Abstract
Corticotrophin-releasing hormone (CRH) plays a pivotal role in the suppression of the gonadotrophin-releaning hormone (GRH) pulse generator in response to stress and intracerebroventricular (icv) administration of calcitonin gene-related peptide (CGRP). We have previously shown both CRH receptor subtypes, CRH-R1 and CRH-R2, are involved in the stress-induced suppression of LH pulses. The aims of the present study were to examine the role of CRH-R1 and CRH-R2 in CGRP-induced suppression of LH pulses, and to investigate the effects of CGRP on CRH expression in the paraventricular nucleus (PVN) and central nucleus of the amygdala (CeA), which have prominent CRH neurone populations that receive dense CGRP innervations. The suppression of LH pulses by CGRP (1.5 μg icv) was completely prevented by intravenous administration of the CRH-R1 antagonist SSR125543Q (7.5 mg/rat iv, 30 min before CGRP), but was not affected by the CRH-R2 antagonist, astressin2-B (100 μg icv, 10 min before CGRP). CGRP increased the CRH mRNA expression in PVN and CeA. These results provide evidence of a role for CRH-R1 in mediating the suppressive effects of CGRP on pulsatile LH secretion in the female rat, and additionally raise the possibility of an involvement of PVN and CeA CRH neuronal populations in this suppression.
Introduction
It has previously been shown that central administration of calcitonin gene-related peptide (CGRP) evokes a classic neuroendocrine stress response by activation of the hypothalamo-pituitary-adrenocortical (HPA) axis, measured by an increase in circulating corticosterone levels (Dhillo et al. Citation2003; Li et al. Citation2004), and a suppression of the gonadotrophin-releasing hormone (GnRH) pulse generator controlling the reproductive neuroendocrine axis (Li et al. Citation2004; Bowe et al. Citation2005). It is well established that corticotrophin-releasing hormone (CRH), the principal factor driving the HPA axis during stress, is a potent inhibitor of the GnRH pulse generator in the monkey and rat (Rivier and Vale Citation1984; Williams et al. Citation1990; Cates et al. Citation2004), although not in the sheep (Clarke et al. Citation1990; Naylor et al. Citation1990). Furthermore, the central action of CGRP on the HPA and reproductive neuroendocrine axes appear to be mediated, at least in part, by CRH since the corticosterone response is abolished by CRH antisera (Kovacs et al. Citation1995) and the non-selective CRH antagonist, α-helical CRF9–41, markedly attenuates the suppression of pulsatile LH secretion (Li et al. Citation2004).
The physiological effects of the CRH family are mediated by two receptor subtypes, CRH-R1 and CRH-R2, both of which have a heterogeneous distribution pattern in the brain and a distinct physiopharmacology, suggesting distinct functional roles for each receptor (Chalmers et al. Citation1995; Primus et al. Citation1997). Of the two receptor subtypes, there is more evidence for a role of CRH-R1 both in behavioural and physiological responses to stress and the pivotal role of CRH in the HPA response to stress is evidently mediated by CRH-R1 (Vale et al. Citation1981). However, both CRH-R1 and CRH-R2 appear to potentially play a role in the stress-induced suppression of the GnRH pulse generator (Li et al. Citation2005, Citation2006).
The CRH neuronal populations mediating the effects of central CGRP on the GnRH pulse generator are currently unclear. Central administration of CGRP results in neuronal activation, measured by c-Fos expression, within both the medial preoptic area (mPOA) and the paraventricular nucleus (PVN), although the neurochemical phenotype of the activated neurones remains unknown (Li et al. Citation2004). It is currently unclear whether the CRH neuronal population in the PVN is involved in stress-induced suppression of LH pulses (Rivest and Rivier Citation1991; Hahn et al. Citation2003; Rivalland et al. Citation2006). Stress has been demonstrated to activate a number of other brain regions containing populations of CRH neurones, including the central nucleus of the amygdala (CeA) (Dayas et al. Citation2001; Curtis et al. Citation2002), which receives a dense CGRP innervation (Harrigan et al. Citation1994; Dobolyi et al. Citation2005).
It is currently unclear which CRH receptor subtype, or CRH neuronal populations are involved in mediating the CGRP-induced suppression of pulsatile LH release. The aims of the present study were to determine the CRH receptor subtypes mediating the effects of central CGRP on both the HPA axis and the GnRH pulse generator, and to examine the changes in CRH gene expression in the PVN and CeA; nuclei which receive CGRP innervation and are involved in stress responses.
Materials and methods
Animals and surgical procedures
Adult female Wistar rats (230–280 g) obtained from B&K Suppliers, Ltd (Hull, UK), were housed under controlled conditions (12 h light/12 h darkness; lights on at 07:00 h; temperature at 22 ± 2°C) and provided with food and water ad libitum. All animal procedures were undertaken in accordance with the United Kingdom Home Office Regulations. All surgical procedures were carried out under anaesthesia induced with ketamine (100 mg/kg ip; Pharmacia and Upjohn Ltd, Crawley, UK) and Rompun (10 mg/kg ip; Bayer, Leverkusen, Germany). Rats were bilaterally ovariectomized and fitted with a guide cannula (22 gauge; Plastics One, Virginia, USA) directed towards the left lateral cerebral ventricle intracerebroventricular (icv): the coordinates for implantation were 1.5 mm lateral, 0.6 mm posterior to bregma and 3.5 mm below the surface of the dura (Paxinos and Watson Citation1986). The guide cannula was fitted with a dummy cannula (Plastics One) to maintain patency (Cates et al. Citation2004). Following a 10-day recovery period, the rats were fitted with two indwelling cardiac catheters via the external jugular veins (Li et al. Citation2003). The catheters were exteriorised at the back of the head and secured to a cranial attachment: the rats were fitted with a 30-cm long metal spring tether (Instec Laboratories, Inc., Boulder, CO, USA). The distal end of the tether was attached to a fluid swivel (Instec Laboratories), which allowed the rat freedom to move around the enclosure. Experimentation commenced 3 days later.
