Abstract
The purpose of the present study was to elucidate if rats with myocardial infarction manifest altered responsiveness to central cardiovascular effects of low doses of angiotensin II (ANG II), and if ANG II and vasopressin (VP) cooperate in the central regulation of cardiovascular functions at rest and during stress. Conscious Sprague–Dawley rats with myocardial infarction induced by left coronary artery ligation, or sham-ligated (SL) controls were infused intracerebroventricularly with artificial cerebrospinal fluid (aCSF), ANG II, ANG II + VP or ANG II + V1a receptor antagonist (V1ANT) 4 weeks after cardiac surgery. In the infarcted but not in the SL rats, the resting mean arterial blood pressure (MABP) was significantly elevated by infusions of ANG II and ANG II + VP, while infusion of ANG II + V1ANT was not effective. During administration of aCSF, the pressor, and tachycardic responses to an air-jet stressor were significantly greater in the infarcted than in the SL rats. In the SL rats, the pressor responses to the stressor were significantly greater during infusions of ANG II, ANG II + VP and ANG II + V1ANT than during infusion of aCSF. The tachycardic response in the SL rats was enhanced only by the combined infusion of ANG II + VP. In the infarcted rats, the pressor and the tachycardic responses to the stressor were similar in all groups. It is concluded that: (1) under resting conditions the infarcted rats manifest sensitisation to the central pressor effect of ANG II and that this effect depends on concomitant stimulation of V1a VP receptors, (2) central ANG II may enhance the pressor response to an alarming stressor in the SL rats through an action which does not depend on the concomitant stimulation of V1a receptors, (3) the cooperative action of ANG II and VP is required for intensification of the tachycardic response to the alarming stressor in the SL rats and (4) exaggeration of the cardiovascular responses to the alarming stressor in the infarcted rats cannot be further augmented by an additional stimulation of central ANG II receptors or combined stimulation of ANG II and VP receptors.
Introduction
A number of studies have provided evidence that angiotensin II (ANG II) and arginine vasopressin (VP) participate in the regulation of arterial blood pressure at central and peripheral sites (Cowley et al. Citation1983; Ganten et al. Citation1985; Martin et al. Citation1985; Pittman et al. Citation1982; Robinson et al. Citation1999; Szczepanska-Sadowska Citation2006). There is a general consensus that central administration of these peptides heightens the sympathetic drive and exerts significant pressor effects (DiBona Citation1999; Ganten et al. Citation1985; Janiak et al. Citation1989; Łoń et al. Citation1996; Noszczyk et al. Citation1993; Paczwa et al. Citation1997). In addition, increasing evidence indicates that ANG II may be involved in resetting central cardiovascular control in various models of hypertension, and in post-infarct cardiac failure (Cudnoch-Jedrzejewska et al. Citation2007; Kagiyama et al. Citation2001; Kubo et al. Citation2000; Leenen et al. Citation1999; Szczepanska-Sadowska et al. Citation1998; Wang and Ma Citation2000; Zhang et al. Citation1999; Zhu et al. Citation2004; Zucker et al. Citation2004). With regard to post-infarct cardiac failure, it has been established that a blockade of central AT1 receptors for ANG II and V1a receptors for VP significantly reduces MABP and renal sympathetic nerve activity in rats with a myocardial infarct while the same procedure was not effective in sham-operated rats (Cudnoch-Jedrzejewska et al. Citation2007; Dobruch et al. Citation2005; Leenen et al. Citation1999; Zhang et al. Citation1999). In comparison with sham-ligated (SL) rats the infarcted rats manifested also exaggerated cardiovascular responses to the alarming stressor that could be normalised by the intracerebroventricular (i.c.v.) administration of an AT1 or V1a antagonist (Cudnoch-Jedrzejewska et al. Citation2007; Dobruch et al. Citation2005; Zhang et al. Citation1999). Accordingly, it was concluded that myocardial infarction results in an enhanced activation of those central AT1 and V1a receptors which participate in cardiovascular regulation under resting conditions and during stress (Cudnoch-Jedrzejewska et al. Citation2007; Dobruch et al. Citation2005; Zhang et al. Citation1999). In our previous studies, we described sensitisation to the central pressor action of VP, and an interaction between AT1 and V1a receptors in central cardiovascular control in infarcted rats (Cudnoch-Jedrzejewska et al. Citation2007; Dobruch et al. Citation2005). Thus far, cardiovascular studies conducted on infarcted rats have focused only on the role of the central AT1 receptors (Cudnoch-Jedrzejewska et al. Citation2007; Zhang et al. Citation1999). However, it is known that ANG II also exerts some neuroregulatory effects via AT2 receptors and (after metabolism to ANG IV) by AT4 receptors (Armando et al. Citation2002; Bottari et al. Citation1993, Braszko Citation2002; Dumont et al. Citation1999; Hogarty et al. Citation1994; Toney and Porter Citation1993; Wright and Harding Citation1998, Citation2004). Furthermore, ANG II may be metabolised to other peptides, whose action in central regulation of the cardiovascular functions has not been fully defined. In the present study, we aimed to find out whether the sensitivity of the infarcted rats to the central cardiovascular effects of ANG II is altered. Also, we wanted to elucidate whether ANG II and VP cooperate in the regulation of cardiovascular parameters at rest and during stress when their concentration in the brain is elevated simultaneously. Addressing these questions appeared to be essential for several reasons. First, both of these neuropeptides are involved in the regulation of such vital functions as thirst and sodium appetite, and in the release of the hypothalamic and pituitary hormones (Armando et al. Citation2002; Carrasco and Van de Kar Citation2003; Fitzsimons Citation1979; Kovacs and Sawchenko Citation1996; Landgraf Citation2006; Mayorov et al. Citation2004; Saad and Camargo Citation2003; Saavedra et al. Citation2005, Citation2006). In addition, an increased concentration of ANG II and VP in the cerebrospinal fluid or specific brain structures has been reported in dogs and rats after haemorrhage and osmotic stimulation (Cameron et al. Citation1985; Demotes-Mainard et al. Citation1986; Szczepanska-Sadowska et al. Citation1983; Veltmar et al. Citation1992). Thus, the above stimulators may produce an additional challenge for ANG II and VP-responding cardiovascular regulatory neurones. Second, ANG II plays an important role in the regulation of VP release (Ganten et al. Citation1985; Hogarty et al. Citation1994; Lee et al. Citation1996; Veltmar et al. Citation1992). There is also some evidence for an increased concentration of ANG II in the cerebrospinal fluid and in the brain of dogs and rats with cardiac failure (Muders et al. Citation2002; Wang and Ma Citation2000; Zucker et al. Citation2004). The specific purpose of the present study was to compare the central cardiovascular effects of i.c.v. infusions of: (1) ANG II alone, (2) ANG II combined with VP and (3) ANG II combined with a V1a vasopressin receptors antagonist in infarcted and SL rats under resting conditions, and after application of an air-jet stressor. Low rates of ANG II and VP infusions were chosen in order to simulate the release of these peptides at the rate that is sub-pressor in SL rats.
