Abstract
Many studies have shown that early life stress may lead to impaired brain development, and may be a risk factor for developing psychiatric pathologies such as depression. However, few studies have investigated the impact that early life stress might have on the onset and development of neurodegenerative disorders, such as Parkinson's disease, which is characterized in part by the degeneration of dopaminergic neurons in the nigrostriatal pathway. The present study subjected rat pups to a maternal separation paradigm that has been shown to model adverse early life events, and investigated the effects that it has on motor deficits induced by a unilateral, intrastriatal injection of 6-hydroxydopamine (12 μg/4 μl). The female rats were assessed for behavioral changes at 28 days post-lesion with a battery of tests that are sensitive to the degree of dopamine loss. The results showed that rats that had been subjected to maternal separation display significantly impaired performance in the vibrissae and single-limb akinesia test when compared to normally reared animals. In addition, there was a significant increase in the loss of tyrosine hydroxylase staining in maternally separated rats. Our results therefore suggest that adverse experiences sustained during early life contribute to making dopamine neurons more susceptible to subsequent insults occurring during more mature stages of life and may therefore play a role in the etiopathogenesis of Parkinson's disease.
Abbreviations: | ||
DA | = | dopamine |
HPA | = | hypothalamic–pituitary–adrenal axis |
MS | = | maternal separation |
MPTP | = | 1-methyl-4-phenyl-1,2,3,6-tetrahydropyridine |
PD | = | Parkinson's disease |
PND | = | post-natal day |
TH-ir | = | tyrosine hydroxylase immunoreactivity |
Introduction
The etiology of neurodegenerative disorders, including Parkinson's disease (PD), continues to be investigated intensively, with the aim of developing improved treatments. Although the precise mechanism by which PD is induced remains unknown, both genetic susceptibility and exposure to environmental toxins are regarded as major risk factors for developing the disease (Yamashita and Matsumoto Citation2007). A number of candidate genes, including PINK 1, LRRK2, DJ-1, and α-synuclein, have been identified, each with mutations that can play a prominent role in the pathogenesis of PD in some individuals. However, such genes seem to be responsible for only a small proportion of PD cases, whereas the vast majority of patients have a sporadic onset (Bonifati Citation2005; Douglas et al. Citation2007).
A possible environmental cause for idiopathic PD was suggested by the observation of PD-like symptoms following exposure to the neurotoxin 1-methyl-4-phenyl-1,2,3,6-tetrahydropyridine (MPTP) (Ballard et al. Citation1985; Burns et al. Citation1985). This discovery was followed by intensive research on the effects of a range of toxic pesticides and herbicides, which strengthened the hypothesis that PD is often initiated by environmental toxins (Gorrell et al. Citation1996; Ben Moyal-Segal and Soreq Citation2006). Among possible toxins responsible for PD are those that trigger an inflammatory response in the CNS. Toxic elements or infectious agents may mediate their deleterious effects by activating microglia and/or astroglia, and this has been proposed as an important mechanism underlying neuronal cell death in PD (Cadepond et al. Citation2002).
Activated microglia and/or astroglia respond to endocrine, neural, and immune factors and are fundamental components of the neuroendocrine–immune axis (Yung-Testas et al. Citation1992; Marchetti et al. Citation2005). Although astro- and/or microgliosis are likely to be of benefit at sites of injury, they can have detrimental consequences on neuronal survival, and their chronic overstimulation can induce neuronal damage (Banati et al. Citation1993). Although activated astrocytes and microglia serve as endogenous sources of various cytokines, that, upon release, orchestrate the cellular responses aimed at re-establishing tissue integrity and repair (Streit Citation2002), their chronic overstimulation may release pro-inflammatory cytokines, reactive oxygen intermediates, proteinases, and complement proteins, thereby provoking severe neuronal damage (Aloisi Citation2001; Le et al. Citation2001). In addition, defective mitochondrial function continues to receive widespread and sustained research attention as a possible cause of PD (Schapira Citation1994, Citation2006; Sherer et al. Citation2002).
These studies usually implicate adverse exposure during adulthood, whereas only limited reports propose a neurodevelopmental basis for neurodegeneration. These include the possible effects on the nigrostriatal dopaminergic system that certain teratogens may have, including exposure to agrichemicals (Barlow et al. Citation2004) and an iron-enriched diet (Jones et al. Citation2002) during the perinatal period, or maneb (Cory-Slechta et al. Citation2005), cocaine (Glatt et al. Citation2004) or bacterial endotoxin lipopolysaccharide (LPS) exposure (Ling et al. Citation2006) during the prenatal period.
The hypothesis that early life events can increase the vulnerability of neurons to later insults is further supported by studies of other types of neurons. If the fetus is exposed to higher than normal levels of glucocorticoid, there can be detrimental effects on the long-term survival rate of cerebellar granule cells, leaving these cells more susceptible to the effects of oxidative stress (Ahlbom et al. Citation2000). In addition, there seems to be an important interaction between exposure to pathogenic inflammatory stimuli, such as gram-negative bacterial endotoxin, and key regulatory neuroendocrine systems, such as the hypothalamic–pituitary–adrenal (HPA) system. Other disturbances during early neonatal life may result in marked effects on HPA axis activity, with increased corticosterone pulse frequency and pulse amplitude, as well as long-lasting immune system dysregulation, including increased sensitivity of lymphocytes to stress-induced suppression of proliferation and protection against adjuvant-induced arthritis (Shanks et al. Citation2000). Similarly disruption of maternal care during early development has been shown to alter the mesolimbic dopamine system rendering subjects more prone to addictive behavior (Meaney et al. Citation2002) and depression-like symptoms (Matthews and Robbins Citation2003).