Compounds
SSR125543Q{4-(2-Chloro-4-methoxy-5-methylphenyl)-N-[(1S)-2-cyclopropyl-1-(3-fluoro-4-methylphenyl)ethyl]5-methyl-N-(2-propynyl)-1,3-thiazol-2-amine, tosylate} (SSR), a selective CRH-R1 antagonist (Gully et al. Citation2002), was a generous gift from Sanofi-Synthelabo Recherche (Toulouse, France). SSR was dissolved in physiological saline containing 5% dimethyl sulfoxide (Sigma-Aldrich) and 5% Cremophor EL (Sigma-Aldrich) and administered intravenously as it is known to readily cross the blood–brain barrier (Gully et al. Citation2002). CGRP (Phoenix Europe GmBH) and astressin2-B (Sigma-Aldrich), a specific CRH-R2 antagonist (Rivier et al. Citation2002), were dissolved in artificial cerebrospinal fluid (aCSF) (Borsody and Weiss Citation2004) before administration. Astressin2-B was administered by icv injection as it is unlikely to cross the blood–brain barrier (Rivier et al. Citation2002). The dose and route of administration of the selective CRF antagonists were based on our previous experience (Li et al. Citation2005, Citation2006).
Experimental procedures
On the morning of experimentation, an internal cannula (Plastics One) with extension tubing preloaded with CGRP (1.5 μg in 4 μl aCSF; n = 8), a combination of CGRP and the specific CRH-R2 antagonist, astressin2-B (1.5 μg CGRP plus 100 μg astressin2-B in 4 μl aCSF; n = 8), astressin2-B alone (100 μg astressin2-B in 4 μl aCSF; n = 6) or aCSF (n = 7) was inserted into the guide cannula, extending 1.0 mm beyond its tip to reach the lateral cerebral ventricle. The distal end of the tubing was extended outside of the animal cage to allow remote infusion without disturbing the rat during the experiment (Cates et al. Citation2004). The rats were attached via one of the two cardiac catheters to a computer-controlled automated blood sampling system, which allows for the intermittent withdrawal of small blood samples (25 μl) without disturbing the animals (Cates et al. Citation2004). Blood sampling commenced at approximately 10:00 h when samples were collected every 5 min for 5 h for LH measurement. After removal of each 25-μl blood sample, an equal volume of heparinized saline (5 U/ml normal saline; CP Pharmaceuticals Ltd, Wrexham, UK) was automatically infused into the animal to maintain patency of the catheter and blood volume. After 110 min of controlled blood sampling, the selective CRH-R2 antagonist, astressin2-B, was injected icv in the relevant animals, followed by icv injection of CGRP 10 min later. For investigating the role of type-1 CRH receptors the specific CRH-R1 antagonist, SSR (7.5 mg in 5% DMSO, 5% Cremophor EL in saline; n = 8), or vehicle (5% DMSO, 5% Cremophor EL in saline; n = 7) was injected intravenously via the second cardiac catheter after 90 min of controlled blood sampling, followed by icv injection of CGRP (1.5 μg in 4 μl aCSF) or aCSF, 30 min later. Blood sampling continued throughout the experiment. Each rat received one treatment only. Blood samples were frozen at − 20°C for later assay to determine plasma LH concentrations.
Additionally, five blood samples were taken manually from all animals for the measurement of plasma corticosterone. Blood samples (50 μl) were withdrawn into heparinized syringes via the second cardiac catheter at − 60, 0, +30, +60 and +120 min, relative to CGRP administration. Plasma was then separated from these blood samples and frozen at − 20°C for later assay to determine plasma corticosterone concentrations.
In situ hybridization
A separate group of ovariectomized rats with icv cannula were administered either 1.5 μg CGRP (Phoenix Pharmaceuticals) in 4 μl aCSF (n = 8), or 4 μl aCSF alone (n = 7). Three hours later, the conscious rats were decapitated using a guillotine (Promed-Tec, Inc., Bellingham, MA, USA). Brains were then removed immediately, snap-frozen on dry ice and stored at − 80°C until processed. Frozen 15 μm coronal sections of the areas containing the PVN or CeA were cut on a cryostat using identifiable anatomical landmarks according to a rat brain atlas (Paxinos and Watson Citation1986) and thaw-mounted on to poly-l-lysine coated slides. Sections were stored at − 80°C until required. Before hybridization, sections were thawed and allowed to dry at room temperature for 10 min, and then fixed in 4% paraformaldehyde (PFA) for 5 min. Following three phosphate-buffered saline (PBS) washes, sections were acetylated for 10 min in 0.1 M triethylamine in 0.9% saline containing 0.025% acetic anhydride. Sections were then dehydrated, and delipidated in chloroform for 5 min. Sections were rehydrated in a series of ethanol baths and left to dry.
