-HT pathways to the latter. In the younger group, novelty stress increased the TRP and KYN levels and decreased the 5-HT/TRP ratio. Thus, novelty stress shifted the balance between the KYN and 5-HT pathways to the former, although the stress did not directly attenuate the 5-HT level. Social isolation decreased the TRP level and increased the 5-HT/TRP ratio. Thus, social isolation shifted the balance between the KYN and 5-HT pathways to the latter. In the older group, novelty stress increased the TRP level and decreased the KYN/TRP ratio. Thus, novelty stress shifted the balance between the KYN and 5-HT pathways to the latter, although the stress did not directly attenuate the KYN level.
Amygdala
Ageing, housing condition, and novelty stress
Ageing (F (5, 52) = 3.580, P = 0.007), housing condition (F (5, 52) = 8.778, P < 0.0001) and novelty stress (F (5, 52) = 11.030, P < 0.0001) significantly altered the dependent measures. The interactions between ageing and housing condition (F (5, 52) = 6.826, P < 0.0001) and between housing condition and novelty stress (F (5, 52) = 3.553, P = 0.008) were significant, whereas the interaction between ageing and novelty stress (F (5, 52) = 1.214, P = 0.32) was not significant. The interaction among ageing, housing condition and novelty stress (F (5, 52) = 4.104, P = 0.003) was significant.
The post hoc tests revealed that ageing significantly decreased the KYN concentration (p < 0.05), whereas it increased the 5-HT concentration (p < 0.05, ).
Young adult group
The results of two-way MANOVA for housing condition and novelty stress were as follows: housing condition (F (24, 5) = 18.549, P < 0.0001) and novelty stress (F (24, 5) = 11.199, P < 0.0001) significantly altered the dependent measures. The interaction between housing condition and novelty stress (F (24, 5) = 6.055, P = 0.0009) was significant.
The post hoc tests revealed that social isolation significantly decreased the TRP (p < 0.01) and KYN (p < 0.01) levels but increased the 5-HT/TRP (p < 0.01) ratio (, ). Novelty stress significantly increased the TRP (p < 0.01) and KYN (p < 0.05) concentrations but decreased the 5-HT/TRP ratio (p < 0.01, , ).
Adult group
The results of two-way MANOVA for housing condition and novelty stress were as follows: housing condition (F (24, 5) = 1.654, P = 0.18) did not significantly alter the dependent measures, whereas novelty stress (F (24, 5) = 4.407, P = 0.005) did. The interaction between housing condition and novelty stress (F (24, 5) = 1.444, P = 0.24) was not significant. The post hoc test revealed that novelty stress significantly decreased the 5-HT/TRP ratio (p < 0.05, ).
Summary
Ageing decreased the KYN concentration and increased the 5-HT concentration in the amygdala. Thus, ageing shifted the balance between the KYN and 5-HT pathways to the latter. In the younger group, novelty stress increased the TRP and KYN levels and decreased the 5-HT/TRP ratio. Thus, novelty stress shifted the balance between the KYN and 5-HT pathways to the former, although the stress did not directly attenuate the 5-HT level. Social isolation decreased the TRP and KYN levels, and increased the 5-HT/TRP ratio. Thus, social isolation shifted the balance between the KYN and 5-HT pathways to the latter. In the older group, novelty stress decreased the 5-HT/TRP ratio. Hence, novelty stress shifted the balance between the KYN and 5-HT pathways to the former, although the stress did not directly elevate KYN concentration.
Dorsal raphe nuclei
Ageing, housing condition, and novelty stress
Ageing (F (5, 52) = 3.851, P = 0.005), housing condition (F (5, 52) = 13.107, P < 0.0001) and novelty stress (F (5, 52) = 13.705, P < 0.0001) significantly altered the dependent measures. The interactions between ageing and housing condition (F (5, 52) = 3.384, P = 0.01) and between housing condition and novelty stress (F (5, 52) = 5.963, P = 0.0002) were significant, whereas the interaction between ageing and novelty stress (F (5, 52) = 1.130, P = 0.35) was not significant. The interaction among ageing, housing condition and novelty stress (F (5, 52) = 3.191, P = 0.001) was significant.
