1. Introduction
Lung parenchyma is a multiscale foam-like tissue with several intricate phenomena. During inspiration, the diaphragm creates a depression on the lung cavity forcing air to enter; alveoli dilatation is favored by the presence of surfactant, a biofilm which decreases the surface tension on the inner surface of the alveoli. Lung parenchyma is composed of vasculature, air ducts, alveolar walls, surfactant; a loss of equilibrium between these components can lead to pathologies, such as fibrosis or emphysema, causing a mechanical vicious circle (Hinz and Suki Citation2016). Understanding how lung parenchyma behaves mechanically with its different components is still a challenge. Experimental characterization has been performed at the organ scale (Richardson et al. Citation2017) or on tissue strips (Bel-Brunon et al. Citation2014) but the former includes structural effects while the latter does not allow for realistic loading (inflation), leading to a poor identification of the constitutive law. The aim of the presented protocol is to acquire experimental data related to lung parenchyma inflation to build and identify a component-based constitutive law for this tissue, focusing on small scales (from alveoli to sub-segment). So to reach a sufficient imaging resolution, the protocol is designed for sub-segment lobules.
2. Method
2.1. Lung segment sampling
Samples with an approximate volume of 0.4 L are withdrawn from extracted pig lungs at rest. They are subtrees from the respiratory tree: they can breathe independently from any other lung segment and with no leaks. The withdrawal is conducted by progressively following the respiratory tree, cutting along the bronchus and bronchioles until reaching the desired sample size, around generation 5 or 6. For each extraction, the lung segment is pressurized to identify and remove superfluous matter belonging to other subtrees.
2.2. Set-up
The lung segment is placed in a 27 L enclosure made out of PMMA acrylic and filled with air initially at atmospheric pressure. A syringe, connected to the enclosure, is activated by hand to remove air, in order to mimic the depression caused by the diaphragm. The bronchial end of the sample is connected with the external environment by a Foley catheter to ensure the atmospheric pressure of the air circulating in the lung segment. The Foley catheter passes through the lid in a hermetic seal and holds the sample by its tip, without it touching the sides of the enclosure. The segment is anatomically divided in sub-volumes, called lobules; due to the Foley catheter, some lobules may be disconnected to the atmospheric pressure during the experiment, and therefore would not inflate under external depression.
2.3. Measurements and loading
A pressure sensor is placed inside the enclosure to quantify the depression. A pressure sensor and a flow sensor are used inside the catheter outside the enclosure to characterize the pseudo-breathing. The lung segment is speckled and two cameras placed outside the enclosure record its motion. The surface deformation on the front face of the sample is deduced from these records by a Stereo-DIC analysis using the sequential correlation algorithm from Vic-3D v7.
The 1.5 L syringe used to remove air from the enclosure is alternatively filled and emptied at different rates, simulating a pseudo-breathing on the lung segment. The resulting flow rate inside the segment is between 0.02 and 0.04 L/s; the maximum inspired volume is about 0.15 L.
2.4. CT-imaging and reconstruction
CT images of each sample were acquired at CERMEP (CERMEP—Imagerie du Vivant, Université de Lyon, Bron, France) after loading. The segment is maintained slightly inflated with a Foley catheter to ensure an optimal visibility of the respiratory tree. The CT-scan uses a 55 μm resolution. The outer and inner segment geometry are reconstructed from the images to simulate the experiment. One of the aims of imaging and reconstructing the inner structure is to match the heterogeneous experimental behavior of the segment, which does not inflate homogeneously. Although they are visible on CT-images, lobules could not be automatically segmented and reconstructed; we rely therefore on the respiratory tree segmentation to identify extremities from which lobules could be artificially grown. The respiratory tree is segmented by region growing, then skeletonized and labeled by generation. The lobules are generated by the 3DVoronoï algorithm applied to extremities of the respiratory tree defined by a similar cumulative resistance to air flow. The reconstruction is a gapless assembly of welded lobules that fill the total segment volume extracted from the images.