In situ hybridization for CRH mRNA was performed on sections using a 48-mer cDNA oligoprobe corresponding to amino acids 22–37 of preprocorticotrophin-releasing hormone where the specificity of the probe was determined previously (Thompson et al. Citation1987). Probes were labelled at the 3′-end with 35S-dATP using terminal deoxyribonucleotidyl transferase (Boehringer Mannheim, Sussex, UK) following the manufacturer's instructions. The labelled probe was recovered using a QIAquick nucleotide removal kit (Qiagen Ltd, Crawley, UK). Typical specific activity was 3–4 × 106 cpm/μl. The hybridization procedures were carried out as described previously (Harbuz and Lightman Citation1989). In brief, sections were hybridized with a labelled probe (1 × 106 cpm/section) using a hybridization buffer containing 50% deionized formamide, 0.5 mg/ml sheared salmon sperm DNA, 0.25 mg/ml yeast tRNA, 10 mM DTT and 100 mg/ml dextran overnight at 37°C. Sections were washed in 1 × SSC (0.15 M NaCl, 0.015 M sodium citrate, pH 7.0) four times for 15 min at 55°C, and then twice for 30 min at room temperature. They were then dipped twice in distilled water, and left to dry. All control and experimental sections were hybridized at the same time.
Image analysis
Hybridized sections and 14C-labelled standards of known radioactivity (Amersham International plc, Bucks, UK) were placed in X-ray cassettes and then apposed to autoradiographic film (Kodak Biomax MS-1, Sigma-Aldrich Co Ltd) for 7–10 days. The relative optical density of the autoradiographic images of CRH mRNA was measured by computer-assisted densitometry (Scion Image, NIH). For each rat, an average integrated optical density was obtained, consisting of at least four anatomically matched brain slices (every fourth section). The average value for each rat was used to calculate the mean for each experimental group. Relative optical density readings were performed with the experimenter blind to the treatment grouping of the subjects. The measurements of CRH mRNA hybridization signals were taken from sections of the PVN and CeA. For cellular localization of CRH mRNA hybrids, sections were then dipped in K-5 photographic emulsion (Ilford Imaging Ltd, Knutsford, UK) and exposed for 21 days. Emulsions were developed and sections counterstained with toluidine blue to histologically verify anatomical location. Anatomical identification of brain regions was based on a rat brain atlas (Paxinos and Watson Citation1986).
Radioimmunoassays
A double-antibody RIA supplied by the National Institute of Diabetes and Digestive and Kidney Diseases (NIDDK, Bethesda, MD, USA) was used to determine LH concentration in the 25 μl whole blood sample. The standard for the LH RIA is NIDDK-rLH-RP-2. The sensitivity of the assay was 0.093 ng/ml. The intraassay variation was 6.0% and the interassay variation was 5.4%. Total corticosterone was determined in plasma (10 μl) by RIA using a rat corticosterone kit (DPC, Los Angeles, CA, USA). The sensitivity of the assay was 7.5 ng/ml. The intra- and the interassay variations were 10.4 and 14.2%, respectively.
Statistical analysis
LH pulses were detected by use of the algorithm ULTRA (van Cauter Citation1988). Two intra-assay coefficients of variation of the assay were used as the reference threshold for pulse detection. The effect of treatment on pulsatile LH secretion was calculated by comparing the mean LH pulse interval before and after drug administration and expressed as “prolongation of LH pulse interval” as a percentage of the pretreatment control value. Statistical significance was tested on raw data using ANOVA followed by a Dunnett's test. Concentrations of corticosterone after drug administration were compared with mean pre-CGRP values. Statistical significance was tested using ANOVA and Dunnett's test. For comparison of in situ hybridization data, four to five sections encompassing each individual brain region were assessed in each animal, and the mean optical density compared between CGRP- and aCSF-treated animals. Statistical significance was tested using a one-way ANOVA followed by a Dunnett's test. For all experiments, p < 0.05 was considered statistically significant.
Results
Intracerebroventricular administration of CGRP resulted in a suppression of pulsatile LH release compared with aCSF-treated control rats (, B and E). Intravenous administration of the CRH-R1-specific antagonist, SSR (7.5 mg; 30 min prior to CGRP administration) completely prevented the inhibitory effects of CGRP (1.5 μg, icv) on pulsatile LH release (p < 0.05; and E). The rats pretreated with the CRH-R2-specific antagonist, astressin2-B (100 μg, icv; 10 min prior to CGRP administration) showed a prolongation of LH pulse interval in response to CGRP injection comparable to that in those rats administered CGRP alone (p < 0.05; and E). Antagonist vehicles had no effect on pulsatile LH release (data not shown).
Figure 1 Representative examples demonstrating the effects of administration ( ↓ ) of (A) 4 μl l intracerebroventricular (icv) aCSF, (B) 1.5 μg icv CGRP, (C) 7.5 mg intravenous SSR125543Q (SSR), followed 30 min later by 1.5 μg icv CGRP and (D) 100 μg astressin2-B (Ast2-B) icv, followed 10 min later by 1.5 μg CGRP icv on pulsatile LH secretion in ovariectomized rats. * denotes LH pulse. (E) Summary of the effect of administration of CGRP with the CRH-R1-specific antagonist, SSR, or the CRH-R2-specific antagonist, Ast2-B, on CGRP-induced suppression of pulsatile LH secretion in ovariectomized rats. Results are shown as percentage differences between pre-infusion and post-infusion LH pulse interval (mean ± SEM). Treatment with SSR completely blocked the CGRP-induced prolongation of LH pulses and was not significantly different from the aCSF control. Ast2-B did not have any significant effect. * p < 0.05 versus aCSF control. n = 6–8 rats per group.