Methods
Animals and surgery
The investigation was carried out on four groups of infarcted and four groups of SL male Sprague–Dawley rats (SPRD/Mől/Lod) which entered the study aged 10–12 weeks. The rats were bred in the Department of Animal Breeding of the Medical University of Warsaw. They were maintained on a 12 h light/12 h dark cycle in a room with regulated temperature (range 22–25°C). Between the experimental sessions, the rats had free access to water and rodent dry pellet diet containing 0.3% NaCl. The experimental protocols were approved by the Ethical Committee on Animal Research of the Medical University of Warsaw. Each rat was subjected to the following procedures: (1) ligation or sham ligation of a coronary artery at the age of 10–12 weeks, (2) implantation of a guide tube leading to a lateral cerebral ventricle at the age of 14–16 weeks and (3) implantation of an arterial catheter at the age of 15–17 weeks. All surgical procedures were performed under chloral hydrate anaesthesia (36 mg/100 g body weight, i.p.).
Myocardial infarction and sham ligation of the coronary artery
The myocardial infarct was produced according to the procedure described previously (Dobruch et al. Citation2005; Selye et al. Citation1960). In brief, the thorax was opened between the fourth and the fifth intercostal space while ventilation was maintained by intermittent ventilation from a small rubber balloon connected with the rat's nose by a plastic tube. The left coronary artery was ligated and the heart was replaced into the thoracic cavity. The thorax was closed and normal ventilation established. Control rats were subjected to the same procedure, but the left coronary artery was not ligated. After surgery all rats received analgesic (buprenorphin sulphate, Polfa; 3 mg/100 g body weight, i.p.) and antibiotic (Taromentin, Polfa, 3 mg/100 g body weight, i.p.) and placed in separate home cages. The rate of survival of the infarcted and SL rats was 53 and 97%, respectively.
Implantation of the guide tube into a lateral cerebral ventricle
Four weeks after coronary artery ligation or SL, a guide tube (o.d. 0.81 mm, MIFAM) was inserted into the left lateral ventricle using stereotaxic apparatus (Kopff). The coordinates were: 1.3 mm posterior to bregma, 2 mm lateral to the midline and 4.5 mm below the surface of the skull (Paxinos and Watson Citation1986). The details of the procedure are described elsewhere (Dobruch et al. Citation2005). The tube was secured with acrylic cement (Duracryl, SPOFA-DENTAL) and closed with a stainless steel stylet (o.d. 0.46 mm). The rat was given Taromentin (Polfa, 3 mg/100 g body weight, i.p.) and returned to the home cage.
Implantation of the catheter in a femoral artery
The arterial catheter was inserted 24–48 h after implantation of the LV guide tube. The arterial line for MABP measurements consisted of the intraarterial part made from 3.5 to 4.0 cm-long tubing (i.d. 0.5 mm, o.d. 0.8 mm) and the external polyvinyl tubing (Scientific Commodities, Inc.). The catheter was filled with 0.9% NaCl containing 500 IU heparin/ml. The intraarterial part was inserted into the aorta through the femoral artery 2.0 cm below the renal arteries. The external tubing was tunnelled under the skin and exteriorized on the neck. After surgery, the skin was closed with sutures and the rat was given Taromentin (Polfa, 3 mg/100 g body weight, i.p.) and returned to the home cage. Twelve hours after anaesthesia, the rats were moving freely in the cage without apparent discomfort.
Plan of experiments
In each experimental session, 15–30 min were allowed for the stabilisation of mean arterial blood pressure (MABP) and heart rate. This was followed by a 10-min period of MABP and heart rate recording before the onset of i.c.v. infusion. Subsequently, the stylet was removed from the guide tube and the infusing cannula was inserted through the guide tube. The infusing cannula was 0.5–1.0 mm longer than the length of the guide tube so that it reached the lumen of the lateral ventricle. The i.c.v. infusion was started and MABP and heart rate were recorded continuously (1) for 50 min under resting conditions and (2) during the application of an alarming stressor (air-jet) and over the next 10 min. The infusions were performed by means of a microsyringe pump (Harvard 22 Infusion Pump, Harvard Smith Natick, MA). The composition of artificial cerebrospinal fluid (aCSF) was: Na+, − 156.0 mmol/l; K+, − 3.0 mmol/l; Ca2 + , − 1.5 mmol/l; Cl− , − 147.0 mmol/l and HCO3− , − 15.0 mmol/l. The alarming air-jet stressor was produced using our own modification (Dobruch et al. Citation2005) of the technique described by Zhang et al. (Citation1999). Compressed air (10 atm) was blown onto the top of the rat's head for 1 s via a laboratory made device. The air jet was applied only once to each rat to avoid adaptation. The magnitude of the cardiovascular response to the stressor was evaluated by measuring the maximum increases in MABP and heart rate from the level immediately preceding application of the air jet.
Experimental series
Four series of experiments were performed on 34 infarcted and 35 SL rats. In Series 1, eight infarcted and eight SL rats received an i.c.v. infusion of aCSF alone (83 nl/min). In Series 2, nine infarcted and nine SL rats received i.c.v. infusion of aCSF for 10 min and then i.c.v. infusion of ANG II (Sigma, 1.7 ng/min, i.e. 1.62 pmol/min) in aCSF (83 nl/min) until the end of the experiment. In Series 3, eight infarcted and nine SL rats received an i.c.v. infusion of aCSF for 10 min and subsequently a combined i.c.v. infusion of ANG II (1.7 ng/min) with VP (1.7 ng/min, i.e. 1.54 pmol/min) in aCSF (83 nl/min) until the end of the experiment. In Series 4, nine infarcted and nine SL rats received an i.c.v. infusion of aCSF followed by a combined i.c.v. infusion of ANG II (1.7 ng/min) with vasopressin V1a receptor antagonist {d(CH2)5[Tyr(Me)2-Ala-NH29]AVP; V1ANT, 3.6 ng/min, i.e. 3.1 pmol/min} until the end of the experiment. d(CH2)5[Tyr(Me)2Ala-NH29]AVP was kindly provided by Maurice Manning (Medical College of Ohio).