The post hoc tests revealed that ageing significantly decreased the TRP (p < 0.01) and KYN (p < 0.05) concentrations ().
Young adult group
The results of two-way MANOVA for housing condition and novelty stress were as follows: housing condition (F (24, 5) = 20.183, P < 0.0001) and novelty stress (F (24, 5) = 12.847, P < 0.0001) significantly altered the dependent measures. The interaction between housing condition and novelty stress (F (24, 5) = 9.851, P < 0.0001) was significant.
The post hoc tests revealed that social isolation significantly decreased the TRP (p < 0.01) concentration and increased the 5-HT/TRP (p < 0.01) ratio (, ). Novelty stress significantly increased the TRP (p < 0.01) level and decreased the 5-HT/TRP ratio (p < 0.01, , ).
Adult group
The results of two-way MANOVA for housing condition and novelty stress were as follows: housing condition (F (24, 5) = 1.634, P = 0.19) did not significantly alter the dependent measures, whereas novelty stress (F (24, 5) = 4.778, P = 0.004) did. The interaction between housing condition and novelty stress (F (24, 5) = 1.709, P = 0.17) was not significant.
The post hoc tests revealed that novelty stress significantly increased TRP (p < 0.01) and KYN (p < 0.01) concentrations and decreased the 5-HT/TRP (p < 0.05) ratio (, ).
Summary
Ageing decreased the TRP and KYN levels in the dorsal raphe nuclei. In the younger group, novelty stress increased the TRP concentration and decreased the 5-HT/TRP ratio. Thus, novelty stress shifted the balance between the KYN and 5-HT pathways to the former, although the stress did not directly attenuate the 5-HT level. Social isolation decreased the TRP level and increased the 5-HT/TRP ratio. Thus, social isolation shifted the balance between the KYN and 5-HT pathways to the latter. In the older group, novelty stress increased the TRP and KYN levels and decreased the 5-HT/TRP ratio. Hence, novelty stress shifted the balance between the KYN and 5-HT pathways to the former, although the stress did not directly attenuate the 5-HT level.
Overview
Ageing shifted the balance between the KYN and 5-HT pathways to the latter. In the younger group, social isolation shifted the balance to the latter, whereas novelty stress shifted it to the former. In the older group, effects of social isolation and novelty stress were restricted to specific brain regions. Ageing and social isolation counteracted novelty stress effects on TRP metabolism.
Discussion
Recent studies have suggested that changes in the balance between the activity of the KYN pathway and that of the 5-HT pathway in TRP metabolism play an important role in depression (Wichers et al. Citation2005; Myint et al. Citation2007). Here, we investigated changes in KYN system activity elicited by ageing, social environment and psychological stress to clarify whether acute psychological stress shifts the balance of activities between the KYN and 5-HT pathways to the former, and how ageing and social environment modulate the shifting of TRP metabolism.
Effects of ageing on tryptophan metabolism
Ageing decreased TRP levels, decreased KYN levels, increased 5-HT levels and increased the 5-HT/TRP ratio in the brain regions studied here. Thus, ageing shifted the balance between the KYN and 5-HT pathways to the latter. Furthermore, ageing diminished TRP and KYN levels without altering the KYN/TRP ratio. Previous studies have suggested that ageing activates the KYN pathway especially in KYNA synthesis (Gramsbergen et al. Citation1992). In a human study, Alzheimer's disease patients and age-matched controls exhibited a decrease in plasma TRP level and an increase in KYN/TRP ratio as compared to the younger control group, and these changes were more prominent in the Alzheimer's disease group than in the age-matched controls (Widner et al. Citation2000). Our results partly confirmed these studies of ageing effects.
In the present study, ageing increased the brain 5-HT level; while some previous studies have reported an increase (Santiago et al. Citation1988; Delion et al. Citation1997), no change (Ponzio et al. Citation1982; Lee et al. Citation1994) or a decrease (Gozlan et al. Citation1990; Luine et al. Citation1990). Thus, the influence of ageing on brain 5-HT levels remains controversial. Our previous study using rats showed a decrease in the 5-hydroxyindoleacetic acid (5-HIAA)/5-HT ratio, or the 5-HT turnover ratio, elicited by ageing (Miura et al. Citation2002b). Evidently, the decreased 5-HT turnover elicited by ageing may have been the cause of the increased 5-HT level. To evaluate the effect of ageing on TRP metabolism especially in altering the 5-HT pathway, the age of 6 months studied in our protocol may have not been sufficient, although mice are usually retired from breeding at this age.
