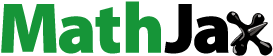
1. Introduction
The temporo-mandibular joint (TMJ) disc is situated between the mandibular condyle and the temporal bone. It is a fibrocartilage tissue allowing smooth jaw movement and serving as a stress distributor. The TMJ disc consists of five characteristic areas, namely the anterior, central, posterior, medial and lateral parts. It is known to have region-dependent material properties (Fernández et al. Citation2013), due to the biochemical content and the fibres distribution, which is found to be concentric in the periphery and uniform in the centre.
Figure 2 a) force displacement curve with Hertz model fitting (R2 = 0.99); b) Young’s modulus; c) squared residuals error fields over the disc picture
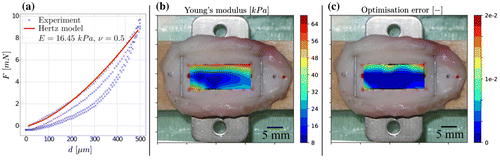
In order to predict its behaviour and/or find specific materials that can be used to substitute the TMJ disc, it is necessary to precisely describe its constitutive law. In this context, a mechanical characterisation taking into account the non-linear, anisotropic and visco-elastic behaviour should be carried out. To deduce it, a numerical approach is required in combination with a rich experimental database. This paper presents the results of a numerical-experimental approach to characterise the local elastic properties through macro-indentation in quasi-static conditions.
2. Methods
2.1 Sample
Porcine TMJ discs were harvested from a pig’s head obtained from a local slaughterhouse. They were carefully extracted using scissor and scalpel. Before testing, the samples were stored at -20°C in physiological solution (NaCl 9 g/L).
2.2 Experimental bench
Indentation tests were carried out using a custom made testing machine consisting of a scale (XS303S, METTLER TOLEDO), a motor (PANDrive, TRINAMIC Motion Control) and a micrometer (Mitutoyo).
During the test, the sample was pinched on a rigid plate and soaked in physiological solution. A spherical indenter of 1.5 mm diameter was used to carry out indentation on 21 positions (Figure 1).
After manually adjusting initial contact between the indenter and the sample, a displacement driven loading was applied with a maximum value of 500 μm over two cycles. The displacement velocity of 1 mm/min was controlled during the tests. Compressive force and displacement were instantaneously recorded thanks to a Delphi compatible cross-platform Lazarus IDE.
2.3 Contact model
Following a finite element preliminary study about the global disc deformation, an analytical Hertz model considering contact between a sphere and a half space was used for faster and easier inverse characterisation of Young’s modulus (E) and Poisson’s ratio (ν). Since the steel indenter’s material properties are very high comparing with the disc tissue stiffness the simplified version of the expression was used (Johnson Citation1985):
where F states for the force, d is the depth of the penetration and R corresponds to the indenter radius.
2.4 Inverse method
Fitting of material parameters was carried out using the Constrained Optimization BY Linear Approximation (COBYLA) method from Scipy Python library, considering the following constrains:
• | Young’s modulus range [1; 109] Pa, | ||||
• | Poisson’s ratio range [0.3; 0.5] |
3. Results and discussion
This experimental bench and protocol allowed characterising the TMJ disc’s anisotropic and visco-elastic behaviour. Figure 2a shows an example of the force as a function of the imposed displacement curve for a point in the centre of the disc. The viscous behaviour of the tissue can be appreciated comparing two cycles illustrated in this figure with a hysteresis between the loading and unloading curves and an accommodation between the first and the second cycle applied in the same conditions. The obtained theoretical curve using the Hertz model (Johnson Citation1985) accurately matches the experimental data with, for this representative case, a regression coefficient of R2 = 0.99. To get a better understanding of the disc’s indentation characterisation, fields of Young’s modulus and error (squared residuals) values have been plotted over the disc picture and are respectively presented in Figure 2b and 2c. Thanks to the identification process, Young’s modulus was found to be region dependent with an average value of 36.17 ± 19.43 kPa (mean ± standard deviation), whereas Poisson’s ratio is constant and equal to 0.5 throughout entire tested area.
Nonetheless the error field reminds the assumption about the infinite half space of the model. Actually, side effects appearing in Figure 2c highlight the limit of this method with high value in Figure 2b. The order of magnitude of Young’s modulus is consistent with other soft tissue characterisation by indentation like CitationDanso et al. (2015) (E = 80 kPa, ν = 0.3) with knee’s meniscus but it is quite low compared to literature on full disc modelling (E = 44.1 MPa, ν = 0.4 in CitationTanaka et al. (2001)). Actually, the developed method aimed to characterise the embedding matrix that surrounds the oriented fibres network and not solicited during indentation test.
4. Conclusions
Macro indentation allows consistent measurement of biomechanical properties on the disc compared to the literature. Due to the complexity of the material an accurate model is required. The inverse method will be further developed using Finite Element Analysis and more complex material models fed with those results as well as further experiments such as tensile test in combination with digital image correlation (CitationBaldit et al. 2014). Finally, combined with three-dimensional imaging techniques, the local material structure related to the anisotropy will be taken into account to describe and predict disc behaviour.
References
- Baldit A, Ambard D, Cherblanc F, Royer P. 2014. Annulus Fibrosus microstructure: an explanation to local heterogeneities. Comput Method Biomec17-S1:38–39.
- Danso EK, Mäkelä JTA, Tanska P, Mononen ME, Honkanen JTJ, Jurvelin JS, Töyräs J, Julkunen P, Korhonen RK. 2015. Characterization of site-specific biomechanical properties of human meniscus-Importance of collagen and fluid on mechanical non linearities. J Biomech. 48:1499–1507.
- Fernández P, Lamela MJ, Ramos A, Fernández-Canteli A, Tanaka E. 2013. The region-dependent dynamic properties of porcine temporomandibular joint disc under unconfined compression. J Biomech. 46:845–848.
- Johnson K. 1985. Contact mechanics. Cambridge University Press.
- Tanaka E, Rodrigo P, Tanaka M, Kawaguchi A, Shibazaki T, Tanne K. 2001. Stress analysis in the TMJ during jaw opening by use of a three-dimensional finite element model based on magnetic resonance images. Int. J. Oral Maxillofac Surg. 30:421–430.