Measurements
During each experimental session, MABP and heart rate were recorded continuously by means of a BIOPAC system (MP100, Santa Barbara, CA, USA). The system determines MABP as the area under the arterial pressure curve divided by the cardiac cycle duration and calculates heart rate (beats/min) from the number of systolic pressure peaks. In eight infarcted and nine SL rats, the experiments were concluded with measurements of the end-diastolic pressure in the left cardiac ventricle (LVEDP). For this the rats were anaesthetised (chloral hydrate 36 mg/100 g body weight, i.p.) and a catheter (Dural Plastics and Engineering, Auburn, Australia (i.d. 0.5 mm; o.d. 0.8 mm)) was inserted into the left cardiac ventricle through the right carotid artery and the aortic arch to measure LVEDP. The data were collected by means of the BIOPAC MP100 system.
Post-mortem examination
After the experiments, the rats were given 100 mg/100 g body weight, i.p. of chloral hydrate and the heart was excised from the thorax, washed with saline and dried with a tissue. The atria were removed and the left ventricle wall (together with the septum) was separated from the right ventricle wall. The infarct size was determined by planimetry as described by Leenen et al. (Citation1999), with some minor modifications (Dobruch et al. Citation2005), and expressed as a percentage of the total left ventricle surface. In addition, the heart was examined histologically to confirm the presence of an infarct scar according to the procedure described previously (Cudnoch-Jędrzejewska et al. Citation2005; Dobruch et al. Citation2005). To verify whether the i.c.v. cannula was reaching the lumen of the cerebroventricular system Evans blue (5 μl) was injected through the same cannula that was used for i.c.v. infusions and the brain was sagittally sectioned to inspect the ventricular system for the presence of the dye. The abdomen was opened and the aorta was inspected to check that the intraarterial catheter was located below the renal arteries and that the aorta was not obstructed.
Statistical analysis
The significance of changes in the cardiovascular parameters was evaluated using Statistica software (release 7.1). In the statistical analysis, 5-min averages of MABP and heart rate were used to assess changes in cardiovascular parameters during the resting period. The Shapiro–Wilk test was used to find out whether the data follow a normal distribution. Because no significant departures of data from normality were found, ANOVA tests were used. Significance of changes in MABP and heart rate from baseline under resting conditions was determined by three-way ANOVA with 2 levels of rats (SL versus infarcted rats) × 4 levels of experimental design (aCSF, ANG II, ANG II + VP, ANG II + V1ANT) × 8 levels of repeated measurements. This was followed by two-way ANOVA with four levels of experimental design and eight levels of repeated measurements as factors, performed separately for the infarcted and the SL rats (Curran-Everett and Benos Citation2004; Ludbrook Citation1994). Significance of MABP fluctuations in time during i.c.v. infusions of aCSF, ANG II, ANG II + VP and ANG II + V1ANT in the individual groups of experiments was assessed by one-way ANOVA followed by the least significance difference method (Winer et al. Citation1991). To evaluate MABP and heart rate responses to the stressor, the maximum changes of these parameters from the level immediately preceding the application of the stressor was used in the statistical analysis. The significance of differences between the air-jet stressor-induced maximum changes in the cardiovascular parameters in different groups of experiments was evaluated with the least significance difference method. Significance was accepted at the level of P < 0.05. All values presented in the text, tables and figures are means ± SE.
Results
The infarct size expressed as the percentage of the total left ventricle surface was similar in all groups of the infarcted rats and was: 35.04 ± 2.04% in the aCSF group, 33.28 ± 1.92% in the ANG II group, 32.88 ± 2.04% in the ANG II + VP group and 35.72 ± 1.92% in the group infused with ANG II+V1ANT.
Cardiovascular Effects Of I.c.v. Infusions Of Acsf, Ang Ii, Ang Ii With Vp Or Ang Ii With V1ant Under Resting Conditions
MABP
Before the onset of the i.c.v. infusion resting MABP did not differ in the individual groups of experiments and was 125 ± 1.5 mm Hg in the aCSF SL group (n = 8), 122 ± 1.9 mm Hg in the aCSF infarct group (n = 8), 125 ± 1.6 mm Hg in the ANG II SL group (n = 9), 123 ± 2.0 mm Hg in the ANG II infarct group (n = 9), 125 ± 1.8 mm Hg in the ANG II + VP SL group (n = 9), 121 ± 2.2 mm Hg in the ANG II + VP infarct group (n = 8), 126 ± 0.9 mm Hg in the ANG II + V1ANT SL group (n = 9), and 122 ± 2.2 mm Hg in the ANG II + V1ANT infarct group (n = 9).
The overall three-way ANOVA on the changes of MABP from baseline for all groups of rats revealed a significant interaction of the experimental group with MABP [(F(7,61) = 4.808; P < 0.001]. Separate analysis performed on the SL rats showed that under resting conditions changes in MABP in individual groups did not differ from each other. Similarly, one-way ANOVA performed on individual groups of experiments revealed that in the SL rats the i.c.v. infusions of aCSF, ANG II alone, and ANG II together with VP or with V1ANT did not affect the resting MABP in comparison to baseline ().
Figure 1 Changes in mean arterial blood pressure (MABP) and heart rate during intracerebroventricular (i.c.v.) administration of artificial cerebrospinal fluid (aCSF, control) and angiotensin II (ANG II) in SL and infarcted rats at rest. #Significant changes in MABP when compared with baseline during i.c.v. infusion of ANG II [F(7,56) = 4.823; P < 0.001], *significant difference in MABP changes between i.c.v. infusion of ANG II and aCSF at the corresponding time {interaction between ANG II infarct and aCSF infarct: [F(7,110) = 2.767; P < 0.05], difference between ANG II infarct and aCSF infarct: [F(1,15) = 21.710; P < 0.001]}. n = 8–9 rats per group.