Effects of social isolation and novelty stress on tryptophan metabolism, and the interaction between the two factors
Young adult group
Novelty stress increased brain TRP and KYN levels, whereas this stress did not change the 5-HT level. Although novelty stress left the KYN/TRP ratio unchanged, it decreased the 5-HT/TRP ratio. Hence, novelty stress shifted the balance between the KYN and 5-HT pathways to the former. Although social isolation left the brain KYN/TRP ratio unchanged, except for an increase in the prefrontal cortex, it increased the 5-HT/TRP ratio. Thus, social isolation shifted the balance between the KYN and 5-HT pathways to the latter.
Novelty stress may increase the brain TRP level by stimulation of the sympathetic nervous system. Plasma-free fatty acids (FFA) displace TRP bound to serum albumin, leading to an increase in the free concentration of TRP, which competes with so-called “large neutral” amino acids (LNAA) for active transport through the blood–brain barrier (Curzon et al. Citation1973). Lipolysis and FFA generation, as a result of sympathetic activation during stress, may have caused an elevation of free circulating TRP and consequently increased TRP transfer across the blood–brain barrier into the brain. In a human study, exercise decreased plasma LNAA and increased plasma free TRP concentrations and increased the free TRP/LNAA ratio (Struder et al. Citation1999). Thus, stress increased free plasma TRP, decreased LNAA and then may have increased the brain TRP level. In an animal study, the physiological stress of foot shock increased brain TRP as well as KYN levels of rats (Pawlak et al. Citation2000). These changes elicited by foot shock were similar to our results indicating an elevation of brain TRP and KYN levels by novelty stress. Thus, brain IDO activity is likely to be present in physiological conditions without immunological challenge. Other studies indicate IDO activity in neurons as well as in microglia and astrocytes (Guillemin et al. Citation2004; Roy et al. Citation2005). The results suggest that brain IDO activity present in the normal physiological conditions can metabolise the increased TRP level elicited by novelty stress into KYN. These changes may not have induced IDO as much as may occur with immunological challenge. Consequently, the stress did not alter the KYN/TRP ratio.
Adult group
Novelty stress increased brain TRP level, but did not change 5-HT level. Although novelty stress left the KYN/TRP ratio unchanged, it decreased the 5-HT/TRP ratio. Thus, novelty stress shifted the balance between the KYN and 5-HT pathways to the former. However, novelty stress decreased the KYN/TRP ratio in the hippocampus. Only in the hippocampus, novelty stress shifted the balance between the KYN and 5-HT pathways to the latter, although the stress shifted the balance to the former in the younger group. The significance of the opposite effect of novelty stress on the balance between the KYN and 5-HT pathways in the hippocampus of the older group is unclear, although this might be relevant to the etiology and pathophysiology of depression. Social isolation shifted the balance between the KYN and 5-HT pathways to the former, but only in the prefrontal cortex.
Summary
Ageing, social isolation and novelty stress, etiological risk factors of depression, did contribute to changes in TRP metabolism hypothesised as a pathophysiological mechanism of depression. However, ageing and social isolation counteracted novelty stress in the direction of shifting the balance between the KYN and 5-HT pathways. These results did not meet our expectation that the three risk factors would synergistically alter TRP metabolism. In the etiology of depression, the role of novelty stress in counteracting other factors remain unknown. Furthermore, the changes were slight as compared with those previously shown to be elicited by direct immunological stimulation, such as by proinflammatory cytokines. In particular, the three etiological risk factors studied here failed to reduce the brain 5-HT level. We suppose that our results indicate prolonged adaptation to these risk factors rather than the physiological condition of depression itself. To produce the conditions assumed to represent depression in an animal model, further breakdown of the prolonged adaptation may be needed, because prolonged adaptation frequently precedes the clinical onset of human depression. Prolonged adaptation to these factors may compromise the availability of protection against new stressors on the body and brain. Such cumulative changes elicited by stress have been termed “allostatic load or overload” (McEwen Citation2004). Hence, prolonged adaptation can be considered the precondition that increases vulnerability. Novel activation of IDO by immunological challenge or other stressors might further modify the previously shifted balance between the KYN and 5-HT pathways elicited by these risk factors and result in the onset of disease.