In the current study, we hypothesized that exposure to stressful stimuli during early life predisposes an individual to develop neurodegenerative disease, specifically PD. Clinical conditions associated with exposure to severe stress (e.g. post-traumatic stress disorder and depression) have been found to demonstrate signs of neuronal degeneration, including evidence of atrophy in the hippocampus (Sapolsky et al. Citation1996; Bremner et al. Citation1997). Similarly, the subjection of rodents and non-human primates to chronic levels of restraint stress (Watanabe et al. Citation1992), chronic multiple stressors (Magarinos and McEwen Citation1995), or psychosocial stress (Uno et al. Citation1989; McKittrick et al. Citation2000; Czeh et al. Citation2001) resulted in degenerative changes seen within hippocampal CA3 pyramidal neurons. In addition, exposure to stressors may also exacerbate the damaging effects on the brain caused by chronic exposure to drugs of abuse (Matuszewich and Yamamoto Citation2004), which can be associated with decreased DA function, as evidenced by reductions in D2 DA receptors and DA release in the striatum (Volkow et al. Citation2007).
We (Daniels et al. Citation2004), and others (Lubach et al. Citation1995; Ladd et al. Citation1996; Lyons et al. Citation1999; Sánchez et al. Citation2001) have previously shown that rat pups periodically deprived of maternal care show behavioral and neurochemical alterations reminiscent of stress-related mental disorders. These observations indicate that maternal deprivation experienced during early life can compromise the normal development and function of the CNS during the adult years, particularly during old age when the CNS is most vulnerable to adverse effects. One effect may be a marked metabolic disturbance of the monoamines DA, serotonin and noradrenaline (Thierry et al. Citation1968).
The unilateral administration of the neurotoxin 6-hydroxydopamine (6-OHDA) into the brain in rodents is frequently used to study various aspects of PD, such as the neurological deficits (Ungerstedt Citation1971; Schwarting and Huston Citation1996), the benefits of brain grafts (Dunnett et al. Citation1983; Curran et al. Citation1993) and the potential therapeutic effects of agents, including neurotrophic factors (Winkler et al. Citation1996) (Zigmond and Keefe Citation1997). To test the hypothesis that early life stress may predispose to PD, rats were subjected to maternal separation (MS) for 3 h a day on post-natal days (PND) 2–14, and injected unilaterally with 6-OHDA into the striatum at PND 35, a time at which the synthesis rates of striatal D1 and D2 DA receptors reach their peaks (Andersen Citation2002; Pienaar et al. Citation2007). We observed that MS significantly increased both the behavioral and neurotoxic effects of 6-OHDA, suggesting a possible predisposing effect of early adversity in the etiology of PD.
Materials and methods
Animals
Sprague–Dawley rats were used to generate female pups for this study. Rats were obtained from the Central Animal Research Facility of the University of Cape Town. The animals were housed in 40 × 25 × 20 cm acrylic cages (four rats per cage) with pine wood shavings that covered the cage floor. Rat chow and tap water were available ad libitum. Environmental temperature was maintained at 22°C and lights were on each day from 06:00 to 18:00 h. The experimental procedures were approved by the Committee for Experimental Animal Research of the University of Cape Town (project number: 006/008) and were also in accordance with the US National Institutes of Health Guide for Care and Use of Laboratory Animals.
Maternal separation paradigm
MS has been described as a potent naturalistic stressor (Stanton et al. Citation1988) and has been used extensively as a model of early life stress by our research group (Daniels et al. Citation2004; Faure et al. Citation2006), and by many others (Lubach et al. Citation1995; Ladd et al. Citation1996; van Oers et al. Citation1998; Lyons et al. Citation1999; Sánchez et al. Citation2001). Adult male–female pairs were housed under normal conditions for mating. Immediately after the rat pups were born, the adult males were removed, while the mothers remained with the pups. Litters were sexed and culled to 8 pups per dam. The various dams with their pups were randomly assigned to either a control (non-separated) or MS group. MS involved physically removing the dam from the home cage, and transferring the pups while still in their cages to another room to prevent communication with the mother via ultrasound vocalizations (Hofer Citation1994). MS commenced on PND 2 and terminated on PND 14, lasting for 3 h per day, from 09:00 to 12:00 h. It is important to note that at no time were the pups handled during this period. The temperature of the room to which the rat pups were transferred was maintained between 31 and 33°C, thereby minimizing the possible influence of hypothermia. After PND 14, the pups returned to normal housing conditions. Control pups were handled identically to experimental ones, except that the pups were not separated from the dam during the first 14 days after they were born. On PND 21 both the MS and the non-MS rats were weaned by permanently removing them from the dam.
Surgical procedures
Surgery was performed on PND 35. To prepare the surgical site, the scalp hair was clipped and the site disinfected with 70% ethanol. Body heat was conserved during surgery by placing the rat on a heating pad (Rex C10, Center for Electronic Services, University of Stellenbosch). Rats were anaesthetized using a combination of ketamine hydrochloride (Anaket-V®, Bayer Healthcare, South Africa) and medetomidine hydrochloride (Domitor®, Pfizer, South Africa) (0.1 ml/100 g, i.p.) and placed in a stereotaxic frame (David Kopf Instruments, Tujunga, USA). Bregma and lambda were used to level the horizontal plane of the skull. From bregma, the following coordinates were used with the leveled skull: anterior, 0.30 mm; lateral, 2.7 mm; ventral, 5.6 mm below the dura mater. A small burr hole was drilled at the determined coordinates and a 22-gauge surgical-steel cannula was slowly inserted into the brain. A 6-OHDA solution was freshly prepared, kept on ice, and protected from exposure to light. The solution consisted of 12 μg (free-base) 6-OHDA (Sigma, St. Louis, MO, USA), dissolved in 4 μl of 0.1% l-ascorbic acid in 0.9% sterile saline, and was administered unilaterally into the left striatum, using a 341-A model syringe pump (Sage Instruments, USA), running at an injection rate of 0.5 μl/min. The cannula was left in place for an additional 5 min following the infusion to facilitate the optimal diffusion of the solution. Subsequently, the needle was slowly retracted from the brain. The hole drilled in the skull was filled with sterilized oxidized cellulose (Ethicon, UK), the incision sutured, and lastly coated with an iodine solution (Purdue Frederick, USA). The rats were placed in individual cages under heating-lamps until full recovery was achieved, after which they were returned to their home cages.