![Figure 1 Changes in mean arterial blood pressure (MABP) and heart rate during intracerebroventricular (i.c.v.) administration of artificial cerebrospinal fluid (aCSF, control) and angiotensin II (ANG II) in SL and infarcted rats at rest. #Significant changes in MABP when compared with baseline during i.c.v. infusion of ANG II [F(7,56) = 4.823; P < 0.001], *significant difference in MABP changes between i.c.v. infusion of ANG II and aCSF at the corresponding time {interaction between ANG II infarct and aCSF infarct: [F(7,110) = 2.767; P < 0.05], difference between ANG II infarct and aCSF infarct: [F(1,15) = 21.710; P < 0.001]}. n = 8–9 rats per group.](/cms/asset/c1e047d9-cca1-4d27-99d9-3b0a6ad49968/ists_a_279482_f0001_b.gif)
Figure 2 Changes in mean arterial blood pressure (MABP) and heart rate during intracerebroventricular (i.c.v.) administration of artificial cerebrospinal fluid (aCSF, control) and infusion of angiotensin II (ANG II) with vasopressin (VP) (ANG II + VP) in sham-ligated and infarcted rats. #Significant change in MABP compared with baseline during i.c.v. infusion of ANG II + VP [F(7,49) = 6.282; P < 0.001], *significant difference in MABP changes between i.c.v. infusion of ANG II + VP and aCSF at the corresponding time {interaction between ANG II + VP infarct and aCSF infarct: [F(7,98) = 4.218; P < 0.001], difference between ANG II + VP infarct and aCSF infarct [(1,14) = 11.315; P < 0.01]}. For ease of comparison changes in MABP during infusion of aCSF in the infarcted and sham-ligated rats are redrawn from . n = 8–9 rats per group.
![Figure 2 Changes in mean arterial blood pressure (MABP) and heart rate during intracerebroventricular (i.c.v.) administration of artificial cerebrospinal fluid (aCSF, control) and infusion of angiotensin II (ANG II) with vasopressin (VP) (ANG II + VP) in sham-ligated and infarcted rats. #Significant change in MABP compared with baseline during i.c.v. infusion of ANG II + VP [F(7,49) = 6.282; P < 0.001], *significant difference in MABP changes between i.c.v. infusion of ANG II + VP and aCSF at the corresponding time {interaction between ANG II + VP infarct and aCSF infarct: [F(7,98) = 4.218; P < 0.001], difference between ANG II + VP infarct and aCSF infarct [(1,14) = 11.315; P < 0.01]}. For ease of comparison changes in MABP during infusion of aCSF in the infarcted and sham-ligated rats are redrawn from Figure 1. n = 8–9 rats per group.](/cms/asset/439c9f98-030d-4ba1-bbb6-e6aef1d49c5a/ists_a_279482_f0002_b.gif)
Figure 3 Changes in mean arterial blood pressure (MABP) and heart rate during intracerebroventricular (i.c.v.) administration of artificial cerebrospinal fluid (aCSF, control) and ANG II with V1a receptor antagonist {d(CH2)5[Tyr(Me)2-Ala-NH29]AVP; V1ANT}in the sham-ligated and the infarcted rats at rest. For ease of comparison changes in MABP during aCSF infusion in the infarcted and the sham-ligated rats are redrawn from . n = 8–9 rats per group.
![Figure 3 Changes in mean arterial blood pressure (MABP) and heart rate during intracerebroventricular (i.c.v.) administration of artificial cerebrospinal fluid (aCSF, control) and ANG II with V1a receptor antagonist {d(CH2)5[Tyr(Me)2-Ala-NH29]AVP; V1ANT}in the sham-ligated and the infarcted rats at rest. For ease of comparison changes in MABP during aCSF infusion in the infarcted and the sham-ligated rats are redrawn from Figure 1. n = 8–9 rats per group.](/cms/asset/936a3139-17ed-4171-988f-e3c689d87f63/ists_a_279482_f0003_b.gif)
The infarcted rats were much more sensitive to central pressor action of ANG II (). The infusion of ANG II and ANG II together with VP in the infarcted rats produced significant increases in MABP from baseline {ANG II: [F(7,56) = 4.823; P < 0.001] and ANG II + VP: [F(7,49) = 6.282; P < 0.001]} ( and ). Significant differences were found between: (1) the infarct aCSF and the infarct ANG II groups {interaction: [F(7,11) = 2.767; P < 0.05]; difference between the groups: [F(1,15) = 21.710; P < 0.001]}, (2) the infarct aCSF and the infarct ANG II + VP groups {interaction: [F(7,98) = 4.218); P < 0.001]; difference between the groups [F(1,14) = 11.315; P < 0.01]} and (3) the ANG II + VP SL and the ANG II + VP infarct groups {interaction: [F(7,105) = 2.166; P < 0.05]; difference between the groups: [F(1,15) = 5.535; P < 0.05]}. Changes in blood pressure found during infusion of ANG II alone and ANG II together with VP did not differ significantly ( and ). The combined infusion of ANG II and V1ANT did not influence the resting blood pressure in infarcted rats. Changes in MABP during the combined infusion of ANG II and V1ANT were significantly lower than those during the infusion of ANG II alone ( and ) {difference between the groups [F(1,16) = 10.117; P < 0.001]}. A significant difference for changes in the resting MABP was also found between the groups infused with ANG II+V1ANT and ANG II + VP {interaction between the groups: [F(7,105) = 3.612; P < 0.01], difference between the groups: [F(1,15) = 9.067; P < 0.01]. In contrast, changes in MABP during infusion of ANG II + V1ANT did not differ from those found during infusion of aCSF.
Heart rate
Before the onset of i.c.v. infusion, resting heart rate was similar in individual groups of experiments and was: 375 ± 9.8 beats/min in the aCSF SL group (n = 8), 384 ± 11.1 beats/min in the aCSF infarct group (n = 8), 379 ± 9.3 beats/min in the ANG II SL group (n = 9), 381 ± 8.7 beats/min in the ANG II infarct group (n = 9), 377 ± 6.1 beats/min in the ANG II + VP SL group (n = 9), 379 ± 7.0 beats/min in the ANG II + VP infarct group (n = 8); 379 ± 7.0 beats/min in the ANG II + V1ANT SL group (n = 9), and 381 ± 9.3 beats/min in the ANG II + V1ANT infarct group (n = 9). Under resting conditions, heart rate was not affected by i.c.v. infusions of aCSF, ANG II, ANG II + VP or ANG II+V1ANT () either in the SL or in the infarcted rats.
Cardiovascular responses to the alarming stressor during i.c.v. infusions of aCSF, ANG II, ANG II with VP and ANG II with V1ANT
Mean arterial blood pressure
MABP immediately before the alarming stressor (air jet) was similar in the individual groups of experiments [126 ± 1.5mm Hg in the aCSF SL group (n = 8); 126 ± 0.9mm Hg in the aCSF infarct group (n = 8); 127 ± 1.7 mm Hg in the ANG II SL group (n = 9); 127 ± 1.8 mm Hg in the ANG II infarct group (n = 9); 126 ± 1.9 mm Hg in the ANG II + VP SL group (n = 9); 128 ± 2.2 mm Hg in the ANG II+VP infarct group (n = 8); 125 ± 1.4 mm Hg in the ANG II + V1ANT SL group (n = 9) and 122 ± 2.1mm Hg in the ANG II + V1ANT infarct group (n = 9)].
