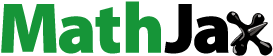
1. Introduction
Whiplash has been defined as a fast motion of the head and neck without a direct force exerted onto the head. A major cause of neck injuries is car accidents, leading to whiplash associated disorders (Laporte et al. Citation2016).
The symptoms include neck pain, fatigue, dizziness, blurred vision, painful lesions (Chalovich & Eisenberg Citation2005), and headaches (Panjabi et al. Citation1998), are non-specific and are reported up to months or years after accidents. Although, the imaging methods, such as MRI or X-Ray, are not sufficient to identify these injuries (Panjabi et al. Citation1998).
The mechanisms of injury is not yet fully understood. It has been shown that precontracted neck muscles, acceleration level and visual inputs would influence the head motion (Eng et al. Citation1997; Brault et al. Citation2000; Kumar et al. Citation2005; Sandoz et al. Citation2016).
The aim of the present study is to model the dynamic of the head/neck complex to understand muscle stabilization strategies. The boundary conditions of the model are based on previous in vivo experiments on 20 volunteers seated on an accelerated sled in frontal direction (Sandoz et al. Citation2016). Some of these volunteers performed EOS Xray images in order to personnalize geometrical parameters. EOS imaging system is a low dose X-rays acquisition system allowing to perform bi-planar acquisition of the subject’s cervical spine. From these X-rays, the 3D personalized geometry of each volunteer’s head neck complex can be built.
2. Methods
The model is a double inverted pendulum multi-body model using Matlab Simulink, representing the upper torso, the neck, and the head (Figure ). Based on EOS X-rays, realistic geometries, and relative position were applied to the body segments. Masses and moments of inertia were based on the literature (Mcconville & Churchill Citation1980).
Figure 1. Simplified representation of the 3-body model superimposed on the initial neutral position of one volunteer on the sled in the experiment.
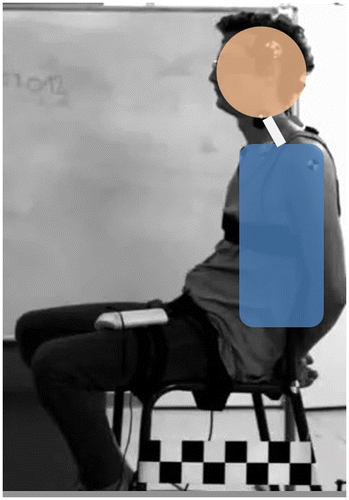
The speed of the sled was imposed to the upper torso of the model, from the experiments. Torques between head/neck and neck/torso are used to stabilize the motion of the segments and to represent the action of the muscles, joints and ligaments (equation 1).(1)
(1)
T is the torque of the joint (head/neck and neck/torso), Δθ is the angle between two adjacent segments, is the coefficient of proportionality, α is the angle of the curvature between torque and Δθ. The values were α = 10 and C0 = 12. Δθ is taken from the simulation according to changing angle of the segments.
Maximal natural Range of Motion (ROM) of the model is based on the real flexion and extension of the neck of each volunteer from the in vivo experiments.
ROM of the head respect to the trunk were obtained depending on the level of acceleration and chosen torques, and compared with inertial measurement units data from the experiments. To evaluate the model, two volunteers data were compared: one male (23yo, 1.83m, 65kg) with a floppy behavior (large head amplitudes), and one male (39yo, 1.80m, 82kg) with stiff behavior (small head amplitudes).
3. Results and discussion
The proposed model is able to represent a realistic behavior of the dynamic flexion/extension of the head and neck for floppy behavior. Five experimental repetitions of identical accelerations (0.25g) were compared to the model using the same boundary conditions. The amplitude of the floppy volunteer, is similar for both the extension and flexion phase (Figure ). The model does not yet consider the initial delay, neither the stabilization phase.
Figure 2. ROM of the head respect to trunk for one male floppy volunteer for 5 identical pulses. Yellow: experiments, red: model.
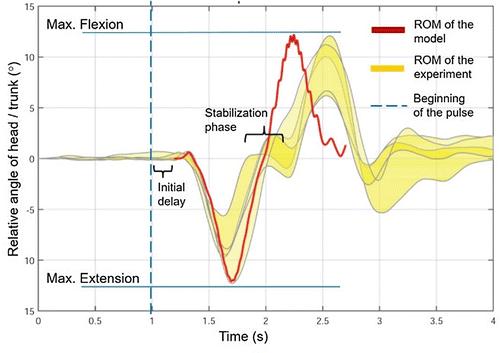
The model was also applied on stiff volunteer. Stiff behavior revealed a less regular motion compare to the floppy behavior in the experiment. The model is not adapted to stiff behavior yet.
The key factor of the model is the torques applied on each joint to find the optimal stabilization. With the current symmetrical torque, the model does not present a stabilization phase (horizontal position between the flexion and the extension at about 1s of the pulse). In order to model the stabilization phase, multiple non-linear parameters will be included.
4. Conclusions
With a simple torque, this 3-body model which include realistic anthropometric data might simulate whiplash-like experiments.
The torque is currently depending on only two parameters (C0 and Δθ). In order to improve the simulation according to the real movements, the torque will take into account multiple other parameters (accelerations, angle velocities…).
References
- Brault JR, Siegmund GP, Wheeler JB. 2000. Cervical muscle response during whiplash: evidence of a lengthening muscle contraction. Clin Biomech. 15:426–435.
- Chalovich JM, Eisenberg E. 2005. NIH public access. Biophys Chem. 257:2432–2437.
- Eng JJ, Winter DA, Patla AE. 1997. Intralimb dynamics simplify reactive control strategies during locomotion. J Biomech. 30:581–588.
- Kumar S, Ferrari R, Narayan Y. 2005. Effect of trunk flexion on cervical muscle EMG to rear impacts. J Orthop Res. 23:1105–1111.
- Laporte S, Wang D, Lecompte J, Blancho S, Sandoz B, Feydy A, Lindberg P, Adrian J, Chiarovano E, de Waele C, et al. 2016. An attempt of early detection of poor outcome after whiplash. Front Neurol.
- Mcconville JT, Churchill T. 1980. Anthropometric relationships of body and body segment moments of inertia. Report. 105.
- Panjabi MM, Cholewicki J, Nibu K, Grauer JN, Babat LB, Dvorak J. 1998. Mechanism of whiplash injury. Clin Biomech. 13:239–249.
- Sandoz B, Rodrigues L, Pitman J, Jem N, Subit D, Vidal P-P, Laporte S. 2016. Vision effect on the head stabilization during controlled linear acceleration of seated volunteers. 4:2016.