Behavioral assessment
The vibrissae-evoked forelimb placing test and the bracing test were done to ascertain the degree of asymmetry following the 6-OHDA injection. These tests were performed between 09:00 and 13:00 h, both 14 and 28 days after the intra-striatal injection by an evaluator blind to treatment allocation. Since the two sets of data were essentially identical, only the 28 day data are reported.
Vibrissae-evoked forelimb placing test
Rats were subjected to the vibrissae-evoked forelimb placing test as previously described by Schallert (Citation2000). This test evaluates the degree of sensorimotor integration that occurs across the two hemispheres. Intact rats instinctively attempt to place their forelimb(s) upon any nearby surface when their whiskers are stimulated (Woodlee et al. Citation2005). Consistent with Parkinsonian akinesia, unilaterally 6-OHDA-lesioned rats fail to produce a placement response from the limbs regardless of the sensory trigger, while the contralateral vibrissae maintain the ability to reliably trigger limb placement. In executing the test, the rat was gently held by the torso, allowing its forelimbs to hang down freely. Independent testing of each forelimb was induced by carefully brushing its vibrissae against the edge of a tabletop. Each vibrissae brush constituted a trial, with 10 trials from each side being performed. If the rat placed its forelimb swiftly upon the table's surface immediately following vibrissae stimulation, a score of 1 was allocated, while a failure to place the paw on the table provided a score of zero.
Bracing (adjusting steps) test
This test evaluates the animal's capacity to adjust its stepping to regain postural stability, when weight-shifts are imposed by the experimenter (Schallert et al. Citation1979, Citation1992; Lindner et al. Citation1995; Olsson et al. Citation1995). The rat was held by its torso with its hindquarters and one forelimb lifted above the surface of a table to allow the weight of its body to be supported by one forelimb alone. The rat was then slowly moved in the forward direction. The number of adjusting steps was recorded as the rat was moved in 30 s trial periods. The test was performed for each forelimb.
Tyrosine hydroxylase immunohistochemistry
The effect of the injections on tyrosine hydroxylase immunoreactivity (TH-ir) was evaluated in three rats belonging to each group. The rats were anaesthetized with Halothane™ (Safeline Pharmaceuticals, Roodepoort, South Africa), after which 60 ml of phosphate buffered saline (PBS) was used to exsanguinate the animals via transcardial perfusion. This was followed by 180 ml of 4% paraformaldehyde–PBS (Merck, South Africa) to fix the brain tissue. The brains were rapidly removed from the skull and placed in 30% sucrose solution for approximately 48 h. The brain was then blocked and frozen over liquid nitrogen vapor until sectioning. To identify TH, free-floating coronal sections 60 μm thick were cut with a cryostat at ( − 20°C. The sections were quenched by incubating with 0.3% hydrogen peroxide for 30 min at room temperature in a humidifying container. This was followed by a succession of washes (5 × 10 min) with PBS (0.15 M, pH 7.4). The sections were placed in a 10 mM citrate buffer (pH 6.0) and boiled in a microwave oven for 15 min. The sections were left to cool, before the wash-cycle was repeated. The sections were then pretreated with 150 μl horse blocking serum and 0.1% Triton X-100 for 30 min before application of the primary monoclonal anti-TH antibody (dilution 1:500, DiaSorin, USA) and overnight incubation at 4°C. The next day the sections were washed in PBS (5 × 10 min), before applying the secondary biotinylated antibody (dilution 1:400) for 1 h at room temperature. The wash routine was repeated before incubating the slices with peroxidase-labeled Mouse IgG ABC reagent (ABC kit, DakoCytomation, USA) with concentrated diaminobenzidine (DAB) as the chromogen. Tissue sections were incubated at room temperature until staining developed. The sections were given a final 15 min rinse with distilled water, before dehydrating them with 96–100% ethanol, clearing with Xylol (Merck, South Africa) and mounting the sections onto clear glass slides.
Quantification of relative TH immunoreactivity
We adopted the method described by Anstrom et al. (Citation2007) to assess the extent of the damage caused by a unilateral 6-OHDA injection. An assessor, blind to the treatment of the rats, performed the evaluation. The sections that were analyzed represented the anterior, middle, and posterior striatum from both control and maternally separated rats, which comprised a total of 24 slides per group. Digital photographic images of all the sections were captured under constant, standardized lighting conditions and exposure using the 10 × objective on a Zeiss microscope (Carl Zeiss, Germany) fitted with Brightfield optics (Nicon, USA) and a Diagnostic Instruments Spot Insight QE camera (Kodak, USA) was used. For each slide, six independent digitalized photographic images were produced 3 per hemisphere (). This was done in a consistent manner anchored by objective landmarks (e.g. corpus callosum (cc) dorsally, optic chiasm ventrally, and the third ventricle that served as the medial anchor-point). The degree of immunocytochemical staining asymmetry of each image was determined with Zeiss Axiovision image analysis software. The density of the TH-ir surface-area of the image of the infused hemisphere was divided by the corresponding measurement of the image of the non-infused hemisphere. The final values were expressed as a ratio of TH-ir on the lesioned side compared to TH-ir on the non-lesioned side and are given as a percentage. Values closer to 100 indicate minimal loss of TH staining, while values closer to zero indicate severe staining asymmetry. The percentage values of the three images were averaged to give an indication of damage per slide, and averaging the percentages of all the slides for a given rat provided the degree of staining asymmetry for a specific rat. The final value was calculated by averaging the percentage scores of the three individual rats per group.
Figure 1 A representative micro-photograph showing decreased tyrosine hydroxylase staining following unilateral 6-OHDA injection in the lateral striatum of a rat that has been subjected to maternal separation. The broken lines depict the three sample areas per slide that were used to quantify TH immunocytochemical stain density using Zeiss K3100 (v.4) analyzing software. LV, left ventricle; CC, corpus callosum; and AC, anterior commissure.
