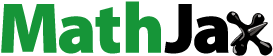
1. Introduction
In the case of large bone defects, allogenic or autologous grafts are not always possible for bone reconstruction due to many shortcomings. Cultivating bone-forming cells within a natural microporous material is a promising solution to enhance the healing process and restoration of bone. The natural coral exoskeleton which has an interconnected porous architecture similarly to spongy bone can be used as a scaffold and has shown great ability to promote the development of bone-forming cells and help the reconstruction of large bone defects in sheep.
In this study, the potential use of a new material as a scaffold in bone tissue engineering, deproteinized dentin, is investigated. Like bone, dentin is a mineralized tissue containing collagen fibers and nano-sized mineral platelets. It is known that the mechanical properties of the support material and the porous structure have an influence on the behavior of seeded cells. Therefore, the first step of our study is to characterize the proposed material to compare it to coral. Thermally deproteinized dentin was already characterized to demonstrate its ability to mimick hypomineralized enamel. We propose here to characterize chemically deproteinized dentin using mercury intrusion porosimetry and nanoindentation.
2. Methods
2.1 Sample Preparation
Extracted teeth were stored for a maximum of 3 months in a 1% chloramine-T solution at 4°C. Dentin discs were cut from crown segments parallel to the occlusal surface at the top of the pulp chamber using a water-cooled, low-speed diamond saw (Isomet, Buehler, Evanston, IL, USA). Enamel was then removed by grinding, any specimens in which pulp horn or enamel was detected were eliminated. The samples were then deproteinized by chemical treatment (protocol described by Chen et al). After the preparation procedure, nine slices were stored in distilled water.
2.2 Field Emission Scanning Electron Microscopy
FESEM was used to observe the pore structure of the deproteinized dentin using a FIB-SEM Helios 660 microscope operated at low voltage (1–5 kV). The two specimens were coated using coater Bio Rad SC 500 (Microscience division, Elescience).
2.3 Mercury Intrusion Porosimetry (MIP)
Mercury porosimetry enables the 3D quantification of the open porosity of various materials. It is based on the fact that mercury is a non-wetting liquid and, therefore, will not penetrate pores by capillary action unless it is forced to do so. During the test, the sample is put under vacuum and an incremental pressure is applied to the mercury so it enters the pores of the sample incrementally. At each pressure step, the volume of mercury entering the sample is measured. By assuming that the pores are cylindrical, the applied pressure P can be related to the pore diameter D via ɣ and θ, the surface tension and contact angle of mercury:
The contact angle and surface tension of mercury are set for all the tests at 130° and 485 dyn/cm. In this study, we used a mercury porosimeter (Micromeritics Autopore IV, Micromeritics) that can detect pores from 6nm to 420μm. To obtain reliable results, the manufacturer recommends a final mercury intrusion volume between 20 and 80% of the stem volume. To fulfill this condition, we used six samples for each test.
2.4 Nanoindentation
The specimen reserved for nanoindentation was embedded before micron polishing. The experiment was performed using a nanoindenter (XP, MTS). The specimens were mounted on a support to ensure the exposure of a flat surface at right angles to the indenter (a calibrated Berkovitch indenter). Two series of 28 acquisitions were realized on one slice with different depth limits (250 and 750 nm). The force–displacement curve of loading and unloading was used to calculate the mechanical properties (elastic modulus and hardness) of each indentation point.
3. Results and discussion
3.1 FESEM
The SEM observation (Figure ) allows qualitative analysis of the interconnected porous network of the material. Dentin has a natural porosity, the tubules, whose diameter after deproteinization is around 1 μm. The deproteinization creates another porosity due to the collagen removal: our samples present only nano-sized mineral platelets and no collagen fibers anymore.
3.2 Porosities
Figure presents the cumulative volume (presented here in terms of porosity) graph. Chemically deproteinized dentin presents two pore sizes: one due to the tubules around 0.9 μm and the second due to the collagen fibers removal around 10 nm. We observe that the second porosity is greater in volume than the first. The total porosity is 20%. Unfortunately, the porosimeter does not allow us to fully explore the sample porosities. The curve does not reach a plateau at the end of the test (at the highest pressure allowed by the porosimeter). So the porosities under 6nm are not taken into account in our porosity assessment whereas it seems that, due to the shape of the curve, deproteinized dentin has porosities smaller than 6 nm.
3.3 Modulus of elasticity and hardness
Chemical deproteinization seems to reduce the mechanical properties of dentin. According to Vennat et al., dentin presents a hardness of 0.52 GPa and an elastic modulus of 19.32 GPa versus 0.41 GPa and 13.99 GPa for chemically deproteinized dentin. We summarized all our results with those collected in the literature in Table (Pore Size corresponds to the order of magnitude of the largest type of pores – e.g. tubules for deproteinized dentin).
Table 1 Mean hardness, elastic modulus and pore size
4. Conclusions
Chemically deproteinized dentin presents two porosities (around 0.9 μm and 10 nm), with a total porosity of 20% (without considering the porosities smaller than 6nm). Its mechanical properties appear to be close to those of coral. As cells are typically 10 μm large, the internal porosities of deproteinized dentin samples are too small to host cells unlike the gold standard scaffold. The cells will not be able to penetrate the intimacy of our material, but we argue it is not a sufficient reason to reject deproteinized dentin as a potential scaffold. It has indeed been seen in previous studies that the internal porosities are not deeply invaded by cells since they lack of nutriments when they are too far from the periphery of the scaffold. From the mechanical point of view, deproteinized dentin presents a hardness and an elastic modulus similar to those of the coral. The osteoblasts have great chances to present a good development on such hard surfaces. As the optimum scaffold architecture is yet not known, this material could be used as a scaffold for bone tissue engineering despite its small porosities. The next step is to culture cells on deproteinized dentin to fully validate its possible use in bone tissue engineering.
Acknowledgements
To Mrs S. Konate, for her technical assistance.
References
- Chen P, Toroian D, Price P, McKittrick J. 2011. Minerals Form a Continuum Phase in Mature Cancellous Bone. Calcif Tissue Int. 88:351–61.
- David B, Bonnefont-Rousselot D, Oudina K, Degat M, Deschepper M, Viateau V, Bensidhoum M, Oddou C, Petite H. 2011. A Perfusion Bioreactor for Engineering Bone Constructs: An In Vitro and In Vivo Study. Tissue Eng Part C. 17:505–16.
- Discher D, Janmey P, Wang Y. 2005. Tissue Cells Feel and Respond to the Stiffness of the Substrate. Science. 310:1139–43.
- John A, Chamberlain J. 1978. Mechanical Porperties of Coral Skeleton: Compressive Strength and its Adaptive Significance. Paleobiology. 4:419–35.
- Nichikawa T, Okamura T, Masuno K, Tominaga K, Wato M, Kokubu M, Imai K, Takeda S, Hidaka M, Tanaka A. 2009. Physical Characteristics and Interior Structure of Coral Skeleton as a Bone Scaffold Material. J Oral Tissue Engin. 7:121–27.
- Petite H, Viateau V, Bensaid W, Meunier A, De Pollak C, Bourguignon M, Oudina K, Sedel L, Guillemin G. 2000. Tissue-engineered bone regeneration. Nat Biotechnol. 18:959–63.
- Vennat E, Denis M, David B, Attal JP. 2015. A natural biomimetic porous medium mimicking hypomineralized enamel. Dent Mater. 31:225–34.