Keywords:
1. Introduction
Craniotomy is a well-known procedure in neurosurgery, during which a bone flap is removed, and is mainly used to save patients with severe head trauma (Chaikoolvatana and Sripech Citation2007). They can also sometimes be performed for inserting implants, like a cochlear implant or the RNS system (NeuroPace, Inc) (Heck et al. Citation2014). The international standard EN 45502-2-3:2010 for cochlear implants requires a 2.5 J hammer test. It is intended to assess the implant’s mechanical resistance against everyday impact scenarios as falls or hits on the head. Human head resistance against impact is reported in the literature between 16 and 28 J in average (Yoganandan et al. Citation1995, Verschueren et al. Citation2007; Delye et al. Citation2007). However, to the authors’ knowledge, the influence of a craniotomy on the skull’s mechanical resistance to impact has never been addressed. Still, studies dealing with the design of cranial implants for skull reconstruction report a higher risk of skull fracture at the interface between the skull and the implant (Tsouknidas et al. Citation2011; Garcia-Gonzalez et al. Citation2017). This raises questions about the influence of a craniotomy on the mechanical resistance of the skull against impact loadings.
The present study gives insights into the effect of a 50 mm-diameter craniotomy on the resistance of the lamb skulls to a 2.8 J impact loading.
2. Methods
2.1. Specimen preparation
Eight lamb heads were obtained from the slaughterhouse and stored at −20 °C. They were thawed for an average of 48 h prior to experiments. The scalp was removed from each specimen and a 3 mm-thick silicone sheet (MED6210, Nusil™) was fixed onto the skull using screws. The specimen was then fixed into cement and the obtained block was fixed to the concrete wall (). The heads were first tested as described. Afterwards, a 50 mm-diameter craniotomy was performed into the skulls using a hole saw ().
2.2. Experimental setup
A pendulum hammer test bench was used for the experiments. The 1,61kg-hammer, with a 25mmradius impact area, was dropped from 20 cm high as to obtain a 2.8 J impact.
First, the complete heads were tested and the skulls were analysed visually for macroscopic lesions. The craniotomy was then performed and the hammer test was carried out on the craniotomy edge ().
2.3. Data measurement
A high-speed camera (MotionBLITZ EoSens® Cube7) recorded the hammer displacement at 5500fps. An image subtraction method was used to compute the horizontal displacement of the hammer in pixels. The average displacement was computed at each time step as the average pixel displacement over the 750 lines of the image, at a pixel-resolution of 0.1 mm. The hammer velocity was then computed.
3. Results and discussion
No macroscopic lesion was observed for the heads without craniotomy. The hammer velocity curves are shown on . Impacts lasted between around 5 and 8 ms and ejection velocities were repeatable over the specimens, with an average of 1.23 m/s. The final energy was therefore of 1.2 J, leading to an energy absorption during impact of 57%.
Figure 2. Hammer velocities for both hammer tests specimen groups. (a) Heads without craniotomy. (b) Heads with craniotomy. (c) Cracks on a head with craniotomy after impact.
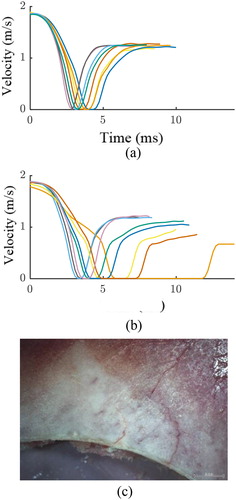
The hammer velocity of the impacts on the heads with craniotomy is shown on . Three of the specimens depict the same curve aspect as the tests without craniotomy, and ejection velocities are stable between 1.18 and 1.22 m/s. These heads did not show any macroscopic lesion after the test.
The five remaining specimens depicted a longer impact duration (>7ms) and lower ejection velocity (0.67–1.11 m/s). Especially, one depicted an impact duration over 12 ms; a major skull fracture was visible for this specimen. The four other specimens depicted cracks departing from the craniotomy (). The diversity of responses obtained among the heads with craniotomy may be explained by the variability of the thickness. µCTs performed on the removed bone flaps revealed that the average thicknesses were comprised between 2.4 and 5.4 mm. Moreover, the standard deviation measured over each bone flap varied between 0.9 and 1.8 mm.
During a craniotomy, part of the skull is removed, weakening it against impacts. Tsouknidas et al. (Citation2011) and Garcia Gonzalez et al. (2017) reported skull weakening at the interface with a skull reconstruction implant.
4. Conclusions
Though impact scenarios on the head remain rare cases, a craniotomy seems to make the skull less resistant to moderate impacts of the order of magnitude of 2.8 J, leading to cracks or even fractures. This aspect could be taken into account when designing skull implants as to increase skull safety.
References
- Chaikoolvatana A, Sripech S. 2007. Quality of life of road accident head-injured patients after craniotomy. Southeast Asian J Trop Med Public Health. 38(4):761–768.
- Heck CN, King-Stephens D, Massey AD, Nair DR, Jobst BC, Barkley GL, Salanova V, Cole AJ, Smith MC, Gwinn RP, et al. 2014. Two-year seizure reduction in adults with medically intractable partial onset epilepsy treated with responsive neurostimulation: final results of the RNS System Pivotal trial. Epilepsia. 55(3):432–441.
- Yoganandan N, Pintar FA, Sances JA, Walsh PR, Ewing CL, Thomas DJ, Snyder RG. 1995. Biomechanics of skull fracture. J Neurotrauma. 12(4):659–668.
- Verschueren P, Delye H, Depreitere B, Van Lierde C, Haex B, Berckmans D, Verpoest I, Goffin J, Vander Sloten J, Van der Perre G. 2007. A new test set-up for skull fracture characterisation. J Biomech. 40(15):3389–3396.
- Delye H, Verschueren P, Depreitere B, Verpoest I, Berckmans D, Vander Sloten J, Van der Perre G, Goffin J. 2007. Biomechanics of frontal skull fracture. J Neurotrauma. 24(10):1576–1586.
- Tsouknidas A, Maropoulos S, Savvakis S, Michailidis N. 2011. FEM assisted evaluation of PMMA and Ti6Al4V as materials for cranioplasty resulting mechanical behaviour and the neurocranial protection. Bio-Med Mater Eng. 21(3):139–147.
- Garcia-Gonzalez D, Jayamohan J, Sotiropoulos S. N, Yoon S. H, Cook J, Siviour C. R, Arias A, Jérusalem A. 2017. On the mechanical behaviour of PEEK and HA cranial implants under impact loading. J Mech Behav Biomed Mater. 69:342–354.