In each experiment, MABP started to increase immediately after the application of the air-jet stressor. The maximum increase in MABP was observed after 6–8 s from the onset of the stimulus. Factorial ANOVA performed on combined data from the infarcted and the SL rats did not show significant differences in the changes of MABP after application of the alarming stressor in the whole population of rats. However, the least difference test revealed significant differences in changes in MABP between the individual groups of the SL rats. In particular, significant differences were found between the aCSF and the ANG II groups (P < 0.05), the aCSF and ANG II + VP groups (P < 0.05) and between the aCSF and ANG II+V1ANT groups (P < 0.05). A significant difference was also found between the aCSF SL and aCSF infarct groups (P < 0.05) ().
Figure 4 Maximum increases in mean arterial blood pressure (MABP) and heart rate evoked by air-jet stress in the infarcted and sham-ligated rats during i.c.v. infusion of artificial cerebrospinal fluid (aCSF), angiotensin II (ANG II), ANG II with vasopressin (VP) and ANG II with VP V1a receptors antagonist {d(CH2)5[Tyr(Me)2-Ala-NH29]AVP; V1ANT}, *significant difference between the corresponding experimental groups, #significant difference between the infarcted and sham-ligated rats (##P < 0.01, #P < 0.05). n = 8–9 rats per group.
![Figure 4 Maximum increases in mean arterial blood pressure (MABP) and heart rate evoked by air-jet stress in the infarcted and sham-ligated rats during i.c.v. infusion of artificial cerebrospinal fluid (aCSF), angiotensin II (ANG II), ANG II with vasopressin (VP) and ANG II with VP V1a receptors antagonist {d(CH2)5[Tyr(Me)2-Ala-NH29]AVP; V1ANT}, *significant difference between the corresponding experimental groups, #significant difference between the infarcted and sham-ligated rats (##P < 0.01, #P < 0.05). n = 8–9 rats per group.](/cms/asset/0997e890-cc93-4235-904b-564e0ab5ff7f/ists_a_279482_f0004_b.gif)
Heart rate
Immediately before application of the air-jet, heart rate did not differ among the groups of SL and infarcted rats [375 ± 9.9 beats/min in the aCSF group (n = 8); 380 ± 11.7 beats/min in the aCSF infarct group (n = 8); 382 ± 8.5 beats/min in the ANG II SL group (n = 9); 382 ± 8.9 beats/min in the ANG II infarct group (n = 9); 377 ± 5.8 beats/min in the ANG II + VP SL group (n = 9); 380 ± 6.9 beats/min in the ANG II + VP infarct group (n = 8); 380 ± 8.2 beats/min in the ANG II + V1ANT SL group (n = 9), 382 ± 8.6 beats/min in the ANG II + V1ANT infarct group (n = 9)]. Application of the air jet caused immediate acceleration of the heart rate in each group of rats. The maximum increase was observed 6–8 s from the start of the air jet. Significant inter-group differences were found for changes in heart rate after application of the alarming stressor {F(7,61) = 6.511; P < 0.001]. The least difference test disclosed significant differences between the aCSF SL and the ANG II + VP SL groups (P < 0.05), the aCSF SL and aCSF infarct groups (P < 0.01), the ANG II SL and ANG II infarct groups (P < 0.01) and the ANG II + VP SL and ANG II + VP infarct groups (P < 0.05) ().
LVEDP determined at the end of the experiments was significantly greater in the infarcted (22.0 ± 1.38mm Hg; n = 8) than in the SL rats (4.3 ± 0.5mm Hg); F(1,15) = 136.58; n = 9; P < 0.001].
Discussion
A major new finding of the present study is the demonstration of significant differences between the central pressor effects of ANG II in rats with a myocardial infarct and control SL rats. The differences were present both in the resting state and after the alarming stressor. Under resting conditions, the infarcted rats manifested significant sensitisation to the central pressor action of ANG II. This effect depended on concomitant stimulation of V1a VP receptors. On the other hand, ANG II and ANG II + VP intensified the cardiovascular responses to the stressor only in the SL rats. In the infarcted rats, the cardiovascular responses to the stressor were significantly greater during the control aCSF infusions than in the SL rats; however, they were not further exaggerated by infusions of ANG II or ANG II + VP.
Central cardiovascular effects of angiotensin II and VP at rest
In the present study, we infused ANG II and VP centrally at the rates that were sub-pressor in the SL controls. In this way, we attempted to simulate an increased release of endogenous ANG II into the brain, such as might occur during chronic stress or osmotic stimulation. The results provide evidence for significant sensitisation of the brain cardiovascular regulatory neurones of the infarcted rats to the pressor action of ANG II under resting conditions. The sensitisation is indicated by the significant increase of MABP in the infarcted rats during i.c.v. infusion of ANG II in comparison with the i.c.v. infusion of aCSF, and by the significant difference between the changes of MABP in the SL and the infarcted groups during infusion of this peptide. Several mechanisms may account for the increased pressor response to ANG II in the infarcted rats. For instance, it may result from a greater number of ANG II receptors in the brain of the infarcted rats as it was shown by Tan et al. (Citation2004). Another possibility may be ANG II-induced generation of some hypertensive mediator(s) in the brain. ANG II is a potent stimulator of VP release (Haack and Möhring Citation1978; Veltmar et al. Citation1992). In our previous study (Dobruch et al. Citation2005), we provided evidence for an increased activation of the brain VP system during the post-infarct state. Therefore, it is likely that the greater pressor response to ANG II in the infarcted rats under resting conditions is related to a greater stimulation of the pressor cardiovascular regulatory neurones by VP. Interestingly, under resting conditions, the combined infusion of VP and ANG II produced a similar pressor effect in the infarcted rats as did infusion of ANG II alone. Taking into account that i.c.v. infusion of VP at the same rate increases MABP in infarcted rats (Dobruch et al. Citation2005) it may be assumed that under resting conditions the pressor responses to low concentrations of ANG II and VP do not potentiate each other and do not summate in the infarcted rats. At present, we suggest that the cooperation of ANG II with VP in infarcted rats involves the neuronal pathway illustrated in (left). Basing on our present and previous results (Dobruch et al. Citation2005, Cudnoch-Jędrzejewska et al. Citation2007) and the studies showing that ANG II stimulates the release of VP (Hogarty et al. Citation1994; Lee et al. Citation1996; Veltmar et al. Citation1992), we propose that angiotensin II AT1 receptors and vasopressin V1a receptors are involved in this cooperation. We also propose (, left) the possibility of modification (augmentation or inhibition) of the sensitising effect by simultaneous action of some other types of ANG II or vasopressin receptors (ATxR, VxR). The sensitisation might be caused also by some other mediators. Especially, an interaction of cytokines with ANG II should be taken into account. Myocardial infarction results in central release of cytokines (Francis et al. Citation2004), while cytokines induce significant sensitisation to the central pressor action of ANG II (Ufnal et al. Citation2006, Żera et al. Citation2006).