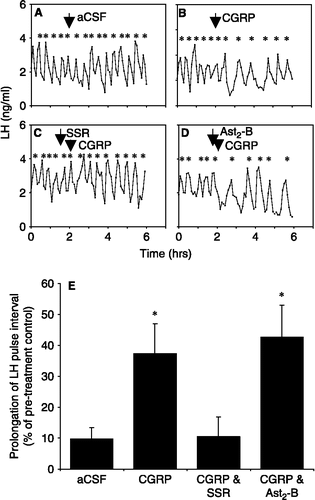
There was no significant difference in mean basal plasma corticosterone concentrations between any of the treatment groups (). The plasma concentrations of corticosterone increased rapidly reaching peak levels between 30 and 60 min after central CGRP administration. Pretreatment with SSR significantly reduced the mean peak levels for corticosterone in CGRP-treated rats (p < 0.05; ). Pretreatment with the CRH-R2 antagonist, astressin2-B, did not affect the CGRP-induced increase in plasma corticosterone concentrations (). Additionally, administration of 1.5 μg CGRP icv resulted in a significant increase in CRH mRNA expression, measured by relative optical density, within both PVN and CeA (p < 0.05; see and ) compared with aCSF controls.
Figure 2 The effect of CRH-R1 antagonist, SSR125543Q (SSR) and CRH-R2 antagonist, astressin2-B (Ast2-B) on central CGRP-induced corticosterone secretion in ovariectomized rats. Time zero represents CGRP administration. SSR (7.5 mg in 0.5 ml saline with 5% DMSO and 5% Cremophor EL vehicle) was administered intravenously 30 min before CGRP, whilst Ast2-B (100 μg in 4 μl aCSF) was administered by intracerebroventricular injection 10 min before CGRP injection. Values for plasma corticosterone concentrations (ng/ml) represent the mean ± SEM *p < 0.05 versus CGRP administration. n = 5–8 rats per group.
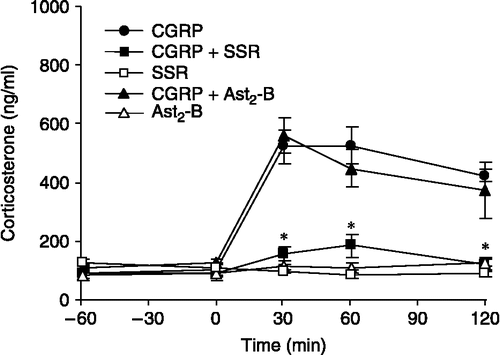
Figure 3 Representative dark field images of CRH mRNA expression in the hypothalamic paraventricular nucleus (PVN) in ovariectomized rats in response to (A) aCSF (4 μl icv) or (B) CGRP (1.5 μg icv). (C) Diagram showing the location of the PVN in a rostral coronal section of the rat brain indicated by the shaded area. (D) Summary of the effect in ovariectomized rats of central CGRP on CRH mRNA expression in the PVN when compared with aCSF-treated (control) rats. CRH mRNA levels are expressed as integrated density, and were calculated by comparing the optical density due to silver grain over the PVN to that of the background, using the analysis package Scion Image. *p < 0.05 versus aCSF-injected control rats. n = 7–8 rats per group.
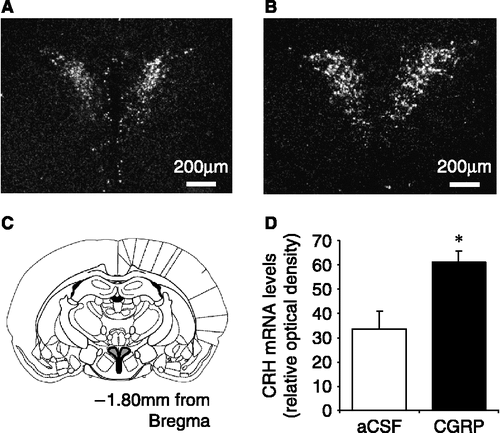
Figure 4 Representative dark field images of CRH mRNA expression in the central nucleus of the amygdala (CeA) in ovariectomized rats in response to (A) aCSF (4 μl icv) or (B) CGRP (1.5 μg icv). (C) Diagram showing the location of the CeA in a rostral coronal section of the rat brain indicated by the shaded area; one side is shown in A, B. (D) Summary of the effect in ovariectomized rats of central CGRP on CRH mRNA expression in the CeA when compared with aCSF-treated (control) rats. CRH mRNA levels are expressed as integrated density, and were calculated by comparing the optical density due to silver grain over the CeA to that of the background, using the analysis package Scion Image. * p < 0.05 versus aCSF-injected control rats. n = 7–8 rats per group.
