1. Introduction
The hand musculoskeletal system is composed of many joints and more than thirty muscles which enables us to grasp and manipulate a wide variety of object. The specific configuration of the hand musculature, including extrinsic muscles originating in the forearm, results in mechanical couplings between finger forces and the wrist joint equilibrium (Snijders et al. Citation1987). More precisely, it has been shown that the position of the wrist has a direct influence on the maximal force the fingers can exert (O’Driscoll et al. 1992; Li Citation2002). For instance, during grasping tasks, participants spontaneously chose a specific wrist position that resulted in the highest grip force values (O’Driscoll et al. 1992). This spontaneous posture has been assumed to correspond to a more advantageous configuration of the force-length behaviour of extrinsic finger flexors (Li Citation2002). Nevertheless, because the in vivo evaluation of muscle forces is difficult, no data has confirmed this hypothesis yet. To address this problem, this study combined an experimental protocol and a musculoskeletal model to assess the influence of the wrist posture on the maximal voluntary grip force and the length and force of 4 muscle groups (Wrist flexors, Finger flexors, Wrist extensors, Finger extensors).
2. Methods
2.1. Experimental procedure
Sixteen volunteers (age: 21.7 ± 2.5 years, hand length: 18.4 ± 1.0 cm) participated in the experimental protocol. They were instructed to exert maximum grip force (MGF) on a cylindrical handle. Three diameters and four wrist postures were tested. One posture was freely chosen by the participants, referred to as “spontaneous” (S) position. The three others were imposed to the participants and consisted in a neutral (N) posture (0° of flexion and deviation), a maximal flexion (F) and a maximal extension (E). Each participant performed two acquisition per condition for a total of 24 MGF. The exerted grip force was recorded with an instrumented cylindrical dynamometer consisting of 6 beams each providing a measure of force using strain gauges (Sixaxes, Argenteuil, FR). Simultaneously, the posture of the wrist and index finger was recorded using a 7-camera motion capture system (Vicon, Oxford, UK; freq: 100 Hz) tracking fourteen markers on the hand. Surface electromyographic (EMG) signals of four extrinsic muscle were also simultaneously acquired (MP-150, BIOPAC Systems, Inc., Goleta, CA, USA). The investigated muscles were the index finger flexor digitorum superficialis (FDS), extensor digitorum communis (EDC), the flexor carpi radialis (FCR) and extensor carpi radialis (ECRL).
For each trial, the maximal grip force was calculated as the mean of the recorded grip force on a 500 ms window centred on the grip force peak. For each subject, this value was then normalized by the maximal value across all trials. Marker positions were first averaged on a window centred on the grip force peak. For each trial, index and wrist joint angles were calculated by first computing segment coordinate systems of the proximal and distal segment and then extracting Cardan angles from the relative orientation matrix using a ZXY sequence. For each trial, the activation level was determined for each muscle by calculating RMS on the same window and normalized by the maximum RMS value across all trials. For each posture, only the diameter corresponding to the highest grip force of the participant was kept for analysis.
2.2. Musculoskeletal model
From the data acquired during the protocol, the current length and force of the studied four muscles were estimated using a musculoskeletal model of the wrist and index finger that consisted of two steps. First, the model estimated the tendon excursion from joint angles using anatomical data and geometrical models (Goislard de Monsabert et al. Citation2018). Second, the length and force of each muscle were evaluated using activation level and tendon excursion via polynomial equations describing the force-length-activation relationships determined from in-vivo data in a previous study (Hauraix et al. Citation2018).
3. Results and discussion
The results of the experiment showed that the spontaneous wrist posture was reproducible across participants (−29.9 ± 9.33°) and resulted in the highest normalised maximum grip forces (0.993 ± 0.02) (). The loss of grip force was about 12.67 ± 6.38% in extension and 37.97 ± 12.89% in flexion which is in agreement with the usual inverted bell-shape curve found in the literature (O’Driscoll et al. 1992). Remarkably, when considering the results at the muscle levels, finger flexors was the least affected by wrist angle changes compared to other muscles and was close to its optimal point in both force levels (0.86 ± 0.091) and lengthening (from 0.96 to 1.03). This result is in contradiction with the usual hypothesis of a direct link between grip force variation and finger flexors length. The most affected muscle was ECRL in lengthening (from 0.92 to 1.21) with a force level of 0.63 ± 0.16. Moreover, the variations follow variations of MGF as the ECRL force is the lowest in extension and flexion. It can be surprising, as ECRL works as an antagonist, although this result corroborates the ones of studies showing the importance of this muscle in stabilising the flexion moments at the wrist joint inherently created by the finger flexors when they exert forces on the grasped object(Snijders et al. Citation1987).
Figure 1. Left pannel presents the normalised maximum grip force against the wrist positions (Mean ± 1SD). Middle and right pannel presents normalised force against length of the FDS and ECRL, resepectively, for each recorded point. The black line represents the force-length relationship at activation = 1. Muscles are in their optimal length at 1. ◆: spontaneous posture. ▼ : extension posture. ▲ : flexion posture. ●: neutral posture.
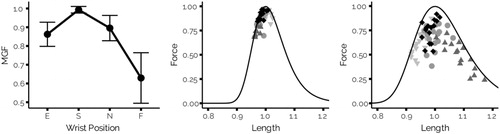
When plotting the sum across the four muscles of the differences between their muscle length and their optimal length (), it appears that the spontaneous posture corresponds to the lowest points and thus the configuration that is the most advantageous for all muscles.
4. Conclusions
Thanks to the combination of both experimental data and musculoskeletal modelling, the influence of wrist position on force production at the muscle and the effector levels were studied. It is the first time that data are provided to examine the hypothesis stating that wrist position spontaneously taken by participants to produce force corresponds to more advantageous force-production capacities of finger flexor muscles. The results of the present study demonstrated that the optimal wrist posture seems to correspond to an optimal point which takes into account the capacities of all muscles crossing the wrist. This study is a first step toward a better understanding of biomechanical properties underlying grasping control and provide relevant information for application in ergonomic design.
References
- Goislard de Monsabert BGD, Edwards D, Shah D, Kedgley A. 2018. Importance of consistent datasets in musculoskeletal modelling: a study of the hand and wrist. Ann Biomed Eng. 46(1):71–85.
- Hauraix H, Goislard De Monsabert B, Herbaut A, Berton E, Vigouroux L. 2018. Force–length relationship modeling of wrist and finger flexor muscles. Med Sci Sports Exerc. 50:2311–2321.
- Li Z-M. 2002. The influence of wrist position on individual finger forces during forceful grip. J Hand Surg. 27(5):886–896.
- O'Driscoll S W, Horii E, Ness R, Cahalan T D, Richards R R, An K-N. 1992. The relationship between wrist position, grasp size, and grip strength. J Hand Surg. 17(1):169–177.
- Snijders CJ, Volkers AC, Mechelse K, Vleeming A. 1987. Provocation of epicondylalgia lateralis (tennis elbow) by power grip or pinching. Med Sci Sports Exerc. 19(5):518–523.