Figure 5 Schematic of cooperation between angiotensin and vasopressin in the central regulation of cardiovascular parameters by angiotensin II (ANG II) and vasopressin (VP) based on present and previous results (Dobruch et al. Citation2005; Cudnoch-Jędrzejewska et al. Citation2007). (A) The myocardial infarct increases involvement of ANG II and VP in central regulation of blood pressure at rest via AT1 and V1a receptors. The present results show that VP is necessary to induce sensitisation to central pressor action of ANG II after a myocardial infarction. ANG II may stimulate the cardiovascular pressor neurones directly and indirectly by increasing release of VP. Cooperative action of these two peptides results in the pressor response. (B) ANG II enhances the pressor effect of an alarming stressor in intact rats. The tachycardic response to the stressor is not altered by separate actions of ANG II or VP but is increased by their cooperative action. The effects exerted by ANG II and VP via AT1 and V1a receptors may be modified by stimulation of other receptors (ATxR and VPxR).
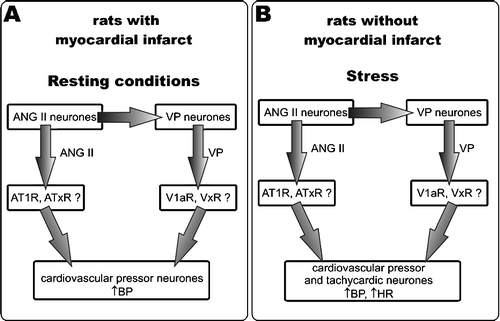
Effect of ANG II on cardiovascular responsiveness to the alarming stressor
The present results confirm other reports in which it was demonstrated that an alarming stressor elicits greater pressor and tachycardic responses in infarcted than in SL rats (Cudnoch-Jedrzejewska et al. Citation2007; Dobruch et al. Citation2005; Zhang et al. Citation1999). Previous studies provided also evidence that differences in the pressor and tachycardic responses to the alarming stressor between infarcted and SL rats can be abolished by blockade of central AT1 and V1a receptors (Cudnoch-Jedrzejewska et al. Citation2007; Dobruch et al. Citation2005; Zhang et al. Citation1999). Accordingly, it was proposed that the increased responsiveness of the cardiovascular regulatory neurones to an alarming stressor during the post-infarct state might be caused by an enhanced activation of central AT1 and V1a receptors (Cudnoch-Jedrzejewska et al. Citation2007; Dobruch et al. Citation2005). The present study reveals that the SL rats respond with significantly greater increases in MABP to the alarming stressor during administration of ANG II than during infusion of aCSF. This finding is in agreement with the previous study of Mayorov and Head (Citation2003) who demonstrated involvement of AT1 receptors within the rostral ventrolateral medulla in regulation of the magnitude and duration of the pressor response to an alarming stressor in normal rabbits. In the present investigation, we demonstrated an interesting phenomenon, namely, that in SL rats the i.c.v. administration of ANG II at the rate that was sub-pressor under resting conditions significantly enhanced the pressor response to the alarming stressor. The same result was obtained during the combined administration of ANG II and VP. This finding indicates that even a small sub-pressor increase in the ANG II concentration in the brain can significantly intensify the pressor responses to the alarming stressor in intact rats. It should be noted that augmentation of the pressor response to stress by ANG II in the SL rats was also present during the combined infusion of V1ANT and ANG II (). Our previous studies (Dobruch et al. Citation2005, Cudnoch-Jędrzejewska et al. Citation2007) strongly suggested that an enhanced stimulation of V1a receptors is involved in the AT1 receptor-mediated exaggeration of cardiovascular responses to stress in the post-infarct state. The present finding suggests that in intact rats ANG II may augment the pressor response using different receptors pathways in which activation of VP V1a receptors does not play a crucial role (). The present results indicate that a simultaneous administration of ANG II and VP elicits the same augmentation of the pressor response to the alarming stressor as infusion of ANG II alone. This finding clearly shows that the pressor action of ANG II is not further augmented by simultaneous administration of VP. Previously, Dobruch et al. (Citation2005) found that an administration of VP does not enhance the pressor response to stress in rats. It is likely that VP may stimulate some other receptors in the brain apart from V1a receptors and in this way limit its own stimulatory effect on those V1a receptors that mediate enhancement of the pressor response to stress. Another possibility is a saturation of VP receptors in the brain of intact rats by the endogenous VP.
The air-jet stressor also significantly increased the heart rate. In agreement with previous studies (Cudnoch-Jedrzejewska et al. Citation2007; Dobruch et al. Citation2005; Zhang et al. Citation1999) the cardioacceleratory response was significantly greater in infarcted than in SL rats. A comparison of the tachycardic responses to the stressor during infusions of aCSF, ANG II, ANG II + VP and ANG II + V1ANT reveals that administration of ANG II alone was not effective either in the SL or the infarcted rats. However, a simultaneous application of ANG II and VP significantly elevated the tachycardic response to stressing in the SL rats. Thus, the cooperative action of small doses of ANG II and VP can effectively augment the cardioacceleratory response to the alarming stressor even if separate application of these two peptides is not effective. The putative cooperation between ANG II and VP in the SL rats is depicted in (right side).
An intriguing finding of the present study was that the same i.c.v. infusions of ANG II or ANG II with VP that were effective in the SL rats did not influence the cardiovascular responses to the alarming stressor in the infarcted rats. This might have resulted from prior elevation of MABP in infarcted rats by infusion of ANG II in the resting state, although this effect of ANG II was rather modest and heart rate was unaffected. Instead, we hypothesize that during the post-infarct state there may be an up-regulation of mechanisms protecting against an excessive elevation of blood pressure by ANG II. Also, the angiotensin receptors may be saturated by endogenous ANG II. Infusion of ANG II together with V1ANT abolished the significant difference in the stressor-induced acceleration of heart rate between the infarcted and SL rats. This finding confirms our previous results that the stimulation of V1a receptors plays an essential role in intensifying the tachycardic response to the alarming stressor during the post-infarct state.