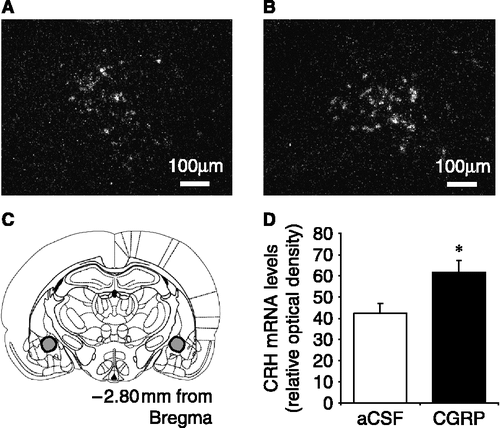
Discussion
Further to our previous studies demonstrating a role for CRH in mediating the inhibitory effects of central CGRP on the GnRH pulse generator (Li et al. Citation2004), the present study has shown that this effect is mediated primarily through CRH-R1 activation since administration of the CRH-R1-specific antagonist SSR completely blocked the inhibitory effect of CGRP on pulsatile LH secretion. In contrast, the CRH-R2-specific antagonist, astressin2-B, has no effect on the CGRP-induced suppression of pulsatile LH release. The efficacy of centrally administered astressin2-B at the dose used has previously been demonstrated to block stress-induced suppression of pulsatile LH secretion (Li et al. Citation2005, Citation2006). The SSR blockade of the inhibitory effects of CGRP on pulsatile LH release was effective for the entire 4 h post-CGRP sampling period, in contrast to the non-selective antagonist, αh-CRH9–41, used in our previous study (Li et al. Citation2004), which was only effective in blocking the CGRP-induced suppression of LH pulses for approximately 2 h. This observation might possibly be related to differing pharmacodynamics between the two antagonists. In vitro studies have demonstrated that the inhibitory effects of SSR are sustained for up to 24 h in the brain and pituitary (Gully et al. Citation2002), whilst in vivo, peripherally administered SSR blocks the effects of centrally administered CRH on ACTH secretion when given up to 6 h before the CRH injection (Gully et al. Citation2002).
Recent studies have demonstrated that both CRH-R1 and CRH-R2 play a role in mediating stress-induced suppression of the GnRH pulse generator although the precise involvement of either CRH receptor depends on the type of the stressor used (Li et al. Citation2005, Citation2006). Whilst the suppression of LH pulses induced by endotoxins or insulin-induced hypoglycaemia are mediated through CRH-R2 (Li et al. Citation2006), restraint stress-induced suppression of pulsatile LH release is mediated by both CRH-R1 and CRH-R2 (Li et al. Citation2005, Citation2006). The present study shows that the CGRP-induced suppression of the GnRH pulse generator appears to be mediated exclusively through CRH-R1. This observation raises the possibility that the role of endogenous CGRP in stress-induced suppression of LH pulses might involve CRH-R1-mediated pathways. We have previously shown that CGRP plays a pivotal role in hypoglycaemic stress-induced suppression of pulsatile LH secretion (Li et al. Citation2004). However, hypoglycaemic stress-induced suppression of the GnRH pulse generator appears to be mediated primarily by CRH-R2 (Li et al. Citation2004). This paradox is difficult to explain, but could be related to differences between the effects of exogenously administered CGRP and that endogenously released by stressful stimuli. Centrally administered CGRP is likely to activate CGRP receptors throughout the brain. By contrast, insulin-induced hypoglycaemia may only activate certain specific CGRP neurones, and not necessarily those involved in the CRH-R1-mediated suppression of pulsatile LH release. Furthermore, the inability of astressin2-B to block the CGRP-induced suppression of pulsatile LH release does not necessarily mean that CGRP has no effect on LH release via CRH-R2, merely that the inhibitory effects through CRH-R1 predominate.
In addition to its pivotal role in mediating the CGRP-induced suppression of LH pulse frequency, CRH-R1 also mediates the CGRP-induced increase in plasma corticosterone, a marker for HPA axis activation. This result is consistent with the well-established view that CRH-R1 is the primary receptor subtype at the level of the anterior pituitary involved in regulating ACTH/corticosterone secretion (Vale et al. Citation1981; Li et al. Citation2006). Further, in the present study, it was shown that after icv administration of CGRP there is an increase in CRH mRNA expression in the PVN. CGRP has previously been shown to cause an increase in Fos immunoreactivity in the PVN (Li et al. Citation2004). Although the phenotype of these activated neurones is unknown (Li et al. Citation2004), the present study suggests that at least some of the neurones might be CRH containing. Furthermore, administration of CGRP, either icv or directly into the PVN, has been shown to result in increased plasma corticosterone level (Kovacs et al. Citation1995; Dhillo et al. Citation2003) whilst treatment of hypothalamic explants with CGRP increases CRH release (Dhillo et al. Citation2003). Thus, it seems likely that CRH neurones activated in the PVN in response to central CGRP are involved in driving the HPA response. However, it is unlikely that the attenuation of the corticosterone response by the CRH-R1 antagonist, SSR, provides an explanation for the ability of this antagonist to prevent the CGRP-induced suppression of LH pulses since we have previously shown that exogenous administration of corticosterone does not affect pulsatile LH secretion in the rat (Li et al. Citation2003). Furthermore, since the CRH-R2 antagonist, astressin2-B, effectively attenuates the suppression of pulsatile LH secretion in response to various stressors without altering the corticosterone response (Li et al. Citation2006), this further confirms an absence of acute glucocorticoid negative feedback on pulsatile LH secretion in the rat.
The extent of involvement of the CRH neuronal population in the PVN in the stress-induced suppression of the reproductive neuroendocrine axis is currently unclear. Administration of noradrenaline directly into the PVN results in a suppression of LH pulses, an effect which can be blocked by icv administration of a CRH antagonist (Tsukamura et al. Citation1994). Furthermore, administration of a catecholamine synthesis inhibitor directly into the PVN blocks stress-induced suppression of pulsatile LH release (Maeda et al. Citation1994). However, electrolytic lesioning of the PVN does not block the suppression of LH pulses caused by interleukin-1β or footshock stress (Rivest and Rivier Citation1991), and neuronal tract tracing studies have found no direct CRH projections from the PVN to the GnRH-rich regions of the mPOA in the rat (Hahn et al. Citation2003) and very few in the sheep (Rivalland et al. Citation2006). Thus, it is currently unknown whether the CRH neurones located in the PVN are involved in the stress-induced suppression of the GnRH pulse generator and subsequent LH release. The lack of direct projections from the PVN to the mPOA (Hahn et al. Citation2003; Rivalland et al. Citation2006) suggests that CRH originating in the PVN does not directly suppress the GnRH neurones of the mPOA. However, the possibility of an indirect effect of PVN CRH neurones on the GnRH neuronal cells of the mPOA remains to be investigated.