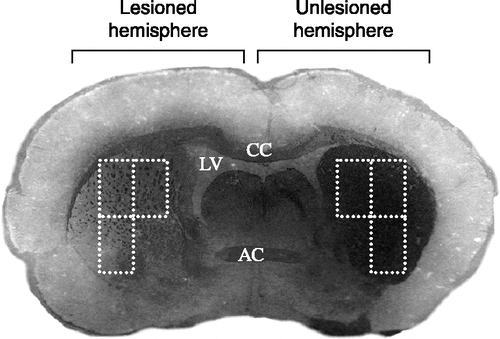
Statistical analysis
The statistical analysis of all the data was performed using Graphpad Prism V.4 (USA). For analyzing the behavioral data, comparisons between groups were performed using the Kruskal–Wallis test, followed by Dunn's Multiple Comparison's test, while the Mann–Whitney test was applied when only two groups were compared. To analyze the results of the TH-immunocytochmistry, a Student's t-test was used. Data are expressed as the group means ± SEM and results are considered to differ significantly if p < 0.05.
Results
Vibrissae-evoked forelimb placing test
Two behavioral tests were employed at 14 and 28 days after lesioning to evaluate the effect of 6-OHDA on the locomotor abilities of rats. This was achieved by comparing limb movements occurring on the side ispsilateral to the lesion with those made by the contralateral limb. Since the results at 14 and 28 days were essentially identical, only the 28 day data will be reported here.
For the vibrissae-elicited forelimb placing test, a failed response was defined as the inability of the rat to place both its paws on the tabletop immediately following stimulation of the vibrissae, when brushing them lightly against the table top surface. No significant difference was detected between MS and non-MS rats in terms of performance by the limb ipsilateral to the lesioned hemisphere. 6-OHDA produced a 65% increase in the number of errors made by the paw contralateral to the lesion compared to the ipsilateral one (p < 0.01). Moreover, the number of failed responses by the contralateral paw was 100% greater in the MS group than in the non-MS group (p < 0.05) ().
Figure 2 Failed responses in non-MS and MS rats during the vibrissae-evoked forelimb placing test performed at 28 days after receiving a unilateral 6-OHDA injection into the left striatum. *p < 0.01 indicates a significant difference from the number of failed responses made by the limb ipsilateral to the lesioned hemisphere, when assessing motor asymmetry in the same rat. **p < 0.05 indicates a significant difference between the normal-reared and the MS group in terms of the number of failed responses made by the limb contralateral to the toxin-infused hemisphere (Kruskal–Wallis test, followed by Dunn's Multiple Comparison test). Data represent the mean ± SEM (n = 15 animals/group).
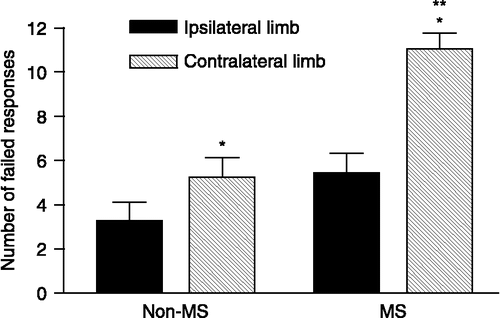
Bracing test
The bracing test showed that in non-MS rats, the number of steps made within a 30 s trial conducted along a straight line by the limb contralateral to the lesioned brain hemisphere was significantly decreased due to 6-OHDA, when compared to the performance by the limb that was ipsilateral to the lesioned hemisphere (p < 0.01). The MS rats revealed a significantly greater deficit in use of the contralateral limb compared to the non-MS rats (62 versus 39%, respectively, p < 0.05; ). No significant difference was detected between the two groups in terms of performance by the limb that was ipsilateral to the lesioned hemisphere (p>0.05, 18.9 ± 0.57 for the non-MS controls versus 19.2 ± 0.6 for the MS rats).
Figure 3 The number of steps taken by the limb contralateral to the lesioned striatum of both groups of rats (*p < 0.01) compared to the performance by the ipsilateral left limb in the bracing test, performed 28 days after a unilateral 6-OHDA injection into the left striatum. Fewer steps were taken by the limb contralateral to the 6-OHDA-induced lesion, and this reduction was exaggerated in the MS group compared to the non-MS group (**p < 0.05; Kruskal–Wallis, followed by Dunn's Multiple Comparison's test). Data represent the mean ± SEM (n = 15 animals/group).
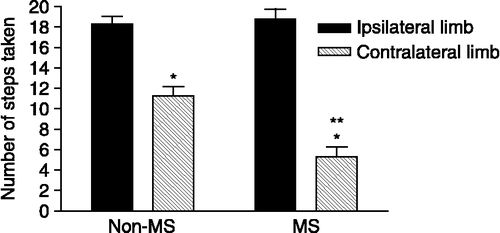
Tyrosine hydroxylase immunocytochemistry
The extent of the intrastriatal lesions was qualitatively assessed by investigating the intensity of TH-ir fibers in coronal sections of the forebrain under a light microscope. There was no significant difference in the staining intensity of TH fibers in the non-lesioned hemisphere of non-MS rats when compared to that of MS animals (43.42% of surface area analyzed for non-MS rats versus 50.71% for MS animals). In non-MS rats 6-OHDA injections produced a consistent and distinct lesion, as reflected by a substantial decrease in immuno-staining in the lesioned striatum compared to the non-lesioned side (p < 0.01, 43.42% for non-lesioned side versus 20.74% for lesioned side). This decrease induced by the 6-OHDA injection resulted in a significantly greater loss of TH-ir in the striatum of MS rats (p = 0.001, 50.71% non-lesioned side versus 8.9% lesioned side).
Discussion
Our results showed that female rats subjected to MS demonstrated exaggerated locomotor deficits compared to normally reared controls after 6-OHDA lesions. Furthermore, compared with non-MS animals, rats subjected to MS displayed greater 6-OHDA-induced loss of DA terminals in the striatum as assessed by the loss of TH-ir. These findings may be related to clinical reports that stressful experiences can worsen the degree of motor-related impairment in PD patients, such as increased tremor when patients express anger or anxiety (Schwab and Zieper Citation1965), and that experiencing severe bouts of stress may even exacerbate the underlying neuropathology (Smith et al. Citation2002).
