1. Introduction
Orthodontic treatments are based on a prolonged application of mechanical forces on the teeth through orthodontic appliances, leading to tooth movement due to the remodeling of the surrounding bone. Bone response is dependent on the biological reactions occurring in the periodontal ligament (PDL) and more specifically on those related to vascular changes (Pavlin and Gluhak-Heinrich Citation2001). Hence, optimal forces and moments are required to obtain the desired tooth displacements without generating deleterious effects. Although the biological events occurring during orthodontic tooth movement are nowadays better understood, the correlation between orthodontic force systems, desmodontal reactions and bone remodeling is not well established (Meikle Citation2005). Little is known about the forces and moments developed on more than two adjacent teeth (Badawi et al. Citation2009).
In this work, we focus our analysis on a buccal upper right canine in infraclusion and extract the experimental measurements of the mechanical forces developed onto the complete dental arch. These are then transferred into a numerical finite element (FE) model to determine the PDL deformation and hence, through a theoretical numerical model, quantify the cellular mechanobiological reactions at the root of orthodontic bone remodeling.
2. Methods
2.1. Experiments
An experimental setup was developed in order to quantify the developed mechanical forces in 3D on each tooth of a maxillary dental arch when inserting an initial 0.014” Nickel Titanium orthodontic archwire within the brackets (Wagner et al. Citation2017a). Once the forces were measured, static equilibrium of the dental arch was considered to obtain the moments developed at the center of each bracket (Wagner et al. Citation2017b). In parallel, histological studies were carried out to extract the cellular distribution and densities within the PDL and adjacent bone (Wagner et al. Citation2018). These information’s made possible correlations between mechanical forces and cellular activations.
2.2. Numerical modeling
A 3D FE model (FE1) was developed () to predict the reaction forces applied on the teeth in a real clinical situation, based on the superelastic constitutive law of the orthodontic wire. This work also accounted for friction between wire and brackets which was determined through the experimental setup.
Figure 1. 3D FE model of a full maxillary arch upon insertion of the first archwire within the brackets.
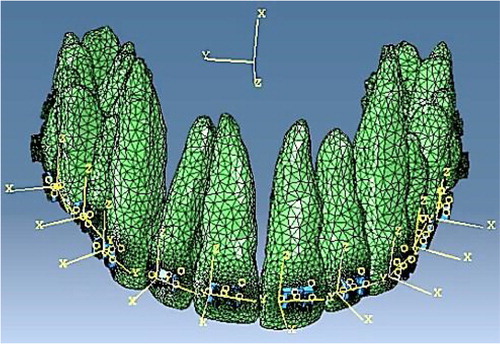
The mechanical force system applied onto the teeth induces a deformation of the PDL. In the compression area, the PDL vascularization is partially occluded causing a decrease in oxygen concentration which is known to increase the proliferation of osteoclasts (Utting et al. Citation2006). On the other hand, in the tensile zone, the oxygen concentration will increase due to PDL stretching, which is the cause of proliferation of osteoblasts (Arnett et al. Citation2003). These key factors are assumed to be at the origin of the bone remodeling phenomena in orthodontics. With this understanding of the PDL physiological behavior, the impact of the oxygen effect on the cells’ evolutions is highlighted (George et al. Citation2018). The phenomena are integrated within a second FE model (FE2) (Spingarn et al. Citation2018), accounting for the mechanobiological coupling between mechanical forces and cellular activation, in order to determine the initiation of the bone remodeling phenomena and tooth movements.
3. Results and discussion
From the applied displacements on the developed experimental setup, forces were measured on three teeth, namely the upper first right premolar, the upper right canine, and the upper right lateral incisor. Once these were extracted, the FE1 model allowed us to define in 3D the forces applied on all teeth of the maxillary arch. Using simple static equilibrium, moments were subsequently calculated for all teeth of the dental arch. The results are presented in for the three teeth of interest.
Table 1. Developed forces and moments in 3D on the upper first right premolar, upper right canine, and upper right incisor as determined from experiments and by the FE1 numerical model.
Once the forces and moments were quantified, they were integrated in the second FE model (FE2) to predict the oxygen variation within the PDL and quantify the cells’ multiplication and migration. The preliminary results show, through a multiphysics algorithm, the initial mechanisms of tooth displacement and preliminary bone remodeling.
4. Conclusion
A simplified theoretical numerical model was developed for the prediction of orthodontic bone remodeling in a case of an orthodontic alignment of a buccal infraoccluded upper right canine. The models were implemented using experimental data of forces and moments measured on an in house developed setup, coupled with histological data of the PDL. Results show preliminary bone remodeling during the initial alignment stage of the orthodontic treatment. More work is still needed to predict long term tooth displacement for practical applications.
Acknowledgements
The authors wish to thank Dr. Morad Bensidhoum and his team of the B3OA Laboratory of osteo-articular Biology, Bioengineering, and Bio-imagery, for help with the histological tests providing the cellular content of the PDL.
References
- Arnett TR, Gibbons DC, Utting JC, Orriss IR, Hoebertz A, Rosendaal M, Meghji S. 2003. Hypoxia is a major stimulator of osteoclast formation and bone resorption. J Cell Physiol. 196(1):2–8.
- Badawi HM, Toogood RW, Carey JPR, Heo G, Major PW. 2009. Three-dimensional orthodontic force measurements. Am J Orthod Dentofac Orthop. 136(4):518–528.
- George D, Allena R, Rémond Y. 2018. Cell nutriments and motility for mechanobiological bone remodeling in the context of orthodontic periodontal ligament deformation. J Cell Immu. 4:26–29.
- Meikle MC. 2005. The tissue, cellular, and molecular regulation of orthodontic tooth movement: 100 years after Carl Sandstedt. Eur J Orthod. 28(3):221–240.
- Pavlin D, Gluhak-Heinrich J. 2001. Effect of mechanical loading on periodontal cells. Crit Rev Oral Biol Med. 12(5):414–424.
- Spingarn C, Wagner D, Rémond Y, George D. 2018. Theoretical modeling of the oxygen diffusion effects within the periodontal ligament for orthodontic tooth displacement. J Cell Immun. 4:44–47.
- Utting JC, Robins SP, Brandao-Burch A, Orriss IR, Behar J, Arnett TR. 2006. Hypoxia inhibits the growth, differentiation and bone-forming capacity of rat osteoblasts. Exp Cell Res. 312(10):1693–1702.
- Wagner D, Bolender Y, Rémond Y, George D. 2017a. Experimental quantification of the mechanical forces and moments applied on three adjacent orthodontic brackets. Biomed Mater Eng. 28(S1):S179–S184.
- Wagner D, Bolender Y, Rémond Y, George D. 2017b. Mechanical equilibrium of forces and moments applied on orthodontic brackets of a dental arch: correlation with literature data on two and three adjacent teeth. Biomed Mater Eng. 28(S1):S169–S177.
- Wagner D, Hafci HE, Bensidhoum M, Bolender Y, Rémond Y, George D. 2018. Periodontal ligament histology for orthodontic bone remodeling: first quantification. J Cell Immu. 4:41–43.