1. Introduction
Assessing the functional ability depending on the prosthesis fitting of individuals with transfemoral amputation (TFA) is critical for overall rehabilitation and prosthetic care. Gait analysis could provide an overwhelming number of biomechanical parameters. We argue that margins of stability (MoS) could be critical indicators of TFA’s control strategy. A study revealed statistically larger MoS on the prosthetic limb when comparing medial-lateral MoS for six TFAs fitted with a socket-suspended prostheses and six able- bodied during treadmill walking (Hof et al. Citation2007). Another study showed limited and non-significant differences when comparing anterior-posterior MoS for ten TFA fitted with mechanical and microprocessor-controlled knees during walking overground (Prinsen et al. Citation2017). There is a need to report MoS more widely, particularly for TFA fitted with bone-anchored prosthesis as osseoperception should improve control. A single case study demonstrated that the load applied by bone-anchored prosthesis fitted with microprocessor-controlled was attenuated by 10-20% compared to mechanical knee (Frossard et al. Citation2013). However, the impact of these loading differences on the overall gait pattern, including medial-lateral MoS, remains unknown.
We hypothesized that medial-lateral MoS produced by this participant may also reveal differences between bone-anchored prostheses fitted mechanical and microprocessor-controlled knees.
2. Methods
2.1. Participant and experiments
This retrospective single-case study involved an active male (41 yr, 1.77 m, 96.6 kg) with a right unilateral transfemoral residuum fitted with screw-type osseointegrated fixation eight years before the study (Frossard et al. Citation2013). The participant walked in straight level walkway at self-selected speed fitted with a mechanical Total Knee (7 trials) and microprocessor-controlled C-Leg (8 trials) knees as well as 13 markers on the legs, arms, and trunk.
Kinematic and dynamics data were captured with a 6- camera motion capture system (Qualisys) and two force plates (Kistler), respectively.
2.2. Margins of stability
Data was processed with a custom made Python program. The centre of pressure (CoP) was directly obtained from the two force plates. A nine-segment model (Tisserand et al. Citation2016) was used for the computation of the centre of mass (CoM) and extrapolated centre of mass (XCoM). XCoM corresponds to CoM position plus a certain ratio of its velocity (representative of the human inverted pendulum’s eigenfrequency) (Hof et al. Citation2007).
The medial-lateral MoS on both prosthetic and sound sides, corresponded to the distance in the medio-lateral direction between XCoM and CoP at the time of the contralateral toe-off ().
Figure 1. Mean trajectories (and standard deviation) of CoM, CoM, and XCoM for all trials with an illustration of the typical prosthetic gait cycle (Rajťúková et al. Citation2014).
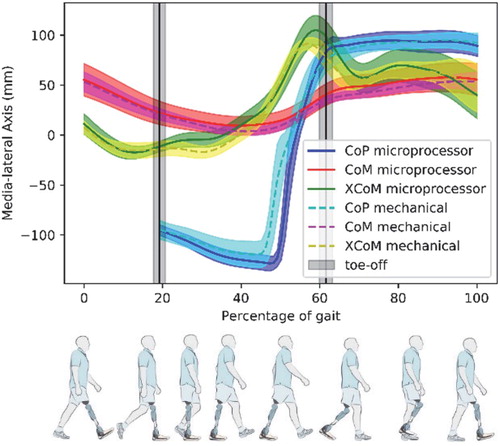
3. Results and discussion
Expressed in percentage of gait cycle in , the CoP, CoM, and XCoM trajectories demonstrated a very low inter-trial variability.
The CoP trajectories corresponding to the mechanical and microprocessor-controlled knees during single stance on either the prosthetic or sound limb could be superimposed while the body weight transfer from the prosthetic to the sound limb during the double support was slightly different (i.e. earlier and longer with the mechanical knee).
The CoM trajectories corresponding to the mechanical and microprocessor-controlled knees could be almost superimposed for the whole gait cycle. Altogether, this consistency and the very low inter-trial variability confirmed that the participant was familiar with both knees. The CoM was notably asymmetrical and shifted over the sound limb, suggesting high compensation mechanisms (i.e. trunk movement, non-straight walking).
The XCoM trajectories showed mainly differences in the middle and late stance on the prosthetic side as translated by the MoS presented in .
TABLE 1. Medial-lateral margin of stability (MoS) on both prosthetic and sound sides.
Obviously, the generalization of these outcomes for this single case is limited. More transferable is the relevance of this parameter and the methodology used to determine the MoS despite typical experimental limitations (e.g. marker placements, force plate targeting, estimation of prosthetic inertial parameters, nine-segment model).
Medial-lateral MoS on the prosthetic side were about 10% smaller with the mechanical knee compared to the microprocessor-controlled knee. However, these MoS remain very large compared to the sound side. The small and even negative MoS on the sound side might indicate a higher demand for adjustments after the initial contact (i.e. ankle muscle activations) (Hof et al. Citation2007). MoS for the participant were larger on the prosthetic side and smaller on the sound side compared to the literature (Hof et al. Citation2007). The walking speed was similar to this study but the differences can be due to other confounders (e.g. setup for walking conditions, prosthetic attachment, prosthetic foot, residuum length, and acclimation). It is also likely that the participant presented high compensation mechanisms which are not captured by the linear pendulum model on which MoS analysis is based (Hof et al. Citation2007). Yet, this participant fitted with a microprocessor-controlled knee demonstrated reduced load applied by bone-anchored prosthesis (Frossard et al. Citation2013), but enlarged medial-lateral MoS on the prosthetic side (negative on the sound side), suggesting a worsen balance control.
4. Conclusions
Our hypothesis that medial-lateral MoS might reveal differences between prostheses was only partially verified. This might be a reflection of the sensitivity of MoS to compensation mechanisms (i.e. trunk movement, non-straight walking) over design of the prosthetic knee.
In all cases, this study confirmed that medial-lateral MoS is a worthwhile parameter to consider when analysing the control strategy of individuals with a TFA.
Acknowledgements
Hubert Curien Partnership (French Ministry of Foreign Affairs), PHC FASIC # 39370PM. The authors wish to thanks Prof R. Brånemark from Massachusetts Institute of Technology, in Cambridge, USA, as well as Drs K. Hagberg and E. Haggstrom from Sahlgrenska University Hospital, in Gothenburg, Sweden for their contribution to the data collection.
References
- Frossard L, Häggström E, Hagberg K, Brånemark R. 2013. Load applied on bone-anchored transfemoral prosthesis: characterization of a prosthesis-a pilot study. J Rehabil Res Dev. 50(5):619–634.
- Hof AL, van Bockel RM, Schoppen T, Postema K. 2007. Control of lateral balance in walking: experimental findings in normal subjects and above-knee amputees. Gait Posture. 25(2):250–258.
- Prinsen EC, Nederhand MJ, Koopman BF, Rietman JS. 2017. The influence of a user-adaptive prosthetic knee on planned gait termination. Proceedings of the International Conference on Rehabilitation Robotics (ICORR), p. 1254–1259.
- Rajťúková V, Michalíková M, Bednarčíková L, Balogová A, Živčák J. 2014. Biomechanics of lower limb prostheses. Proc Eng. 96:382–391.
- Tisserand R, Robert T, Dumas R, Chèze L. 2016. A simplified marker set to define the center of mass for stability analysis in dynamic situations. Gait Posture. 48:64–67.