In studies of 6-OHDA-treated rats, compensatory adaptations in the remaining DA neurons are observed, with rats often completely recovering from the gross motor deficits ordinarily expected from a larger dose or even not manifesting any gross motor symptoms at all (Abercrombie et al. Citation1990; Robinson et al. Citation1994; Fornaquera and Schwarting 1999). However, studies have also shown that the presentation of stressors to these seemingly recovered animals often results in sustained neurological impairments (Stricker and Zigmond Citation1974; Snyder et al. Citation1985).
Our finding that MS rats display greater impairments than non-MS animals after 6-OHDA lesioning could reflect a direct effect of early life stress on neuronal function. Zhang et al. (Citation2002) found, for example, that maternal deprivation enhances cell death in the cerebral cortex and hippocampus. Thus, it is feasible that MS may have increased the vulnerability of DA neurons to the oxidative stress that is associated with 6-OHDA administration, although further work is necessary to confirm the role of such putative mechanisms. Rearing conditions have been shown to induce long-lasting alterations in the central monoaminergic systems of the brain (Daniels et al. Citation2004), particularly DA levels (Miura et al. Citation2002; Arborelius and Ecklund Citation2007), DA receptor density (Ploj et al. Citation2003), and decreased DA turnover (Matthews et al. Citation2001). Although these changes have been reported for brain areas other than the SN or the striatum, it raises the possibility that similar alterations could also have occurred within the nigrostriatal system.
An alternative mechanism may involve biochemical pathways affected by glucocorticoids, since it is well accepted that stress can trigger increased glucocorticoid release into the peripheral circulation. We (Daniels et al. Citation2004) and others (Ishikawa et al. Citation1992; Plotsky and Meaney Citation1993; Ladd et al. Citation2000; Slotten et al. Citation2006) have previously shown that early life stress results in a dysregulated HPA axis, reflected by aberrant baseline corticosterone levels and an abnormal corticosterone response to subsequent stress. Although this biochemical alteration may initially serve to improve focus, processing, and response to the stressful event, an excessive amplitude and duration of such changes may lead to neuronal cell death (Sapolsky Citation2002).
In accordance with this possibility, administration of corticosterone to adrenalectomized rats has been shown to increase neuronal lesions induced by the injection of quinolinic acid into the striatum (Ngai and Herbert Citation2005), and to decrease glucocorticoid receptor density (Morale et al. Citation2004). Moreover, the key role played by glucocorticoids in stress-induced neuropathology has been shown, particularly for the hippocampus, to reduce the ability of some cells to survive toxic threats. Mechanisms by which this may occur include reduced energy metabolism that is initiated by an inhibition of glucose uptake processes (Horner et al. Citation1990). Alternatively, this may involve increased extracellular Ca2 + signaling (Han et al. Citation2005), decreased expression of neurotrophic factors, for example brain-derived neurotrophic factor (Cosi et al. Citation1993; Schaaf et al. Citation1998; Lippmann et al. Citation2007), or by stimulating apoptosis through the reduced expression of anti-apoptotic proteins and the increased expression levels of pro-apoptotic proteins (Almeida et al. Citation2000; Sapolsky Citation2000; Cárdenas et al. Citation2002). Since it has been shown that the cell-mediated immune status of animals are affected by exposure to early social deprivation, with evidence of a decreased ratio of helper to suppressor T cells (Lewis et al. Citation2000), compromised immune function may also have played a role in the augmented neurological impairments seen in the MS rats.
In summary, exposure to early life stress exacerbated the toxic effects of 6-OHDA on locomotor activity and decreased the degree of striatal TH expression determined on the lesioned hemispheric side. We do not yet know whether the effects seen are a direct consequence that the separation stress may have had on the central dopaminergic system, or whether it was induced indirectly via other centrally acting factors. Thus, considerable further investigation will be required to elucidate the mechanism of the effects that we have observed. We believe, however, that such investigations are well advised as they will provide important insights into the means by which early adversity can increase the risk of developing a neurodegenerative condition such as PD.
Acknowledgements
This research was supported in part by the USPHS (DA018087 and NS019608). We gratefully acknowledge the help of Professor Daan Nel (Center for Statistical Consultation, University of Stellenbosch, South Africa), for his help offered in statistically analyzing the data, Dr Carol Chase (University of Stellenbosch, South Africa) for assisting in setting up the software for quantifying the density of TH staining and Mrs. Shula Johnson and Dr Musa Mabandla, both from the University of Cape Town (South Africa), for breeding and maternal separation of the rats.