3. Results and discussion
3.1. Inflation test
Preliminary tests showed that using a 2 mm thick layer of PMMA has no significant influence on the stereoDIC computed fields for the area of interest and an optical set-up typical of our experiment. An example of a strain field is presented in ; it can be computed for most of the anterior side of the segment. Due to geometric effects and regional inflation, this field is very heterogeneous.
Figure 1. Left: Lung segment in the enclosure with applied speckle pattern – Right: Max principal strain pattern during inflation.
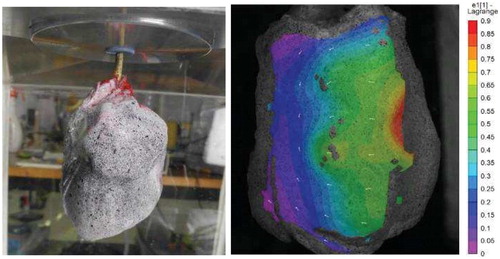
The time evolution of the strain field from DIC is consistent with the measured air flow at the tip of the catheter. There is no observation of any inertial effect of the inflation compared to the pressure profile measured in the enclosure. However, the depression is not perfectly controlled. For the inflation to be representative of the in-vivo mechanism, depression should be driven following a repeatable and realistic breathing cycle in order to avoid viscoelasticity misrepresentation or overload complications. To control such an inflation cycle, the enclosure and syringe can be filled with an incompressible fluid such as water instead of air. The induced refraction is then to be corrected; however, the preliminary study has shown that even with water, refraction effects on the stereo-DIC results are negligible for our experiments.
3.2. Geometric reconstruction
As shown in , and contrary to human anatomy, the respiratory tree does not exhibit symmetric divisions. So tree extremities of the same generation do not seem relevant as seeds to grow lobules of similar volume. Tree cumulative resistance seems more relevant, based on a visual comparison between 3D lobule reconstruction () and segment CTimages. However this observation requires further quantitative analysis.
Figure 2. Reconstruction of a segment (a) tree structure (b) lobules generated as Voronoï cells from an equivalent cumulative resistance criterion.
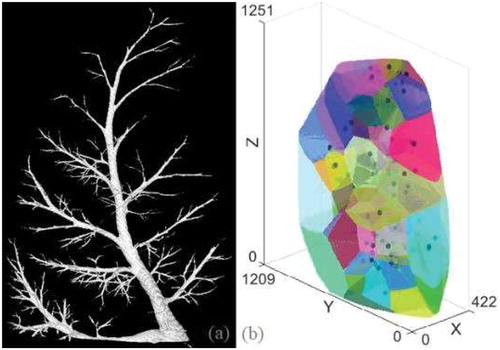
CT-scan images are captured using horizontally positioned segments. To improve the protocol robustness, the horizontal position can be used during the experiments so that experimental and imaging boundary conditions are the same (particularly gravity effect) and therefore avoid any registration step.
4. Conclusions
With this first set-up, we obtained more insight into lung segment inflation which is useful to increase robustness of the existing set-up. We also plan to evaluate inflation before and after bronchoalveolar lavage to evaluate the mechanical contribution of surfactant to the resulting lung parenchyma behavior.
References
- Bel-Brunon A, Kehl S, Martin C, Uhlig S, Wall WA. 2014. Numerical identification method for the non-linear viscoelastic compressible behavior of soft tissue using uniaxial tensile tests and image registration - application to rat lung parenchyma. J Mech Behav Biomed Mater. 29:360–374.
- Hinz B, Suki B. 2016. Does breathing amplify fibrosis? Am J Respir Crit Care Med. 114(1):9–11.
- Richardson S, Gamage TPB, HajiRassouliha A, Jackson T, Hedges K, Clark A, Taberner A, Tawhai MH, Nielsen PM. 2017. Towards a Real-Time Full-Field Stereoscopic Imaging System for Tracking Lung Surface Deformation Under Pressure Controlled Ventilation. In Int Conf Med Image Comput Comput Assist Interv. 119–130.