CGRP administration also significantly increased CRH mRNA expression within the CeA, a brain region that could potentially be involved in the stress-induced suppression of LH release. The CeA contains a large population of CRH neurones (Swanson et al. Citation1983) and is activated in response to stress (Dayas et al. Citation2001; Curtis et al. Citation2002). Electrical stimulation of the CeA results in the activation of the autonomic nervous system, including increases in heart rate, blood pressure and plasma catecholamines (Bonvallet et al. Citation1972; Schlor et al. Citation1984); all of which are also observed in response to central administration of CRH (Brown and Fisher Citation1985; Fisher Citation1989). Furthermore, lesions of the CeA attenuate these autonomic responses and corticosterone release evoked by either restraint or interleukin-1β administration (Zhang et al. Citation1986; van de Kar et al. Citation1991; Xu et al. Citation1999). Both restraint and chronic social stress also result in an up-regulation of CRH mRNA expression within the CeA (Kalin et al. Citation1994; Albeck et al. Citation1997). There is also strong evidence for an involvement of CGRP in regulating the activity of CRH neurones in the CeA. Abundant CGRP projections from the parabrachial nucleus form dense synaptic contacts with CRH neurones in the CeA (Harrigan et al. Citation1994; Dobolyi et al. Citation2005). Expression of CGRP receptors (Oliver et al. Citation1998) and a high density of CGRP-binding sites have also been identified within the CeA (van Rossum et al. Citation1997). Additionally, direct microinjection of CGRP into the CeA results in autonomic responses (Nguyen et al. Citation1986; Brown and Gray Citation1988) similar to those observed following electrical stimulation of the CeA (Bonvallet et al. Citation1972; Schlor et al. Citation1984). In the present study, the increased CRH mRNA within the CeA in response to central CGRP suggests that central CGRP activates the CRH neurones of the CeA. A variety of stressors, including both footshock and restraint, have been shown to result in an increase in CRH mRNA expression within the CeA (Hsu et al. Citation1998; Makino et al. Citation1999). Furthermore, immobilization stress in the rat results in an increase in Fos expression within the CGRP perikarya of the parabrachial nucleus (Kainu et al. Citation1993). These studies suggest that the CGRP neurones of the parabrachial nucleus might be involved in the physiological stress response, potentially through an interaction with the CeA CRH neurone population. However, although the CGRP induced activation of CRH neurones within the CeA appears to be involved in the autonomic response to stress, whether their activation by CGRP is involved in the regulation of the GnRH pulse generator remains to be established. The CRH neurones of the CeA do not appear to project directly to the GnRH-rich mPOA (Hahn et al. Citation2003), and though the amygdala has been shown to have an inhibitory influence on LH secretion (Kawakami and Kimura Citation1975), the role of the amygdala in the stress-induced suppression of pulsatile LH release is poorly understood. Whilst CGRP appears to activate CRH neurones in both the PVN and CeA, further work is required to determine whether these CRH neuronal populations are responsible for mediating the inhibitory effects of CGRP on the GnRH pulse generator. Nevertheless, the present study provides evidence of a role for CRH-R1 in CGRP-induced suppression of hypothalamic GnRH pulse generator activity in the rat.
Acknowledgements
The authors wish to thank Dr Parlow of the National Institute of Diabetes, Digestive and Kidney Diseases for providing the LH RIA kit, and Dr D. Riochet of Sanofi-Synthelabo Recherche for the generous gift of SSR125543Q. This work was supported by The Wellcome Trust and the Guy's and St Thomas' Charitable Foundation (UK). J.E.B. is a recipient of a Guy's and St Thomas' Charitable Foundation PhD Studentship.