References
- Abercrombie E.D, Bonatz AE, Zigmond MJ. Effects of l-DOPA on extracellular dopamine in striatum of normal and 6-hydroxydopamine-treated rats. Brain Res 1990; 525: 36–44
- Ahlbom E, Goqvadze V, Chen M, Celsi G, Ceccatelli S. Prenatal exposure to high levels of glucocorticoids increases the susceptibility of cerebellar granule cells to oxidative stress-induced cell death. Proc Natl Acad Sci USA 2000; 97: 14726–14730
- Almeida OF, Conde GL, Crochemore C, Demeneix BA, Fischer D, Hassan AH, Meyer M, Holsboer F, Michaelidis TM. Subtle shifts in the ratio between pro- and anti-apoptotic molecules after activation of corticosteroid receptors decide neuronal fate. FASEB J 2000; 14: 779–790
- Aloisi F. Immune function of microglia. Glia 2001; 36: 165–179
- Andersen SL. Changes in the second messenger cyclic AMP during development may underlie motoric symptoms in attention deficit/hyperactive disorder (ADHD). Behav Brain Res 2002; 130: 197–201
- Anstrom KK, Schallert T, Woodlee MT, Shattuck A, Roberts DC. Repetitive vibrissae-elicited forelimb placing before and immediately after unilateral 6-hydroxydopamine improves outcome in a model of Parkinson's disease. Behav Brain Res 2007; 179: 183–191
- Arborelius L, Ecklund MB. Both long and brief maternal separation produces persistent changes in tissue levels of brain monoamines in middle-aged female rats. Neuroscience 2007; 145: 738–750
- Ballard PA, Tetrud JW, Langston JW. Permanent human parkinsonism due to 1-methyl-4-phenyl-1,2,3,6-tetrahydropyridine (MPTP): Seven cases. Neurology 1985; 35: 949–956
- Banati R, Gehrmann BJ, Schubert P, Kreutzberg GW. Cytotoxicity of microglia. Glia 1993; 7: 111–118
- Barlow BK, Richfield EK, Cory-Slechta DA, Thiruchelvam M. A fetal risk factor for Parkinson's disease. Dev Neurosci 2004; 26: 11–23
- Ben Moyal-Segal L, Soreq H. Gene–environment interactions in sporadic Parkinson's disease. J Neurochem 2006; 97: 1740–1755
- Bonifati V. Genetics of Parkinson's disease. Minerva Med 2005; 96: 175–186
- Bremner JD, Randall P, Vermetten E, Staib L, Bronen RA, Mazure C, Capelli S, McCarthy G, Innis RB, Charney DS. Magnetic resonance imaging-based measurement of hippocampal volume in posttraumatic stress disorder related to childhood physical and sexual abuse – a preliminary report. Biol Psychiatry 1997; 41: 23–32
- Burns RS, LeWitt PA, Ebert MH, Pakkenberg H, Kopin IJ. The clinical syndrome of striatal dopamine deficiency. Parkinsonism induced by 1-methyl-4-phenyl-1,2,3,6-tetrahydropyridine (MPTP). N Engl J Med 1985; 312: 1418–1421
- Cárdenas SP, Parra C, Bravo J, Morales P, Lara HE, Herrera-Marschitz M, Fiedler JL. Corticosterone differentially regulates bax, bcl-2 and bcl-x mRNA levels in the rat hippocampus. Neurosci Lett 2002; 331: 9–12
- Cory-Slechta DA, Thiruchelvam M, Richfield EK, Barlow BK, Brooks AI. Developmental pesticide exposures and the Parkinson's disease phenotype. Birth Defects Res A Clin Mol Teratol 2005; 73: 136–139
- Cosi C, Speorri PE, Comelli MC, Guidolin D, Skaper SD. Glucocorticoids depress activity-dependent expression of BDNF mRNA in hippocampal neurones. Neuroreport 1993; 4: 527–530
- Curran EJ, Albin RL, Becker JB. Adrenal medulla grafts in the hemiparksonian rat: Profile of behavioral recovery predicts restoration of the symmetry between the two striata in measures of pre- and postsynaptic dopamine function. J Neurosci 1993; 13: 3864–3877
- Czeh MT, Watanabe T, Frahm J, De Biurrun G, van Kampen M, Bartolomucci A, Fuchs E. Stress-induced changes in cerebral metabolites, hippocampal volume, and cell proliferation are prevented by antidepressant treatment with tianeptine. Proc Natl Acad Sci USA 2001; 98: 12796–12801
- Daniels WMU, Pietersen CY, Carstens ME, Stein DJ. Maternal separation in rats lead to anxiety-like behaviour and a blunted ACTH response and altered neurotransmitter levels in response to a subsequent stressor. Metab Brain Dis 2004; 19: 3–14
- Douglas MR, Lewthwaite AJ, Nicholl DJ. Genetics of Parkinson's disease and parkinsonism. Expert Rev Neurother 2007; 7: 657–666
- Dunnett SB, Bjorklund A, Schmidt RH, Stenevi U, Iversen SD. Intracerebral grafting of neuronal cell suspensions. IV. Behavioral recovery in rats with unilateral 6-OHDA lesions following implantation of nigral cell suspensions in different forebrain sites. Acta Physiol Scand Suppl 1983; 522: 29–37
- Faure J, Uys JDK, Marais L, Stein DJ, Daniels WMU. Early maternal separation followed by later stressors leads to dysregulation of the HPA-axis and increases in hippocampal NGF and NT-3 levels in a rat model. Metab Brain Dis 2006; 21: 181–188
- Fornaguera J, Schwarting RK. Early behavioral changes after nigro-striatal system damage can serve as predictors of striatal dopamine depletion. Prog Neuropsychopharmacol Bial Psychiatry. 1999; 23: 1353–1368, 8
- Glatt SJ, Trksak GH, Cohen OS, Simeone BP, Jackson D. Prenatal cocaine exposure decreases nigrostriatal dopamine release in vitro: Effects of age and sex. Synapse 2004; 53: 74–89
- Gorrell JM, DiMonte D, Graham D. The role of the environment in Parkinson's disease. Environ Health Perspect 1996; 104: 652–654
- Han JZ, Lin W, Chen YZ. Inhibition of ATP-induced calcium influx in HT4 cells by glucocorticoids: Involvement of protein kinase A. Acta Pharmacol Sin 2005; 26: 199–204
- Hofer MA. On the nature and consequences of early loss. Psychosom Med 1994; 58: 570–581