General discussion
The present study reveals that even a small increase of the ANG II concentration in the brain may have a significant influence on regulation of the cardiovascular system and that this influence is altered after myocardial infarction. Sensitisation to the central pressor action of ANG II in infarcted rats implies that the release of ANG II into the brain in an amount that is sub-pressor in intact rats may increase MABP after myocardial infarction. Also, only in intact rats, ANG II may augment cardiovascular responses to an alarming stressor, even at a concentration too low to alter MABP at rest. The cooperation of VP and ANG II differed at rest and during stress, suggesting that different pools of neurones or mediators participate in sensitisation to the central pressor action of ANG II at rest in infarcted rats and in modulation of the cardiovascular responses to an alarming stressor in intact rats. At rest, sensitisation to the central pressor action of ANG II in infarcted rats strongly depended on concomitant stimulation of V1a VP receptors. Also, at rest ANG II acts in the brain to stimulate the pressor but not the cardioacceleratory pathway. ANG II-induced enhancement of the pressor responses to stress does not crucially depend on VP and V1a receptors, but the cardioacceleratory pathway requires action of both ANG II and VP.
In summary, the present study provides evidence for sensitisation in infarcted rats to central pressor action of low doses of ANG II at rest, and for differential influence of ANG II on pressor and tachycardic responses to an alarming stressor in infarcted and intact rats.
Acknowledgements
The authors are indebted to Professor Maurice Manning from the Department of Biochemistry and Molecular Biology from the Medical College of Ohio, Toledo for the generous supply of d(CH2)5[Tyr(Me)2-Ala-NH29]AVP, and to Mrs Marzanna Tkaczyk and Mr Marcin Kumosa for their valuable technical assistance. This study obtained the financial support from the Medical University of Warsaw (grant 1MA/W2/2005-2006) and from the Ministry of Education and Science (grant 2P05A 182 29).
References
- Armando I, Terron JA, Falcon-Neri A, Takeshi I, Hauser W, Inagami T, Saavedra JM. Increased angiotensin II AT(1) receptor expression in paraventricular nucleus and hypothalamic-pituitary-adrenal axis stimulation in AT(2) receptor gene disrupted mice. Neuroendocrinology 2002; 76: 137–147
- Bottari SP, De Gasparo M, Steckelings UM, Levens NR. Angiotensin II receptor subtypes: Characterization, signalling, mechanisms, and possible physiological implications. Front Neuroendocrinol 1993; 14: 123–171
- Braszko JJ. AT2 but not AT1 receptor antagonism abolishes angiotensin II increase of the acquisition of conditioned avoidance responses in rats. Behav Brain Res 2002; 131: 79–86
- Cameron V, Espiner EA, Nicholls MG, Donald RA, Mac Farlane MR. Stress hormones in blood and cerebrospinal fluid of conscious sheep: Effect of hemorrhage. Endocrinology 1985; 116: 1460–1465
- Carrasco GA, Van de Kar LD. Neuroendocrine pharmacology of stress. Eur J Pharmacol 2003; 463: 235–272
- Cowley AW, Jr., Quillen EW, Jr., Skelton MM. Role of vasopressin in cardiovascular regulation. Fed Proc 1983; 42: 3170–3176
- Cudnoch-Jedrzejewska A, Szczepanska-Sadowska E, Dobruch J, Morton M, Koperski Ł, Wasiutynski A, Wsół A, Kowalewski S. Fluid consumption, electrolyte excretion and heart remodeling in rats with myocardial infarct maintained on regular and high sodium intake. J Physiol Pharmacol 2005; 56: 599–610
- Cudnoch-Jedrzejewska A, Dobruch J, Puchalska L, Szczepanska-Sadowska E. Interaction of AT1 receptors and V1a receptors-mediated effects in the central cardiovascular control during the post-infarct state. Regul Pept 2007; 142: 86–94
- Curran-Everett D, Benos DJ. Guidelines for reporting statistics in journals published by the American Physiological Society. Physiol Genomics 2004; 18: 249–251
- Demotes-Mainard J, Chauveau J, Rodriguez F, Vincent JD, Poulain DA. Septal release of vasopressin in response to osmotic, hypovolemic and electrical stimulation in rats. Brain Res 1986; 381: 314–321
- DiBona GF. Central sympathoexcitatory actions of angiotensin II: Role of type 1 angiotensin II receptors. J Am Soc Nephrol 1999; 11: S90–S94
- Dobruch J, Cudnoch-Jedrzejewska A, Szczepanska-Sadowska E. Enhanced involvement of brain vasopressin V1 receptors in cardiovascular responses to stress in rats with myocardial infarction. Stress 2005; 8: 273–284
- Dumont EC, Rafrafi S, Laforest S, Drolet G. Involvement of central angiotensin receptors in stress adaptation. Neuroscience 1999; 93: 877–884
- Fitzsimons JT. The physiology of thirst and sodium appetite. Cambridge University Press, Cambridge 1979; 128–265
- Francis J, Zhang ZH, Weiss RM, Felder R. Neural regulation of the proinflamatory cytokine response to acute myocardial infarction. Am J Physiol Heart Circ Physiol 2004; 287: H791–H797
- Ganten D, Unger T, Lang RE. The dual role of angiotensin and vasopressin as plasma hormones and neuropeptides in cardiovascular regulation. J Pharmacol 1985; 16(suppl II)51–68
- Haack D, Mőhring J. Vasopressin-mediated blood pressure response to intraventricular injection of angiotensin II in the rat. Pflügers Arch 1978; 373: 167–173
- Hogarty DC, Tran DN, Phillips MI. Involvement of angiotensin receptor subtypes in osmotically induced release of vasopressin. Brain Res 1994; 637: 126–132
- Janiak P, Kasson BG, Brody MJ. Central vasopressin raises arterial pressure by sympathetic activation and vasopressin release. Hypertension 1989; 13: 935–940
- Kagiyama S, Varela A, Phillips MI, Galli SM. Antisense inhibition of brain rennin-angiotensin system decreased blood pressure in chronic 2-kidney, 1 clip hypertensive rats. Hypertension 2001; 37: 371–375
- Kovacs KJ, Sawchenko PE. Sequence of stress-induced alterations in indices of synaptic and transcriptional activation in parvocellular neurosecretory neurons. J Neurosci 1996; 16: 262–273