References
- Albeck DS, McKittrickm CR, Blanchard DC, Blanchard RJ, Nikulina J, McEwen BS, Sakai RR. Chronic social stress alters levels of corticotropin-releasing factor and arginine vasopressin mRNA in rat brain. J Neurosci 1997; 17: 4895–4903
- Bonvallet M, Bobo EG. Changes in phrenic activity and heart rate elicited by localized stimulation of amygdala and adjacent structures. Electroencephalogr Clin Neurophysiol 1972; 32: 1–16
- Borsody MK, Weiss JM. The effects of endogenous interleukin-1 bioactivity on locus coeruleus neurons in response to bacterial and viral substances. Brain Res 2004; 1007: 39–56
- Bowe JE, Li XF, Kinsey-Jones JS, Paterson S, Brain SD, Lightman SL, O'Byrne KT. Calcitonin gene-related peptide-induced suppression of luteinizing hormone pulses in the rat: The role of endogenous opioid peptides. J Physiol 2005; 566: 921–928
- Brown MR, Fisher LA. Corticotropin-releasing factor: Effects on the autonomic nervous system and visceral systems. Fed Proc 1985; 44: 243–248
- Brown MR, Gray TS. Peptide injections into the amygdala of conscious rats: Effects on blood pressure, heart rate and plasma catecholamines. Regul Pept 1988; 21: 95–106
- Cates PS, Li XF, O'Byrne KT. The influence of 17beta-oestradiol on corticotrophin-releasing hormone induced suppression of luteinising hormone pulses and the role of CRH in hypoglycaemic stress-induced suppression of pulsatile LH secretion in the female rat. Stress 2004; 7: 113–118
- Chalmers DT, Lovenberg TW, De Souza EB. Localization of novel corticotropin-releasing factor receptor (CRF2) mRNA expression to specific subcortical nuclei in rat brain: Comparison with CRF1 receptor mRNA expression. J Neurosci 1995; 15: 6340–6350
- Clarke IJ, Horton RJ, Doughton BW. Investigation of the mechanism by which insulin-induced hypoglycemia decreases luteinizing hormone secretion in ovariectomized ewes. Endocrinology 1990; 127: 1470–1476
- Curtis AL, Bello NT, Connolly KR, Valentino RJ. Corticotropin-releasing factor neurones of the central nucleus of the amygdala mediate locus coeruleus activation by cardiovascular stress. J Neuroendocrinol 2002; 14: 667–682
- Dayas CV, Buller KM, Crane JW, Xu Y, Day TA. Stressor categorization: Acute physical and psychological stressors elicit distinctive recruitment patterns in the amygdala and in medullary noradrenergic cell groups. Eur J Neurosci 2001; 14: 1143–1152
- Dhillo WS, Small CJ, Jethwa PH, Russell SH, Gardiner JV, Bewick GA, Seth A, Murphy KG, Ghatei MA, Bloom SR. Paraventricular nucleus administration of calcitonin gene-related peptide inhibits food intake and stimulates the hypothalamo-pituitary-adrenal axis. Endocrinology 2003; 144: 1420–1425
- Dobolyi A, Irwin S, Makara G, Usdin TB, Palkovits M. Calcitonin gene-related peptide-containing pathways in the rat forebrain. J Comp Neurol 2005; 489: 92–119
- Fisher LA. Central autonomic modulation of cardiac baroreflex by corticotropin-releasing factor. Am J Physiol 1989; 256: H949–H955
- Gully D, Geslin M, Serva L, Fontaine E, Roger P, Lair C, Darre V, Marcy C, Rouby PE, Simiand J, Guitard J, Gout G, Steinberg R, Rodier D, Griebel G, Soubrie P, Pascal M, Pruss R, Scatton B, Maffrand JP, Le FG. 4-(2-Chloro-4-methoxy-5-methylphenyl)-N-[(1S)-2-cyclopropyl-1-(3-fluoro-4-methylphenyl) ethyl]5-methyl-N-(2-propynyl)-1,3-thiazol-2-amine hydrochloride (SSR125543A): A potent and selective corticotrophin-releasing factor(1) receptor antagonist. I. Biochemical and pharmacological characterization. J Pharmacol Exp Ther 2002; 301: 322–332
- Hahn JD, Kalamatianos T, Coen CW. Studies on the neuroanatomical basis for stress-induced oestrogen-potentiated suppression of reproductive function: Evidence against direct corticotropin-releasing hormone projections to the vicinity of luteinizing hormone-releasing hormone cell bodies in female rats. J Neuroendocrinol 2003; 15: 732–742
- Harbuz MS, Lightman SL. Responses of hypothalamic and pituitary mRNA to physical and psychological stress in the rat. J Endocrinol 1989; 122: 705–711
- Harrigan EA, Magnuson DJ, Thunstedt GM, Gray TS. Corticotropin releasing factor neurons are innervated by calcitonin gene-related peptide terminals in the rat central amygdaloid nucleus. Brain Res Bull 1994; 33: 529–534
- Hsu DT, Chen FL, Takahashi LK, Kalin NH. Rapid stress-induced elevations in corticotropin-releasing hormone mRNA in rat central amygdala nucleus and hypothalamic paraventricular nucleus: An in situ hybridization analysis. Brain Res 1998; 788: 305–310
- Kainu T, Honkaniemi J, Gustafsson JA, Rechardt L, Pelto-Huikko M. Co-localization of peptide-like immunoreactivities with glucocorticoid receptor- and Fos-like immunoreactivities in the rat parabrachial nucleus. Brain Res 1993; 615: 245–251
- Kalin NH, Takahashi LK, Chen FL. Restraint stress increases corticotropin-releasing hormone mRNA content in the amygdala and paraventricular nucleus. Brain Res 1994; 656: 182–186
- Kawakami M, Kimura F. Inhibition of ovulation in the rat by electrical stimulation of the lateral amygdale. Endocrinol Jpn 1975; 22: 61–65
- Kovacs A, Biro E, Szeleczky I, Telegdy G. Role of endogenous CRF in the mediation of neuroendocrine and behavioral responses to calcitonin gene-related peptide in rats. Neuroendocrinology 1995; 62: 418–424
- Li XF, Mitchell JC, Wood S, Coen CW, Lightman SL, O'Byrne KT. The effect of oestradiol and progesterone on hypoglycaemic stress-induced suppression of pulsatile luteinizing hormone release and on corticotropin-releasing hormone mRNA expression in the rat. J Neuroendocrinol 2003; 15: 468–476
