1. Introduction
Postural stability and performance during dynamical motor tasks depends on at least two biomechanical factors, namely the mobility of the postural chain (Bouisset and Do Citation2008) and the base of support (BOS) dimension (Yiou et al. Citation2017). Constraining one of these factors may alter balance and/or motor performance, unless motor adaptations are developed. One condition that simultaneously alters both factors is the use of high-heel (HH) shoes, which forces the feet into a plantarflexed position and induces a reduction in the BOS dimension. Recent studies showed that musculoskeletal disorders and feet deformities with HH wear are related to increased forefoot pressure (Speksnijder et al. Citation2005). Wearing rigid HH shoes also increases knee flexion and lumbar lordosis, thus inducing a modification of gait pattern (Wiedemeijer and Otten Citation2018). However, to date, the question how HH shoes wear may influence dynamical postural control during locomotor task remains to be clarified. In the present study, the gait initiation (GI) paradigm, which is the transient phase between quiet standing posture and ongoing locomotion (Brenière et al. Citation1987), was used to address this question. GI is classically divided into a postural phase (corresponding to the “Anticipatory Postural Adjustments”, APAs) followed by a step-execution phase ending at the time of swing foot contact. Postural stability during GI is known to depend on the capacity of the central nervous system (CNS) to develop these APAs and to actively brake the vertical fall of the center of mass (COM) during step-execution (Yiou et al. Citation2017). The present study was designed to test the hypothesis that the wear of HH shoes induces mechanical constraints on ankle mobility and BOS dimension that alter the CNS capacity to generate convenient APAs and brake the COM fall, with consequent increased fall risks.
2. Methods
2.1. Population
Thirteen healthy young adults female performed series of ten gait initiation trials on a force-plate (0.9 x 1.80 m, AMTI, Watertown, USA), in the three following conditions: without HH shoes (“control”), with 6 cm high-heel shoes (“HH-6”) and with 9 cm high-heel shoes (“HH-9”). These conditions were randomly assigned across subjects. GI was performed at a self-paced speed.
2.1. Biomechanical parameters
The following biomechanical parameters were quantified in each trial: ML/AP BOS size in the initial posture, ML size of the BOS at the rear feet level (corresponding to the ML distance between the heels or the stilettos) in the initial posture, APAs duration, APAs amplitude (peaks of center of pressure (COP) and COM velocity at toe off in the sagittal plane), step length, step-execution phase duration, anteroposterior (AP) COM velocity at the time of foot contact (step performance), mediolateral (ML) margin-of-stability (MOS, indicator of stability, Hof et al. Citation2005) at the time of foot contact, and an indicator of the capacity to actively brake the COM fall, the “braking index”. In addition, the ML stiffness of the stance leg during step-execution was computed thanks to GI mechanical modelling. The COM velocity was obtained with simple integration of the COM acceleration. COM acceleration was obtained thanks to the second Newton’s law. In each condition, mean ± 1 SD were computed for each variable. The normality of data was checked using the Kolmogorov-Smirnov test and the homogeneity of variances was checked using the Bartlett test. One way repeated measures ANOVAs with the condition (“control”, “HH-6”, “HH-9”) as within subject factor was used. A significant outcome was followed-up with the Tukey post hoc test.
3. Results and discussion
Biomechanical traces. In each condition, toe off was systematically preceded by APAs, during which the COP was shifted backwards and towards the swing leg. The COM velocity was directed forwardly and toward the stance leg during these APAs. The vertical COM velocity was “V” shaped, indicating COM braking before foot contact.
Initial posture. There was a significant effect of the condition on the AP BOS size and on the ML BOS size measured at the rear feet level. In contrast, the ML BOS size remained unchanged.
APAs. There was a significant effect of the condition on APAs duration (F2,24 = 4.1, p < 0.05), AP (F2,24 = 10.2, p < 0.001) and ML (F2,24 = 5.2, p < 0.05) COP peak amplitude, AP (F2,24 = 10.2, p < 0.001) and ML (F2,24 = 6.8, p < 0.01) COM velocity at toe off.
Step execution. There was a significant effect of the condition on step performance (F2,24 = 12.4, p < 0.001), stance leg stiffness (F2,24 = 4.4, p < 0.05) but not on step length nor duration.