Acknowledgements
This research was supported by a grant from the Japanese Ministry of Health, Labour and Welfare for Comprehensive Research on Aging and Health.
References
- Bonaccorso S, Marino V, Puzella A, Pasquini M, Biondi M, Artini M, Almerighi C, Verkerk R, Meltzer H, Maes M. Increased depressive ratings in patients with hepatitis C receiving interferon-alpha-based immunotherapy are related to interferon-alpha-induced changes in the serotonergic system. J Clin Psychopharmacol 2002; 22: 86–90
- Capuron L, Ravaud A, Neveu PJ, Miller AH, Maes M, Dantzer R. Association between decreased serum tryptophan concentrations and depressive symptoms in cancer patients undergoing cytokine therapy. Mol Psychiatry 2002; 7: 468–473
- Capuron L, Neurauter G, Musselman DL, Lawson DH, Nemeroff CB, Fuchs D, Miller AH. Interferon-alpha-induced changes in tryptophan metabolism relationship to depression and paroxetine treatment. Biol Psychiatry 2003; 54: 906–914
- Curzon G, Friedel J, Knott PJ. The effect of fatty acids on the binding of tryptophan to plasma protein. Nature 1973; 242: 198–200
- Delion S, Chalon S, Guilloteau D, Lejeune B, Besnard JC, Durand G. Age-related changes in phospholipid fatty acid composition and monoaminergic neurotransmission in the hippocampus of rats fed a balanced or an n-3 polyunsaturated fatty acid-deficient diet. J Lipid Res 1997; 38: 680–689
- Gozlan H, Daval G, Verge D, Spampinato U, Fattaccini CM, Gallissot MC, el Mestikawy S, Hamon M. Aging associated changes in serotoninergic and dopaminergic pre- and postsynaptic neurochemical markers in the rat brain. Neurobiol Aging 1990; 11: 437–449
- Gramsbergen JB, Schmidt W, Turski WA, Schwarcz R. Age-related changes in kynurenic acid production in rat brain. Brain Res 1992; 588: 1–5
- Guillemin GJ, Smythe G, Takikawa O, Brew BJ. Expression of indoleamine 2,3-dioxygenase and production of quinolinic acid by human microglia, astrocytes, and neurons. Glia 2004; 49: 15–23
- Kendler KS, Kessler RC, Neale MC, Heath AC, Eaves LJ. The prediction of major depression in women: Toward an integrated etiologic model. Am J Psychiatry 1993; 150: 1139–1148
- Konsman JP, Parnet P, Dantzer R. Cytokine-induced sickness behaviour: Mechanisms and implications. Trends Neurosci 2002; 25: 154–159
- Laich A, Neurauter G, Widner B, Fuchs D. More rapid method for simultaneous measurement at tryptophan and kynurenine by HPLC. 2002; 48: 579–581, Clin.Chem
- Lee JM, Ross ER, Gower A, Paris JM, Martensson R, Lorens SA. Spatial learning deficits in the aged rat: Neuroanatomical and neurochemical correlates. Brain Res Bull 1994; 33: 489–500
- Luine V, Bowling D, Hearns M. Spatial memory deficits in aged rats: Contributions of monoaminergic systems. Brain Res 1990; 537: 271–278
- McEwen BS. Protection and damage from acute and chronic stress: Allostasis and allostatic overload and relevance to the pathophysiology of psychiatric disorders. Ann NY Acad Sci 2004; 1032: 1–7
- Miura H, Qiao H, Ohta T. Attenuating effects of the isolated rearing condition on increased brain serotonin and dopamine turnover elicited by novelty stress. Brain Res 2002a; 926: 10–17
- Miura H, Qiao H, Ohta T. Influence of aging and social isolation on changes in brain monoamine turnover and biosynthesis of rats elicited by novelty stress. Synapse 2002b; 46: 116–124