- Horner HC, Packan DR, Sapolsky RM. Glucocorticoids inhibit glucose transport in cultured hippocampal neurons and glia. Neuroendocrinol 1990; 52: 57–64
- Ishikawa M, Hara CH, Ohdo S, Ogawa N. Plasma corticosterone response of rats with sociopsychological stress in the communication box. Physiol Behav 1992; 52: 475–480
- Jones BC, Wheeler DS, Beard JL, Grigson PS. Iron deficiency in rats decreases acquisition of and suppresses responding for cocaine. Pharmacol Biochem Behav 2002; 73: 813–819
- Ladd CO, Owens MJ, Nemeroff CB. Persistent changes in corticotropin-releasing factor neuronal systems induced by maternal deprivation. Endocrinology 1996; 137: 1212–1218
- Ladd CO, Huot RL, Thrivikraman KV, Nemeroff CB, Meaney MJ, Plotsky PM. Long-term behavioral and neuroendocrine adaptations to adverse early experience. Prog Brain Res 2000; 122: 81–103
- Lewis MH, Gluck JP, Petitto JM, Hensley LL, Ozer H. Early social deprivation in nonhuman primates: Long-term effects on survival and cell-mediated immunity. Biol Psychiatry 2000; 47: 119–126
- Le W, Rowe D, Xie W, Ortiz I, He Y, Appel SH. Microglial activation and dopaminergic cell injury: An in vitro model relevant to Parkinson's disease. J Neurosci 2001; 21: 8447–8455
- Lindner MD, Winn SR, Baetge EE, Hammang JP, Gentile FT, Doherty E, McDermott PE, Frydel B, Ullman MD, Schallert T, et al. Implantation of encapsulated catecholamine and GDNF producing cells in rats with unilateral dopamine depletions and Parkinsonian symptoms. Exp Neurol 1995; 132: 62–76
- Ling Z, Zhu Y, Tong C, Snyder JA, Lipton JW, Carvey PM. Progressive dopamine neuron loss following supra-nigral lipopolysaccharide (LPS) infusion into rats exposed to LPS prenatally. Exp Neurol 2006; 199: 499–512
- Lippmann M, Bress A, Nemeroff CB, Plotsky PM, Monteggia LM. Long-term behavioural and molecular alterations associated with maternal separation in rats. Eur J Neurosci 2007; 25: 3091–3098
- Lubach GR, Coe CL, Ershler WB. Effects of early rearing environment on immune responses of infant rhesus monkeys. Brain Behav Immun 1995; 9: 31–46
- Lyons WE, Mamounas LA, Ricaurte GA, Coppola V, Reid SW, Bora SH, Wihler C, Koliatsos VE, Tessarollo L. Brain-derived neurotrophic factor-deficient mice develop aggressiveness and hyperphagia in conjunction with brain serotonergic abnormalities. Proc Natl Acad Sci USA 1999; 96: 15239–15244
- Magarinos AM, McEwen BS. Stress-induced atrophy of apical dendrites of hippocampal CA3c neurons: Involvement of glucocorticoid secretion and excitatory amino acid receptors. Neuroscience 1995; 69: 89–98
- Marchetti B, Kettenmann H, Streit WJ. Glia-neuron crosstalk in neuroinflammation, neurodegeneration, and neuroprotection. Brain Res Rev 2005; 48: 129–489
- Matthews K, Robbins TW. Early experience as a determinant of adult behavioural responses to reward: The effects of repeated maternal separation in the rat. Neurosci Biobehav Rev 2003; 27: 45–55
- Matthews JW, Dalley JW, Matthews C, Tsai TH, Robbins TW. Periodic maternal separation of neonatal rats produces region- and gender-specific effects on biogenic amine content in postmortem adult brain. Synapse 2001; 40: 1–10
- Matuszewich L, Yamamoto BK. Effects of chronic stress on methamphetamine-induced dopamine depletions in the striatum. Ann NY Acad Sci 2004; 1032: 312–314
- McKittrick CR, Magarinos AM, Blanchard DC, Blanchard RJ, McEwen BS, Sakai RR. Chronic social stress reduces dendritic arbors in CA3 of hippocampus and decreases binding to serotonin transporter sites. Synapse 2000; 36: 85–94
- Meaney MJ, Brake W, Gratton A. Environmental regulation of the development of mesolimbic dopamine systems: A neurobiological mechanism for vulnerability to drug abuse?. Psychoneuroendocrinology 2002; 27: 127–138
- Miura H, Qiao H, Ohta T. Attenuating effects of the isolated rearing condition on increased brain serotonin and dopamine turnover elicited by novelty stress. Brain Res 2002; 926: 10–17
- Morale MC, Serra PA, Delogu MR, Migheli R, Rocchita G, Tirolo C, Caniglia S, Testa N, L'Wpiscopo F, Gennuso F, Scoto GM, Barden N, Miele E, Desole MS, Marchetti B. Glucocorticoid receptor deficiency increases vulnerability of the nigrostriatal dopaminergic system: Critical role of glial nitric oxide. FASEB J 2004; 18: 164–166
- Ngai LY, Herbert J. Glucocorticoid enhances the neurotoxic actions of quinolinic acid in the striatum in a cell-specific manner. J Neuroendocrinol 2005; 17: 424–434
- van Oers HJ, de Kloet ER, Levine S. Early vs. late maternal deprivation differentially alters the endocrine and hypothalamic responses to stress. Brain Res Dev Brain Res 1998; 111: 245–252
- Olsson M, Nikkhah G, Bentlage C, Bjorklund A. Forelimb akinesia in the rat Parkinson model: Differential effects of dopamine agonists and nigral transplants as assessed by a new stepping test. J Neurosci 1995; 15: 3865–3875
- Pienaar IS, Schallert T, Russell VA, Kellaway LA, Carr JA, Daniels WMU. Early pubertal female rats are more resistant than males to 6-hydroxydopamine neurotoxicity and behavioural deficits: A possible role for trophic factors. Restor Neurol Neurosci 2007; 25: 1–14
- Ploj K, Roman E, Nylander I. Long-term effects of maternal separation on ethanol intake and brain opioid and dopamine receptors in male Wistar rats. Neuroscience 2003; 121: 787–799
- Plotsky PM, Meaney MJ. Early, postnatal experience alters hypothalamic corticotropin-releasing factor (CRF) mRNA, median eminence CRF content and stress-induced release in adult rats. Brain Res Mol Brain Res 1993; 18: 195–200