- Li XF, Bowe JE, Mitchell JC, Brain SD, Lightman SL, O'Byrne KT. Stress-induced suppression of the gonadotropin-releasing hormone pulse generator in the female rat: A novel neural action for calcitonin gene-related peptide. Endocrinology 2004; 145: 1556–1563
- Li XF, Bowe JE, Lightman SL, O'Byrne KT. Role of corticotropin-releasing factor receptor-2 in stress-induced suppression of pulsatile luteinizing hormone secretion in the rat. Endocrinology 2005; 146: 318–322
- Li XF, Bowe JE, Kinsey-Jones JS, Brain SD, Lightman SL, O'Byrne KT. Differential role of corticotrophin-releasing factor receptor types 1 and 2 in stress-induced suppression of pulsatile luteinising hormone secretion in the female rat. J Neuroendocrinol 2006; 18: 602–610
- Naylor AM, Porter DW, Lincoln DW. Central administration of corticotrophin-releasing factor in the sheep: Effects on secretion of gonadotrophins, prolactin and cortisol. J Endocrinol 1990; 124: 117–125
- Maeda K, Cagampang FR, Coen CW, Tsukamura H. Involvement of the catecholaminergic input to the paraventricular nucleus and of corticotropin-releasing hormone in the fasting-induced suppression of luteinizing hormone release in female rats. Endocrinology 1994; 134: 1718–1722
- Makino S, Shibasaki T, Yamauchi N, Nishioka T, Mimoto T, Wakabayashi I, Gold PW, Hashimoto K. Psychological stress increased corticotropin-releasing hormone mRNA and content in the central nucleus of the amygdala but not in the hypothalamic paraventricular nucleus in the rat. Brain Res 1999; 850: 136–143
- Nguyen KQ, Sills MA, Jacobowitz DM. Cardiovascular effects produced by microinjection of calcitonin gene-related peptide into the rat central amygdaloid nucleus. Peptides 1986; 7: 337–339
- Oliver KR, Wainwright A, Heavens RP, Hill RG, Sirinathsinghji DJ. Distribution of novel CGRP1 receptor and adrenomedullin receptor mRNAs in the rat central nervous system. Brain Res Mol Brain Res 1998; 57: 149–154
- Paxinos G, Watson C. The rat brain in stereotaxic coordinates4th ed. Academic Press, New York 1986
- Primus RJ, Yevich E, Baltazar C, Gallager DW. Autoradiographic localization of CRF1 and CRF2 binding sites in adult rat brain. Neuropsychopharmacology 1997; 17: 308–316
- Rivalland ET, Tilbrook AJ, Turner AI, Iqbal J, Pompolo S, Clarke IJ. Projections to the preoptic area from the paraventricular nucleus, arcuate nucleus and the bed nucleus of the stria terminalis are unlikely to be involved in stress-induced suppression of GnRH secretion in sheep. Neuroendocrinology 2006; 16: 1–13
- Rivest S, Rivier C. Influence of the paraventricular nucleus of the hypothalamus in the alteration of neuroendocrine functions induced by intermittent footshock or interleukin. Endocrinology 1991; 129: 2049–2057
- Rivier C, Vale W. Influence of corticotropin-releasing factor on reproductive functions in the rat. Endocrinology 1984; 114: 914–921
- Rivier J, Gulyas J, Kirby D, Low W, Perrin MH, Kunitake K, DiGruccio M, Vaughan J, Reubi JC, Waser B, Koerber SC, Martinez V, Wang L, Taché Y, Vale W. Potent and long-acting corticotropin releasing factor (CRF) receptor 2 selective peptide competitive antagonists. J Med Chem 2002; 45: 4737–4747
- Schlor KH, Stumpf H, Stock G. Baroreceptor reflex during arousal induced by electrical stimulation of the amygdala or by natural stimuli. J Auton Nerv Syst 1984; 10: 157–165
- Swanson LW, Sawchenko PE, Rivier J, Vale WW. Organization of ovine corticotropin-releasing factor immunoreactive cells and fibers in the rat brain: An immunohistochemical study. Neuroendocrinology 1983; 36: 165–186
- Thompson RC, Seasholtz AF, Herbert E. Rat corticotropin-releasing hormone gene: Sequence and tissue-specific expression. Mol Endocrinol 1987; 1: 363–370
- Tsukamura H, Nagatani S, Cagampang FR, Kawakami S, Maeda K. Corticotropin-releasing hormone mediates suppression of pulsatile luteinizing hormone secretion induced by activation of alpha-adrenergic receptors in the paraventricular nucleus in female rats. Endocrinology 1994; 134: 1460–1466
- Vale W, Spiess J, Rivier C, Rivier J. Characterization of a 41-residue ovine hypothalamic peptide that stimulates secretion of corticotropin and beta-endorphin. Science 1981; 213: 1394–1397
- van Cauter E. Estimating false-positive and false-negative errors in analyses of hormonal pulsatility. Am J Physiol 1988; 254: E786–E794
- van de Kar LD, Piechowski RA, Rittenhouse PA, Gray TS. Amygdaloid lesions: Differential effect on conditioned stress and immobilization-induced increases in corticosterone and renin secretion. Neuroendocrinology 1991; 54: 89–95
- van Rossum D, Hanisch UK, Quirion R. Neuroanatomical localization, pharmacological characterization and functions of CGRP, related peptides and their receptors. Neurosci Biobehav Rev 1997; 21: 649–678
- Williams CL, Nishihara M, Thalabard JC, Grosser PM, Hotchkiss J, Knobil E. Corticotropin-releasing factor and gonadotropin-releasing hormone pulse generator activity in the rhesus monkey, electrophysiological studies. Neuroendocrinology 1990; 52: 133–137
- Xu Y, Day TA, Buller KM. The central amygdala modulates hypothalamic-pituitary-adrenal axis responses to systemic interleukin-1beta administration. Neuroscience 1999; 94: 175–183
- Zhang JX, Harper RM, Ni HF. Cryogenic blockade of the central nucleus of the amygdala attenuates aversively conditioned blood pressure and respiratory responses. Brain Res 1986; 386: 136–145