- Miura H, Qiao H, Kitagami T, Ohta T. Fluvoxamine, a selective serotonin reuptake inhibitor, suppresses tetrahydrobiopterin in the mouse hippocampus. Neuropharmacology 2004; 46: 340–348
- Miura H, Qiao H, Kitagami T, Ohta T, Ozaki N. Effects of fluvoxamine on levels of dopamine, serotonin, and their metabolites in the hippocampus elicited by isolation housing and novelty stress in adult rats. Int J Neurosci 2005a; 115: 367–378
- Miura H, Qiao H, Kitagami T, Ohta T, Ozaki N. Fluvoxamine, a selective serotonin reuptake inhibitor, suppresses tetrahydrobiopterin levels and dopamine as well as serotonin turnover in the mesoprefrontal system of mice. Psychopharmacology (Berl) 2005b; 177: 307–314
- Miura H, Kitagami T, Ozaki N. Suppressive effect of paroxetine, a selective serotonin uptake inhibitor, on tetrahydrobiopterin levels and dopamine as well as serotonin turnover in the mesoprefrontal system of mice. Synapse 2007; 61: 698–706
- Moffett JR, Namboodiri MA. Tryptophan and the immune response. Immunol Cell Biol 2003; 81: 247–265
- Myint AM, Kim YK. Cytokine–serotonin interaction through IDO: A neurodegeneration hypothesis of depression. Med Hypotheses 2003; 61: 519–525
- Myint AM, Kim YK, Verkerk R, Scharpe S, Steinbusch H, Leonard B. Kynurenine pathway in major depression: Evidence of impaired neuroprotection. J Affect Disord 2007; 98: 143–151
- Pawlak D, Takada Y, Urano T, Takada A. Serotonergic and kynurenic pathways in rats exposed to foot shock. Brain Res Bull 2000; 52: 197–205
- Paykel ES. Life events, social support and depression. Acta Psychiatr Scand Suppl 1994; 377: 50–58
- Ponzio F, Calderini G, Lomuscio G, Vantini G, Toffano G, Algeri S. Changes in monoamines and their metabolite levels in some brain regions of aged rats. Neurobiol Aging 1982; 3: 23–29
- Roy EJ, Takikawa O, Kranz DM, Brown AR, Thomas DL. Neuronal localization of indoleamine 2,3-dioxygenase in mice. Neurosci Lett 2005; 387: 95–99
- Santiago M, Machado A, Reinoso-Suarez F, Cano J. Changes in biogenic amines in rat hippocampus during development and aging. Life Sci 1988; 42: 2503–2508
- Smith RS. The macrophage theory of depression. Med Hypotheses 1991; 35: 298–306
- Stone TW, Darlington LG. Endogenous kynurenines as targets for drug discovery and development. Nat Rev Drug Discov 2002; 1: 609–620
- Struder HK, Hollmann W, Platen P, Wostmann R, Weicker H, Molderings GJ. Effect of acute and chronic exercise on plasma amino acids and prolactin concentrations and on [3H]ketanserin binding to serotonin2A receptors on human platelets. Eur J Appl Physiol Occup Physiol 1999; 79: 318–324
- Wichers MC, Maes M. The role of indoleamine 2,3-dioxygenase (IDO) in the pathophysiology of interferon-alpha-induced depression. J Psychiatry Neurosci 2004; 29: 11–17
- Wichers MC, Koek GH, Robaeys G, Verkerk R, Scharpe S, Maes M. IDO and interferon-alpha-induced depressive symptoms: A shift in hypothesis from tryptophan depletion to neurotoxicity. Mol Psychiatry 2005; 10: 538–544
- Widner B, Werner ER, Schennach H, Wachter H, Fuchs D. Simultaneous measurement of serum tryptophan and kynurenine by HPLC. Clin Chem 1997; 43: 2424–2426
- Widner B, Leblhuber F, Walli J, Tilz GP, Demel U, Fuchs D. Tryptophan degradation and immune activation in Alzheimer's disease. J Neural Transm 2000; 107: 343–353
- Widner B, Laich A, Sperner-Unterweger B, Ledochowski M, Fuchs D. Neopterin production, tryptophan degradation, and mental depression—what is the link?. Brain Behav Immun 2002; 16: 590–595