- Robinson TE, Mocsary Z, Camp DM, Whishaw IQ. Time course of recovery of extracellular dopamine following partial damage to the nigrostriatal dopamine system. J Neurosci 1994; 14: 2687–2696
- Sánchez MM, Ladd CO, Plotsky PM. Early adverse experience as a developmental risk factor for later psychopathology: Evidence from rodent and primate models. Dev Psychopathol 2001; 13: 419–449
- Sapolsky RM. The possibility of neurotoxicity in the hippocampus in major depression: A primer on neuron death. Biol Psychiatry 2000; 48: 755–765
- Sapolsky RM. Chickens, eggs and hippocampal atrophy. Nat Neurosci 2002; 5: 1111–1113
- Sapolsky RM, Trafton J, Tombaugh GC. Excitotoxic neuron death, acidotic endangerment and the paradox of acidotic protection. Adv Neurol 1996; 71: 237–244
- Schaaf MJ, de Jong J, de Kloet ER, Vreudenhil E. Downregulation of BDNF mRNA and protein in the rat hippocampus by corticosterone. Brain Res 1998; 813: 112–120
- Schallert T. CNS plasticity and assessment of forelimb sensorimotor outcome in unilateral rat models of stroke, cortical ablation, parkinsonism and spinal cord injury. Neuropharmacol 2000; 39: 777–787
- Schallert T, De Ryck M, Whishaw IQ, Teitelbaum P. Excessive bracing reactions and their control by atropine and l-dopa in an animal analog of parkinsonism. Exp Neurol 1979; 65: 33–34
- Schallert T, Norton D, Jones TA. A clinically relevant unilateral rat model of parkinsonian akinesia. J Neural Transplant Plast 1992; 3: 332–333
- Schapira AH. Evidence for mitochondrial dysfunction in Parkinson's disease – a critical appraisal. Movement Dis 1994; 9: 125–138
- Schapira AH. Mitochondrial disease. Lancet 2006; 368: 70–82
- Schwab RS, Zieper I. Effects of mood, motivation, stress, and alertness on the performance in Parkinson's disease. Psychiatr Neurol 1965; 150: 345–357
- Schwarting RK, Huston JP. The unilateral 6-hydroxydopamine lesion model in behavioral brain research. Analysis of functional deficits, recovery and treatments. Prog Neurobiol 1996; 50: 275–331
- Shanks N, Windle RJ, Perks PA, Harbuz MS, Jessop DS, Ingram CD, Lightman SL. Early-life exposure to endotoxin alters hypothalamic–pituitary–adrenal function and predisposition to inflammation. Proc Natl Acad Sci USA 2000; 97: 5645–5650
- Sherer TB, Betarbet R, Greenamyre JT. Environment, mitochondria, and Parkinson's disease. Neuroscientist 2002; 8: 192–197
- Slotten HA, Kalinichev M, Hagan JJ, Marsden CA, Fone KC. Long-lasting changes in behavioural and neuroendocrine indices in the rat following neonatal maternal separation: Gender-dependent effects. Brain Res 2006; 1097: 123–132
- Smith AD, Castro SL, Zigmond MJ. Stress-induced Parkinson's disease: A working hypothesis. Physiol Behav 2002; 77: 527–531
- Snyder AM, Snyder EM, Zigmond MJ. Stress-induced neurological impairments in an animal model of parkinsonism. Ann Neurol 1985; 18: 544–551
- Stanton ME, Gutierrez YR, Levine S. Maternal deprivation potentiates pituitary–adrenal stress responses in infant rats. Behav Neurosci 1988; 102: 692–700
- Streit WJ. Microglia as neuroprotective: Immunocompetent cells of the CNS. Glia 2002; 40: 133–139
- Stricker EM, Zigmond MJ. Effects on homeostasis of intraventricular injections of 6-hydroxydopamine in rats. J Comp Physiol Psychol 1974; 86: 973–994
- Thierry AM, Javoy F, Glowinski J, Kety SS. Effects of stress on the metabolism of norepinephrine, dopamine and serotoninin the central nervous system of the rat. I. Modifications of norepinephrine turnover. J Pharmacol Exp Ther 1968; 163: 163–171
- Ungerstedt U. Postsynaptic supersensitivity after 6-hydroxy-dopamine induced degeneration of the nigro-striatal dopamine system. Acta Physiol Scand Suppl 1971; 367: 69–93
- Uno H, Tarara R, Else JG, Suleman MA, Sapolsky RM. Hippocampal damage associated with prolonged and fatal stress in primates. J Neurosci 1989; 9: 1705–1711
- Volkow ND, Fowler JS, Wang GJ, Swanson JM, Telang F. Dopamine in drug abuse and addiction: Results of imaging studies and treatment implications. Arch Neurol 2007; 64: 1575–1579
- Watanabe Y, Gould E, Daniels DC, Cameron H, McEwen BS. Tianeptine attenuates stress-induced morphological changes in the hippocampus. Eur J Pharmacol 1992; 222: 157–162
- Winkler C, Sauer H, Lee CS, Bjorklund A. Short-term GDNF treatment provides long term rescue of lesioned nigral dopaminergic neurons in a rat model of Parkinson's disease. J Neurosci 1996; 16: 7206–7215
- Woodlee MT, Asseo-Garcíam AM, Zhao X, Liu S-J, Jones TA, Schallert T. Testing forelimb placing ‘across the midline’ reveals distinct, lesion-dependent patterns of recovery in rats. Exp Neurol 2005; 191: 310–317
- Yamashita H, Matsumoto M. Molecular pathogenesis, experimental models and new therapeutic strategies for Parkinson's disease. Regen Med 2007; 2: 447–455
- Yung-Testas I, Renoir JM, Brugnard GL, Greene EE. Demonstration of steroid hormones receptor and steroid action in primary cultures of rat glial cells. J Steroid Biochem Mol Biol 1992; 41: 3–8
- Zhang LX, Levine S, Dent G, Zhan Y, Xing G, Okimoto D, Kathleen Gordon M, Post RM, Smith MA. Maternal deprivation increases cell death in the infant rat brain. Brain Res Dev Brain Res 2002; 133: 1–11
- Zigmond MJ, Keefe KA. 6-Hydroxydopamine as a tool for studying catecholamines in adult animals: Lessons from the neostriatum. Highly selective neurotoxins: Basic and clinical applications, R Kostrzewa. Humana Press, Totowa 1997; 75–108