Stability. There was a significant effect of the condition on the braking index (F2,24 = 31.6, p < 0.001) but not on the MOS. Globally, post hoc tests showed that the mean values of each of these variables significantly decreased from the “control” to the “HH-9” condition ().
Figure 1. Effect of HH on selected biomechanical parameters. *, ** and *** indicate a significant difference between bars with p < 0.05, p < 0.01 and p < 0.001, respectively.
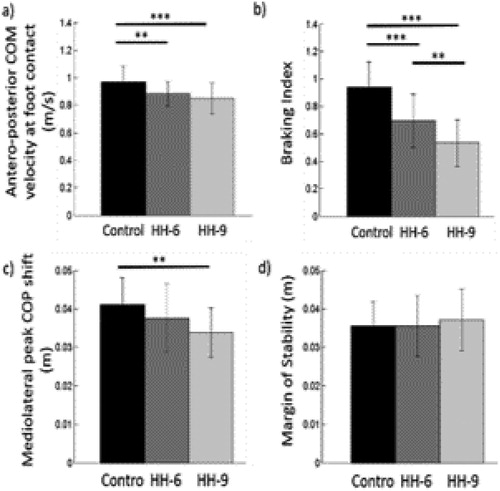
The reduced ML and AP APAs amplitude could be ascribed to the smaller possibility of COP shift in the two HH conditions compared to the control condition. The AP BOS size in the initial posture was indeed 25 ± 2 cm in the control condition and fell to 23 ± 2 cm and 21 ± 2 cm in the HH-6 and HH-9 condition, respectively. The ML BOS size at the rear feet was 30 ± 2 cm in the control condition and fell to 25 ± 2 cm in both the HH-6 and HH-9 condition. The lower AP BOS size restricted the possibility of COP backward shift during APAs in the two HH conditions, which resulted in the lower step performance. The result that the ML MOS remained invariant across the conditions - despite the lower ML APAs amplitude in the two HH conditions - could likely be ascribed to an effect of the reduced leg stiffness in these two test conditions. Reduced leg stiffness is indeed known to have a positive effect on ML stability. We thus propose that this reduced stance leg stiffness (probably induced by modulating the level of stance leg muscles contraction) reflects strategy to compensate for the altered capacity to generate ML APAs in the two HH conditions. In contrast, the capacity to brake the vertical COM fall was affected by the HH use, which could be ascribed to the lower stance ankle mobility. This finding is in accordance with recent study on the effect of a rigid ankle orthosis wear on this stability parameter (Delafontaine et al. Citation2017).
4. Conclusions
This study shows that the use of HH shoes induces drastic changes in the GI postural organization, altering step performance and vertical COM braking, thus increasing the risk of fall. These changes increased with the HH height. Subjects were however able to maintain the ML stability unchanged thanks to adaptation of stance leg stiffness. MOS invariance is in line with our previous studies suggesting that the MOS is a controlled stability parameter (Yiou et al. Citation2017). Future studies will investigate whether female top models are able develop alternative and more efficient strategies to maintain stability and step performance.
References
- Bouisset S, Do MC. 2008. Posture, dynamic stability, and voluntary movement. Neurophysiol Clin. 38(6):345–362.
- Brenière Y, Do MC, Bouisset S. 1987. Are dynamic phenomena prior to stepping essential to walking?. J Mot Behav. 19(1):62–76.
- Delafontaine A, Gagey O, Colnaghi S, Do MC, Honeine JL. 2017. Rigid ankle foot orthosis deteriorates mediolateral balance control and vertical braking during gait initiation. Front Hum Neurosci. 11(214).
- Hof AL, Gazendam MG, Sinke WE. 2005. The condition for dynamic stability. J Biomech. 38(1):1–8.
- Speksnijder CM, Vd Munckhof RJH, Moonen SAFCM, Walenkamp GHIM. 2005. The higher the heel the higher the forefoot-pressure in ten healthy women. Foot. 15(1):17–21.
- Wiedemeijer MM, Otten E. 2018. Effects of high heeled shoes on gait. A review. Gait Posture. 61:423–430.
- Yiou E, Caderby T, Delafontaine A, Fourcade P, Honeine J-L. 2017. Balance control during gait initiation: State-of-the-art and research perspectives. WJO. 8(11):815–828.