- Kubo T, Yamaguchi H, Tsujimura M, Hagiwara Y, Fukumori R. Angiotensin system in the anterior hypothalamic area is involved in the maintenance of hypertension in spontaneously hypertensive rats. Brain Res Bull 2000; 52: 291–296
- Landgraf R. The involvement of the vasopressin system in stress-related disorders. CNS Neurol Disord Drug Targets 2006; 5: 167–179
- Lee MA, Bohm M, Paul M, Bader M, Ganten U, Ganten D. Physiological characterization of the hypertensive transgenic rat TGR(mREN2)27. Am J Physiol 1996; 270: E919–E929
- Leenen FHH, Yuan B, Huang BS. Brain “ouabain” and angiotensin II contribute to cardiac dysfunction after myocardial infarction. Am J Physiol Heart Circ Physiol 1999; 277: H1786–H1792
- Łoń S, Szczepańska-Sadowska E, Szczypaczewska M. Evidence that centrally released arginine vasopressin is involved in central pressor action of angiotensin II. Am J Physiol Heart Circ Physiol 1996; 270: H167–H173
- Ludbrook J. Repeated measurements and multiple comparisons in cardiovascular research. Cardiovasc Res 1994; 28: 303–311
- Martin SM, Malkinson TJ, Veale WL, Pittman QJ. The action of centrally administered arginine vasopressin on blood pressure in the conscious rabbits. Brain Res 1985; 348: 137–145
- Mayorov DN, Head GA. AT1 receptors in the RVLM mediate pressor responses to emotional stress in rabbits. Hypertension 2003; 41: 1168–1173
- Mayorov DN, Head GA, De Matteo R. Tempol attenuates excitatory actions of angiotensin II in the rostral ventrolateral medulla during emotional stress. Hypertension 2004; 44: 101–106
- Muders F, Riegger GA, Bahner U, Palkovits M. The central vasopressinergic system in experimental left ventricular hypertrophy and dysfunction. Prog Brain Res 2002; 139: 275–279
- Noszczyk B, Łoń S, Szczepańska-Sadowska E. Central cardiovascular effects of AVP and AVP analogs with V1, V2 and “V3” agonistic or antagonistic properties in conscious dog. Brain Res 1993; 610: 115–126
- Paczwa P, Budzikowski AS, Szczepańska-Sadowska E. Enhancement of central pressor effect of AVP in SHR and WKY rats by intracranial N(G)-nitro-l-arginine. Brain Res 1997; 748: 51–61
- Paxinos G, Watson C. The rat brain in stereotaxic coordinates. Academic Press, New York 1986
- Pittman QJ, Lawrence D, McLean L. Central effects of arginine vasopressin on blood pressure in rats. Endocrinology 1982; 110: 1058–1060
- Robinson MM, McLean GP, Thurnhorst RL, Johnson AK. Interactions of the systemic and brain renin–angiotensin systems in the control of drinking and the central mediation of pressor responses. Brain Res 1999; 842: 55–61
- Saad WA, Camargo LA. Influence of angiotensin II receptor subtypes of the paraventricular nucleus on the physiological responses induced by angiotensin II injection into the medial septal area. Arq Bras Cardiol 2003; 80: 396–405
- Saavedra JM, Ando H, Armando I, Baiardi G, Bregonzio C, Juorio A, Macova M. Anti-stress and anti-anxiety effects of centrally acting angiotensin II AT1 receptor antagonists. Regul Pept 2005; 128: 227–238
- Saavedra JM, Armando I, Bregonzio C, Juorio A, Macova M, Pavel J, Sanchez-Lemus E. A centrally acting anxiolytic angiotensin II AT1 receptor antagonist prevents the isolation stress-induced decrease in cortical CRF1 receptor and benzodiazepine binding. Neuropsychopharmacology 2006; 31: 1123–1134
- Selye H, Bajusz E, Grasso S, Mendell P. Simple techniques for the surgical occlusion of coronary vessels in the rat. Angiology 1960; 11: 398–407
- Szczepanska-Sadowska E. Neuropeptides in neurogenic disorders of the cardiovascular control. J Physiol Pharmacol 2006; 57(suppl 11)31–59
- Szczepanska-Sadowska E, Gray D, Simon-Oppermann C. Vasopressin in blood and third ventricle CSF during dehydration, thirst, and hemorrhage. Am J Physiol 1983; 245: R549–R555
- Szczepanska-Sadowska E, Paczwa P, Łoń S, Ganten D. Increased pressor function of central vasopressinergic system in hypertensive renin transgenic rats. J Hypertens 1998; 16: 1505–1514
- Tan J, Wang H, Leenen FHH. Increases in brain and cardiac AT1 receptor and ACE densities after myocardial infarct in rats. Am J Physiol Heart Circ Physiol 2004; 286: H1665–H1671
- Toney GM, Porter JP. Effects of blockade of AT1 and AT2 receptors in brain on the central angiotensin II pressor response in conscious spontaneously hypertensive rats. Neuropharmacology 1993; 32: 581–589
- Ufnal M, Dudek M, Żera T, Szczepanska-Sadowska E. Centrally administered interleukin-1beta sensitizes to the central pressor action of angiotensin II. Brain Res 2006; 1100: 64–72
- Veltmar A, Culman J, Qadri F, Rascher W, Unger T. Involvement of adrenergic and angiotensinergic receptors in the paraventricular nucleus in the angiotensin II-induced vasopressin release. J Pharmacol Exp Ther 1992; 263: 1253–1260
- Wang W, Ma R. Cardiac sympathetic afferent reflexes in heart failure. Heart Fail Rev 2000; 5: 57–71
- Winer BJ, Brown DR, Michels KM. Statistical principles in experimental Design. McGraw-Hill, Inc, New York 1991; 1–1057
- Wright J, Harding JW. Brain angiotensin receptor subtypes in the control of physiological and behavioral responses. Neurosci Biobehav Rev 1998; 18: 21–53
- Wright J, Harding JW. The brain angiotensin system and extracellular matrix molecules in neural plasticity, learning and memory. Progr Neurobiol 2004; 72: 263–293
- Żera T, Ufnal M, Szczepanska-Sadowska E. Tumor necrosis factor-alpha sensitizes to pressor action of centrally administered angiotensin II. J Physiol Pharmacol 2006; 57(suppl 2)253
- Zhang W, Huang BS, Leenen FHH. Brain renin-angiotensin system and sympathetic hyperactivity in rats after myocardial infarction. Am J Physiol Heart Circ Physiol 1999; 276: H1608–H1615
- Zhu GQ, Gao L, Patel KP, Zucker IH, Wang W, Zucker IH, Wang W. ANG II in the paraventricular nucleus potentates the cardiac sympathetic afferent reflex in rats with heart failure. J Appl Physiol 2004; 97: 1746–1754
- Zucker IH, Schultz HD, Li Y-F, Wang Y, Wang W, Patel KP. The origin of sympathetic outflow in heart failure: The role of angiotensin II and nitric oxide. Progr Biophys Mol Biol 2004; 84: 217–232